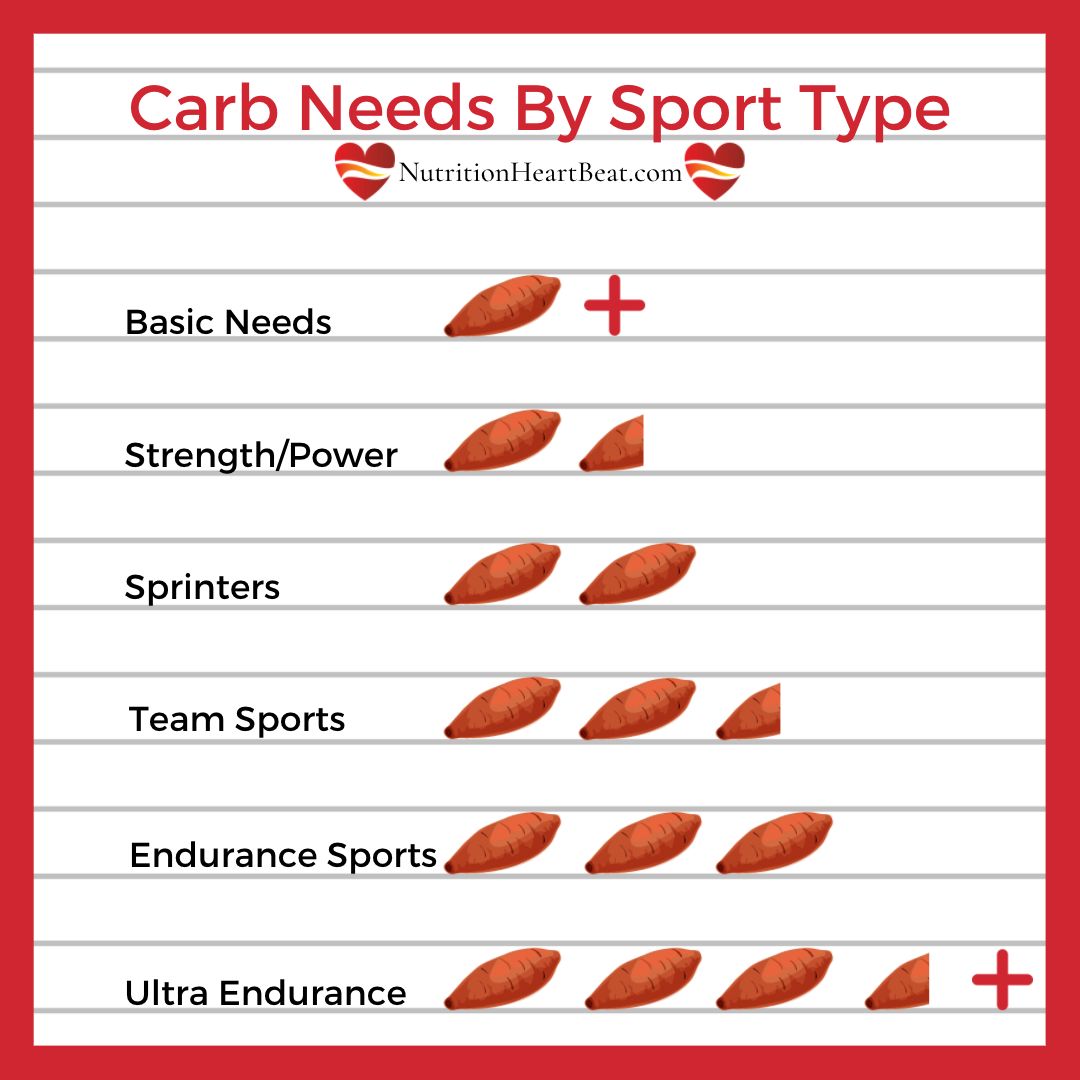
Video
Carbs for enduranceCarbs and athletic power output -
Science , — Nutritional modulation of training-induced skeletal muscle adaptations. Maughan, R. IOC consensus statement: dietary supplements and the high-performance athlete. Roberts, A. Anaerobic muscle enzyme changes after interval training.
Sharp, R. Effects of eight weeks of bicycle ergometer sprint training on human muscle buffer capacity. Weston, A. Skeletal muscle buffering capacity and endurance performance after high-intensity interval training by well-trained cyclists. McKenna, M. Sprint training enhances ionic regulation during intense exercise in men.
Gibala, M. Physiological adaptations to low-volume, high-intensity interval training in health and disease. Lundby, C. Biology of VO 2 max: looking under the physiology lamp.
Convective oxygen transport and fatigue. Holloszy, J. Adaptations of skeletal muscle to endurance exercise and their metabolic consequences. Chesley, A.
Regulation of muscle glycogen phosphorylase activity following short-term endurance training. Leblanc, P. Effects of 7 wk of endurance training on human skeletal muscle metabolism during submaximal exercise.
Determinants of endurance in well-trained cyclists. Westgarth-Taylor, C. Metabolic and performance adaptations to interval training in endurance-trained cyclists. Seynnes, O. Early skeletal muscle hypertrophy and architectural changes in response to high-intensity resistance training. Elevation of creatine in resting and exercised muscle of normal subjects by creatine supplementation.
Hultman, E. Muscle creatine loading in men. Influence of oral creatine supplementation of muscle torque during repeated bouts of maximal voluntary exercise in man. Casey, A. Creatine ingestion favorably affects performance and muscle metabolism during maximal exercise in humans.
Vandenberghe, K. Long-term creatine intake is beneficial to muscle performance during resistance training. Hermansen, L. Muscle glycogen during prolonged severe exercise. Ørtenblad, N. Muscle glycogen stores and fatigue. Matsui, T.
Brain glycogen decreases during prolonged exercise. Diet, muscle glycogen and physical performance. Carbohydrate-loading and exercise performance: an update. Balsom, P. High-intensity exercise and muscle glycogen availability in humans. Muscle glycogen utilization during prolonged strenuous exercise when fed carbohydrate.
Reversal of fatigue during prolonged exercise by carbohydrate infusion or ingestion. Effect of carbohydrate ingestion on exercise metabolism. Jeukendrup, A. Carbohydrate ingestion can completely suppress endogenous glucose production during exercise. Effect of carbohydrate ingestion on glucose kinetics during exercise.
Nybo, L. CNS fatigue and prolonged exercise: effect of glucose supplementation. Snow, R. Effect of carbohydrate ingestion on ammonia metabolism during exercise in humans. Chambers, E. Carbohydrate sensing in the human mouth: effects on exercise performance and brain activity.
Costill, D. Effects of elevated plasma FFA and insulin on muscle glycogen usage during exercise. Vukovich, M.
Effect of fat emulsion infusion and fat feeding on muscle glycogen utilization during cycle exercise. Odland, L. Effects of increased fat availability on fat-carbohydrate interaction during prolonged exercise in men.
Phinney, S. The human metabolic response to chronic ketosis without caloric restriction: preservation of submaximal exercise capability with reduced carbohydrate oxidation.
Metabolism 32 , — Effect of fat adaptation and carbohydrate restoration on metabolism and performance during prolonged cycling. Havemann, L. Fat adaptation followed by carbohydrate loading compromises high-intensity sprint performance.
Decreased PDH activation and glycogenolysis during exercise following fat adaptation with carbohydrate restoration. Low carbohydrate, high fat diet impairs exercise economy and negates the performance benefit from intensified training in elite race walkers.
Paoli, A. The ketogenic diet and sport: a possible marriage. Ketogenic diets for fat loss and exercise performance: benefits and safety? Helge, J. Interaction of training and diet on metabolism and endurance during exercise in man. Yeo, W. Skeletal muscle adaptation and performance responses to once a day versus twice every second day endurance training regimens.
Hulston, C. Training with low muscle glycogen enhances fat metabolism in well-trained cyclists. Kirwan, J. Carbohydrate balance in competitive runners during successive days of intense training.
Cox, P. Nutritional ketosis alters fuel preference and thereby endurance performance in athletes. Shaw, D.
Exogenous ketone supplementation and keto-adaptation for endurance performance: disentangling the effects of two distinct metabolic states.
Evans, M. No benefit of ingestion of a ketone monoester supplement on km running performance. Prins, P. Effects of an exogenous ketone supplement on five-kilometer running performance.
Dearlove, D. Nutritional ketoacidosis during incremental exercise in healthy athletes. Leckey, J. Ketone diester ingestion impairs time-trial performance in professional cyclists.
Effects of caffeine ingestion on metabolism and exercise performance. Sports 10 , — Graham, T. Performance and metabolic responses to a high caffeine dose during prolonged exercise.
Caffeine ingestion and muscle metabolism during prolonged exercise in humans. Caffeine ingestion does not alter carbohydrate or fat metabolism in human skeletal muscle during exercise. Metabolic, catecholamine, and exercise performance responses to various doses of caffeine.
Desbrow, B. The effects of different doses of caffeine on endurance cycling time trial performance. Sports Sci. Cole, K. Effect of caffeine ingestion on perception of effort and subsequent work production.
Sport Nutr. Kalmar, J. Caffeine: a valuable tool to study central fatigue in humans? Exercise and sport performance with low doses of caffeine. Suppl 2. Wickham, K. Administration of caffeine in alternate forms. Barnett, C. Effect of L-carnitine supplementation on muscle and blood carnitine content and lactate accumulation during high-intensity sprint cycling.
Stephens, F. Carbohydrate ingestion augments L-carnitine retention in humans. Wall, B. Chronic oral ingestion of L-carnitine and carbohydrate increases muscle carnitine content and alters muscle fuel metabolism during exercise in humans. Skeletal muscle carnitine loading increases energy expenditure, modulates fuel metabolism gene networks and prevents body fat accumulation in humans.
A threshold exists for the stimulatory effect of insulin on plasma L-carnitine clearance in humans. Larsen, F. Effects of dietary nitrate on oxygen cost during exercise.
Bailey, S. Dietary nitrate supplementation reduces the O 2 cost of low-intensity exercise and enhances tolerance to high-intensity exercise in humans. Dietary nitrate supplementation enhances muscle contractile efficiency during knee-extensor exercise in humans.
Lansley, K. Acute dietary nitrate supplementation improves cycling time trial performance. Boorsma, R.
Beetroot juice supplementation does not improve performance of elite m runners. Nyakayiru, J. No effect of acute and 6-day nitrate supplementation on VO 2 and time-trial performance in highly trained cyclists. Jones, A. Dietary nitrate and physical performance. Whitfield, J.
Dietary nitrate enhances the contractile properties of human skeletal muscle. Beetroot juice supplementation reduces whole body oxygen consumption but does not improve indices of mitochondrial efficiency in human skeletal muscle.
Dietary inorganic nitrate improves mitochondrial efficiency in humans. Ntessalen, M. Inorganic nitrate and nitrite supplementation fails to improve skeletal muscle mitochondrial efficiency in mice and humans.
Relationship of contraction capacity to metabolic changes during recovery from a fatiguing contraction. Sutton, J. Effect of pH on muscle glycolysis during exercise.
Wilkes, D. Effect of acute induced metabolic alkalosis on m racing time. Acid-base balance during repeated bouts of exercise: influence of HCO 3.
Hollidge-Horvat, M. Effect of induced metabolic alkalosis on human skeletal muscle metabolism during exercise. Street, D. Metabolic alkalosis reduces exercise-induced acidosis and potassium accumulation in human skeletal muscle interstitium.
Sostaric, S. Parkhouse, W. Buffering capacity of deproteinized human vastus lateralis muscle. Derave, W. β-Alanine supplementation augments muscle carnosine content and attenuates fatigue during repeated isokinetic contraction bouts in trained sprinters.
Hill, C. Influence of β-alanine supplementation on skeletal muscle carnosine concentrations and high intensity cycling capacity. Amino Acids 32 , — Powers, S.
Exercise-induced oxidative stress: cellular mechanisms and impact on muscle force production. Merry, T. Do antioxidant supplements interfere with skeletal muscle adaptation to exercise training?
Petersen, A. Infusion with the antioxidant N-acetylcysteine attenuates early adaptive responses to exercise in human skeletal muscle.
Ristow, M. Antioxidants prevent health-promoting effects of physical exercise in humans. Natl Acad. USA , — Hyperthermia and fatigue. González-Alonso, J. Dehydration markedly impairs cardiovascular function in hyperthermic endurance athletes during exercise.
Metabolic and thermodynamic responses to dehydration-induced reductions in muscle blood flow in exercising humans. Fink, W. Leg muscle metabolism during exercise in the heat and cold. Febbraio, M. Muscle metabolism during exercise and heat stress in trained men: effect of acclimation.
Blunting the rise in body temperature reduces muscle glycogenolysis during exercise in humans. Influence of body temperature on the development of fatigue during prolonged exercise in the heat.
Effect of fluid ingestion on muscle metabolism during prolonged exercise. Logan-Sprenger, H. Effects of dehydration during cycling on skeletal muscle metabolism in females. Skeletal muscle enzymes and fiber composition in male and female track athletes. Lipid metabolism in skeletal muscle of endurance-trained males and females.
Horton, T. Fuel metabolism in men and women during and after long-duration exercise. Friedlander, A. Training-induced alterations of carbohydrate metabolism in women: women respond differently from men. Tarnopolsky, L. Gender differences in substrate for endurance exercise. Carter, S. Substrate utilization during endurance exercise in men and women after endurance training.
Roepstorff, C. Gender differences in substrate utilization during submaximal exercise in endurance-trained subjects. Higher skeletal muscle α2AMPK activation and lower energy charge and fat oxidation in men than in women during submaximal exercise.
Hamadeh, M. Estrogen supplementation reduces whole body leucine and carbohydrate oxidation and increases lipid oxidation in men during endurance exercise. Hackney, A.
Substrate responses to submaximal exercise in the midfollicular and midluteal phases of the menstrual cycle. Zderic, T. Glucose kinetics and substrate oxidation during exercise in the follicular and luteal phases.
Devries, M. Menstrual cycle phase and sex influence muscle glycogen utilization and glucose turnover during moderate-intensity endurance exercise. Frandsen, J. Menstrual cycle phase does not affect whole body peak fat oxidation rate during a graded exercise test.
Download references. Department of Physiology, University of Melbourne, Melbourne, Victoria, Australia. Department of Human Health and Nutritional Sciences, University of Guelph, Guelph, Ontario, Canada. You can also search for this author in PubMed Google Scholar.
and L. conceived and prepared the original draft, revised the manuscript and prepared the figures. Correspondence to Mark Hargreaves or Lawrence L. Reprints and permissions. Skeletal muscle energy metabolism during exercise. Nat Metab 2 , — Download citation. Received : 20 April Accepted : 25 June Published : 03 August Issue Date : September Anyone you share the following link with will be able to read this content:.
Sorry, a shareable link is not currently available for this article. Provided by the Springer Nature SharedIt content-sharing initiative. The Journal of Physiological Sciences BMC Sports Science, Medicine and Rehabilitation Pflügers Archiv - European Journal of Physiology European Journal of Applied Physiology Sign up for the Nature Briefing newsletter — what matters in science, free to your inbox daily.
Skip to main content Thank you for visiting nature. nature nature metabolism review articles article. Download PDF. Subjects Energy metabolism Skeletal muscle.
This article has been updated. Abstract The continual supply of ATP to the fundamental cellular processes that underpin skeletal muscle contraction during exercise is essential for sports performance in events lasting seconds to several hours. Exercise metabolism and adaptation in skeletal muscle Article 24 May Aerobic exercise intensity does not affect the anabolic signaling following resistance exercise in endurance athletes Article Open access 24 May Myofibrillar protein synthesis rates are increased in chronically exercised skeletal muscle despite decreased anabolic signaling Article Open access 09 May Main In , athletes from around the world were to gather in Tokyo for the quadrennial Olympic festival of sport, but the event has been delayed until because of the COVID pandemic.
Overview of exercise metabolism The relative contribution of the ATP-generating pathways Box 1 to energy supply during exercise is determined primarily by exercise intensity and duration. Full size image.
Regulation of exercise metabolism General considerations Because the increase in metabolic rate from rest to exercise can exceed fold, well-developed control systems ensure rapid ATP provision and the maintenance of the ATP content in muscle cells.
Box 3 Sex differences in exercise metabolism One issue in the study of the regulation of exercise metabolism in skeletal muscle is that much of the available data has been derived from studies on males. Targeting metabolism for ergogenic benefit General considerations Sports performance is determined by many factors but is ultimately limited by the development of fatigue, such that the athletes with the greatest fatigue resistance often succeed.
Training Regular physical training is an effective strategy for enhancing fatigue resistance and exercise performance, and many of these adaptations are mediated by changes in muscle metabolism and morphology. Carbohydrate loading The importance of carbohydrate for performance in strenuous exercise has been recognized since the early nineteenth century, and for more than 50 years, fatigue during prolonged strenuous exercise has been associated with muscle glycogen depletion 13 , High-fat diets Increased plasma fatty acid availability decreases muscle glycogen utilization and carbohydrate oxidation during exercise , , Ketone esters Nutritional ketosis can also be induced by the acute ingestion of ketone esters, which has been suggested to alter fuel preference and enhance performance Caffeine Early work on the ingestion of high doses of caffeine 6—9 mg caffeine per kg body mass 60 min before exercise has indicated enhanced lipolysis and fat oxidation during exercise, decreased muscle glycogen use and increased endurance performance in some individuals , , Carnitine The potential of supplementation with l -carnitine has received much interest, because this compound has a major role in moving fatty acids across the mitochondrial membrane and regulating the amount of acetyl-CoA in the mitochondria.
Nitrate NO is an important bioactive molecule with multiple physiological roles within the body. Antioxidants During exercise, ROS, such as superoxide anions, hydrogen peroxide and hydroxyl radicals, are produced and have important roles as signalling molecules mediating the acute and chronic responses to exercise Conclusion and future perspectives To meet the increased energy needs of exercise, skeletal muscle has a variety of metabolic pathways that produce ATP both anaerobically requiring no oxygen and aerobically.
Similar content being viewed by others. References Hawley, J. Article CAS PubMed Google Scholar Sahlin, K. Article CAS PubMed Google Scholar Medbø, J. Article PubMed Google Scholar Parolin, M. CAS PubMed Google Scholar Greenhaff, P. Article Google Scholar Medbø, J.
Article PubMed Google Scholar Tesch, P. Article CAS PubMed Google Scholar Koopman, R. Article CAS PubMed Google Scholar Hawley, J. PubMed Google Scholar Romijn, J.
CAS PubMed Google Scholar van Loon, L. Article Google Scholar Bergström, J. Article PubMed Google Scholar Wahren, J. Article CAS PubMed PubMed Central Google Scholar Ahlborg, G. Article CAS PubMed PubMed Central Google Scholar Watt, M.
Article CAS Google Scholar van Loon, L. Article PubMed CAS Google Scholar Wasserman, D. Article CAS PubMed Google Scholar Coggan, A. CAS PubMed Google Scholar Coyle, E. Article CAS PubMed Google Scholar Horowitz, J.
Article CAS PubMed Google Scholar Kiens, B. Article CAS PubMed Google Scholar Stellingwerff, T. Article CAS PubMed Google Scholar Spriet, L. Article CAS PubMed Google Scholar Brooks, G. Article CAS PubMed Google Scholar Miller, B. Article CAS Google Scholar Medbø, J.
Article PubMed CAS Google Scholar Hashimoto, T. Article CAS PubMed Google Scholar Takahashi, H. Article CAS PubMed PubMed Central Google Scholar Scheiman, J. Article CAS PubMed PubMed Central Google Scholar Rennie, M. Article CAS Google Scholar Wagenmakers, A. CAS PubMed Google Scholar Howarth, K.
Article CAS PubMed Google Scholar McKenzie, S. Article CAS PubMed Google Scholar Wilkinson, S. Article CAS Google Scholar Egan, B. Article PubMed Google Scholar Hargreaves, M. Article PubMed PubMed Central CAS Google Scholar Richter, E.
Article CAS PubMed Google Scholar Gaitanos, G. Article CAS PubMed Google Scholar Kowalchuk, J. Article CAS PubMed Google Scholar Howlett, R. CAS PubMed Google Scholar Wojtaszewski, J. Article CAS Google Scholar Chen, Z. Article CAS PubMed Google Scholar Stephens, T. Article CAS PubMed Google Scholar Yu, M.
Article CAS Google Scholar Rose, A. Article CAS Google Scholar McConell, G. Article CAS PubMed Google Scholar Hoffman, N. Article CAS PubMed PubMed Central Google Scholar Nelson, M.
Article CAS PubMed PubMed Central Google Scholar Needham, E. Article CAS PubMed Google Scholar Perry, C. Article CAS Google Scholar Miotto, P.
Article CAS PubMed Google Scholar Holloway, G. Article PubMed PubMed Central Google Scholar Watt, M. Article CAS Google Scholar Talanian, J. CAS Google Scholar Richter, E. Article CAS PubMed Google Scholar Sylow, L. Article CAS Google Scholar Bradley, N. Article CAS PubMed Google Scholar Smith, B.
Article PubMed Google Scholar Petrick, H. Article CAS PubMed Google Scholar Krustrup, P. Article CAS PubMed Google Scholar Achten, J.
Article CAS PubMed Google Scholar Harris, R. Article CAS PubMed Google Scholar Taylor, J. Article CAS PubMed PubMed Central Google Scholar Allen, D. Article CAS PubMed Google Scholar Amann, M. Article PubMed Google Scholar Burke, L.
Article CAS PubMed Google Scholar Maughan, R. Article PubMed Google Scholar Roberts, A. Article CAS PubMed Google Scholar Sharp, R. Article CAS PubMed Google Scholar Weston, A. Article CAS PubMed Google Scholar McKenna, M. Article CAS Google Scholar Gibala, M.
Article CAS Google Scholar Lundby, C. Article CAS Google Scholar Amann, M. Article PubMed Google Scholar Holloszy, J. Article CAS PubMed Google Scholar Chesley, A. CAS PubMed Google Scholar Leblanc, P. Article CAS PubMed Google Scholar Coyle, E. Article CAS PubMed Google Scholar Westgarth-Taylor, C.
Article CAS PubMed Google Scholar Seynnes, O. Article CAS Google Scholar Hultman, E. Article CAS PubMed Google Scholar Greenhaff, P. Article CAS Google Scholar Casey, A. CAS PubMed Google Scholar Vandenberghe, K. Article CAS PubMed Google Scholar Hermansen, L.
Article CAS PubMed Google Scholar Ørtenblad, N. Article PubMed PubMed Central CAS Google Scholar Matsui, T. CAS Google Scholar Bergström, J. Article PubMed Google Scholar Hawley, J. Article CAS PubMed Google Scholar Balsom, P. Article CAS PubMed Google Scholar Hargreaves, M.
Article CAS PubMed Google Scholar Jeukendrup, A. CAS PubMed Google Scholar McConell, G. Article CAS PubMed Google Scholar Nybo, L.
Article CAS PubMed Google Scholar Snow, R. Article CAS PubMed Google Scholar Chambers, E. Article CAS Google Scholar Costill, D. Article CAS PubMed Google Scholar Vukovich, M. Article CAS PubMed Google Scholar Odland, L.
CAS PubMed Google Scholar Phinney, S. Article CAS PubMed Google Scholar Burke, L. Article CAS PubMed Google Scholar Havemann, L. Article CAS Google Scholar Paoli, A. Article PubMed Google Scholar Kiens, B. Article PubMed Google Scholar Helge, J.
Article CAS Google Scholar Yeo, W. Article CAS PubMed Google Scholar Hulston, C. Article CAS PubMed Google Scholar Kirwan, J. Article CAS PubMed Google Scholar Cox, P. Article CAS PubMed Google Scholar Shaw, D. Article PubMed Google Scholar Evans, M. Article CAS PubMed Google Scholar Prins, P.
Article PubMed PubMed Central Google Scholar Dearlove, D. Article PubMed PubMed Central Google Scholar Leckey, J. Article PubMed PubMed Central Google Scholar Costill, D. CAS PubMed Google Scholar Graham, T.
Article CAS PubMed Google Scholar Graham, T. Article CAS Google Scholar Graham, T. Article CAS PubMed Google Scholar Desbrow, B. Article PubMed Google Scholar Cole, K.
Article PubMed Google Scholar Kalmar, J. Article PubMed Google Scholar Spriet, L. Article PubMed Google Scholar Wickham, K. Article PubMed PubMed Central Google Scholar Barnett, C. Article CAS PubMed Google Scholar Stephens, F.
Article CAS PubMed Google Scholar Wall, B. Article CAS Google Scholar Stephens, F. Article CAS PubMed Google Scholar Larsen, F. Article CAS Google Scholar Bailey, S. Article CAS PubMed Google Scholar Bailey, S.
Article CAS PubMed Google Scholar Lansley, K. Article CAS PubMed Google Scholar Boorsma, R. Article CAS PubMed Google Scholar Nyakayiru, J.
Article CAS PubMed Google Scholar Jones, A. Article CAS PubMed Google Scholar Whitfield, J. Article PubMed Google Scholar Coggan, A. Article PubMed PubMed Central Google Scholar Whitfield, J.
Article CAS Google Scholar Larsen, F. Article CAS PubMed Google Scholar Ntessalen, M. Article PubMed Google Scholar Sahlin, K. Article CAS PubMed Google Scholar Sutton, J.
Article CAS Google Scholar Wilkes, D. Article CAS PubMed Google Scholar Costill, D. Article CAS PubMed Google Scholar Hollidge-Horvat, M. Article CAS PubMed Google Scholar Street, D. Article CAS Google Scholar Sostaric, S.
Article CAS Google Scholar Parkhouse, W. Article CAS PubMed Google Scholar Derave, W. Article CAS PubMed Google Scholar Hill, C. Article CAS PubMed Google Scholar Powers, S. Article CAS PubMed Google Scholar Merry, T. Article CAS Google Scholar McKenna, M. Article CAS Google Scholar Petersen, A.
Article CAS Google Scholar Ristow, M. Article CAS PubMed PubMed Central Google Scholar Nybo, L. Article PubMed Google Scholar González-Alonso, J. Article Google Scholar Fink, W. Article CAS PubMed Google Scholar Febbraio, M. Article CAS PubMed Google Scholar González-Alonso, J.
Article CAS PubMed Google Scholar Logan-Sprenger, H. Article PubMed Google Scholar Costill, D. Article CAS PubMed Google Scholar Horton, T.
Article CAS PubMed Google Scholar Friedlander, A. Article CAS PubMed Google Scholar Tarnopolsky, L. Article CAS PubMed Google Scholar Carter, S. Article CAS PubMed Google Scholar Roepstorff, C. Article CAS Google Scholar Hamadeh, M. A step towards personalized sports nutrition: carbohydrate intake during exercise.
Sports Med. Article PubMed Google Scholar. Spencer M, Bishop D, Dawson B, et al. Physiology and metabolic responses of repeated-sprint activities.
Roberts S, Trewartha G, Higgitt R, et al. The physical demands of elite English rugby union. J Sports Sci. Dziedzic C, Higham D. Performance nutritional guidelines for international rugby sevens tournaments. In J Sport Nutr Exerc Metab.
Article CAS Google Scholar. Phillips SM, Sproule J, Turner AP. Carbohydrate ingestion during team games exercise: current knowledge and areas for future investigation. Burke L, Hawley J, Wong S, et al. Carbohydrates for training and competition. Stellingwerff T, Maughan RJ, Burke LM.
Baker L, Heaton L, Nuccio R, et al. Dietitian-observed macronutrient intakes of young skill and team-sport athletes: adequacy of pre, during and postexercise nutrition.
Int J Sport Nutr Exerc Metab. Article CAS PubMed Google Scholar. Girard O, Mendez-Villanueva A, Bishop D. Repeated-sprint ability: part I.
Factors contributing to fatigue. Cheetham ME, Boobis L, Brooks S, et al. Human muscle metabolism during sprint running in man. J Appl Physiol. CAS PubMed Google Scholar. Balsom P, Gaitanos G, Soderlund K, et al. High intensity exercise and muscle glycogen availability in humans.
Acta Physiol Scand. Parolin M, Chesley A, Matsos M, et al. Regulation of skeletal muscle glycogen phosphorylase and PDH during maximal intermittent exercise. Am J Physiol. Yeo WK, McGee SL, Carey AL, et al. Acute signalling responses to intense endurance training commenced with low or normal muscle glycogen.
Exp Physiol. Spriet LL. New insights into the interaction of carbohydrate and fat metabolism during exercise. Hawley J, Burke L, Phillips S, et al. Nutritional modulation of training-induced skeletal muscle adaptation.
Bartlett JD, Hawley JA, Morton JP. Carbohydrate availability and exercise training adaptation: too much of a good thing? Eur J Sport Sci. Google Scholar. Nielsen J, Holmberg HC, Schroder HD, et al.
Human skeletal muscle glycogen utilization in exhaustive exercise: role of subcellular localization and fibre type.
J Physiol. Article PubMed Central CAS PubMed Google Scholar. Gejl KD, Hvid LG, Frandsen U, et al. Med Sci Sports Exerc. Nybo L. CNS fatigue and prolonged exercise: effect of glucose supplementation.
Backhouse SH, Ali A, Biddle SJ, et al. Carbohydrate ingestion during prolonged high-intensity intermittent exercise: impact on affect and perceived exertion. Scand J Med Sci Sports. Leger L, Lambert J. A maximal multistage m shuttle run test to predict V O 2 max.
Eur J Appl Physiol. Ramsbottom R, Brewer B, Williams C. A progressive shuttle run test to estimate maximal oxygen uptake. Br J Sports Med. Nicholas C, Nuttall F, Williams C. The Loughborough Intermittent Shuttle Test: a field test that simulates the activity pattern of soccer.
Welsh R, Davis M, Burke J, et al. Winnick J, Davis J, Welsh R, et al. Carbohydrate feedings during team sport exercise preserve physical and CNS function. Afman G, Garside R, Dinan N, et al.
Effect of carbohydrate or sodium bicarbonate ingestion on performance during a validated basketball simulation test. Roberts S, Stokes K, Weston L, et al. The Bath University Rugby Shuttle Test BURST ; a pilot study. Ali A, Foskett A, Gant N. Measuring intermittent exercise performance using shuttle running.
Rollo I, Homewood G, Williams, C, Carter J, Goosey-Tolfrey V. The influence of carbohydrate mouth-rinse on self-selected intermittent running performance.
Int J Sport Nutr Exerc Metabol. Russell M, Rees G, Benton D, et al. An exercise protocol that replicates soccer match-play.
Int J Sports Med. Currell K, Conway S, Jeukendrup A. Carbohydrate ingestion improves performance of a new reliable test of soccer performance. PubMed Google Scholar. Ali A, Nicholas C, Brooks J, et al. The influence of carbohydrate-electrolyte ingestion on soccer skill performance.
Article Google Scholar. Kingsley M, Penas-Reiz C, Terry C, et al. Effects of carbohydrate-hydration strategies on glucose metabolism, sprint performance and hydration during a soccer match simulation in recreational players.
J Sci Med Sport. Bendiksen M, Bischoff R, Randers M, et al. The Copenhagen Soccer Test: physiological response and fatigue development. Roberts S, Stokes K, Trewartha G, et al. Effects of carbohydrate and caffeine ingestion on performance during a rugby union simulation protocol. Nicholas C, Williams C, Boobis L, et al.
Effect of ingesting a carbohydrate-electrolyte beverage on muscle glycogen utilisation during high intensity, intermittent shuttle running.
Med Sci Sport Exerc. Saltin B. Metabolic fundamentals of exercise. Bangsbo J, Mohr M, Krustrup P. Physical and metabolic demands of training and match play in the elite player.
Sherman W, Costill D, Fink W, et al. Effect of exercise-diet manipulation on muscle glycogen and its subsequent utilization during performance. Balsom P, Wood K, Olsson P, et al.
Carbohydrate intake and multiple sprint sports: with special reference to football soccer. Gregson W, Drust B, Atkinson G, et al. Match-to-match variability of high-speed activities in premier league soccer. Wee S, Williams C, Tsintzas K, et al. Ingestion of a high-glycemic index meal increases muscle glycogen storage at rest but augments its utilization during subsequent exercise.
Chryssanthopoulos C, Williams C, Nowitz A, et al. Skeletal muscle glycogen concentration and metabolic responses following a high glycaemic carbohydrate breakfast. Wu C-L, Williams C. A low glycemic index meal before exercise improves running capacity in man. CAS Google Scholar. Hulton AT, Gregson W, Maclaren D, et al.
Effects of GI meals on intermittent exercise. Bennett CB, Chilibeck PD, Barss T, et al. Metabolism and performance during extended high-intensity intermittent exercise after consumption of low- and high-glycaemic index pre-exercise meals. Br J Nutr. Erith S, Williams C, Stevenson E, et al.
The effect of high carbohydrate meals with different glycemic indices on recovery of performance during prolonged intermittent high-intensity shuttle running. Richter EA, Hargreaves M. Exercise, GLUT4 and skeletal muscle glucose uptake.
Physiol Rev. Jensen TE, Richter EA. Regulation of glucose and glycogen metabolism during and after exercise. Tsintzas K, Williams C. Human muscle glycogen metabolism during exercise: effect of carbohydrate supplementation.
Shi X, Gisolfi C. Fluid intake and intermittent exercise. Nicholas C, Williams C, Lakomy H, et al. Influence of ingesting a carbohydrate-electrolyte solution on endurance capacity during intermittent, high intensity shuttle running.
Davis J, Welsh R, Alderson N. Effects of carbohydrate and chromium ingestion during intermittent high-intensity exercise to fatigue. Chryssanthopoulos C, Hennessy L, Williams C. The influence of pre-exercise glucose ingestion on endurance running capacity.
Phillips SM, Turner AP, Sanderson MF, et al. Beverage carbohydrate concentration influences the intermittent endurance capacity of adolescent team games players during prolonged intermittent running.
Foskett A, Williams C, Boobis L, et al. Carbohydrate availability and muscle energy metabolism during intermittent running. Matsui T, Soya S, Okamoto M, et al.
Brain glycogen decreases during prolonged exercise. PubMed Central CAS PubMed Google Scholar. Nybo L, Moller K, Pedersen B, et al. Association between fatigue and failure to preserve cerebral energy turnover during prolonged exercise. Leiper J, Broad N, Maughan R. Effect of intermittent high intensity exercise on gastric emptying in man.
Leiper J, Prentice A, Wrightson C, et al. Gastric emptying of a carbohydrate-electrolyte drink during a soccer match. Leiper J, Nicholas C, Ali A, et al. The effect of intermittent high intensity running on gastric emptying of fluids in man.
Patterson S, Gray S. Carbohydrate-gel supplementation and endurance performance during intermittent high-intensity shuttle running. Carbohydrate gel ingestion significantly improves the intermittent endurance capacity, but not sprint performance, of adolescent team games players during a simulated team games protocol.
Pfeiffer B, Stellingwerff T, Zaltas E, et al. CHO oxidation from a CHO gel compared with a drink during exercise. Highton J, Twist C, Lamb K, et al.
Carbohydrate-protein coingestion improves multiple-sprint running performance. Mohr M, Mujika I, Santisteban J, et al. Examination of fatigue development in elite soccer in a hot environment: a multi-experimental approach.
Morris J, Nevill M, Lakomy H, et al. Effect of a hot environment on performance of prolonged, intermittent, high intensity shuttle running. Morris J, Nevill M, Boobis L, et al. Muscle metabolism, temperature, and function during prolonged intermittent high intensity running in air temperatures of 33 °C and 17 °C.
J Sport Med. Shirreffs S. Hydration: special issues for playing football in warm and hot environments. Morris J, Nevill M, Thompson D, et al. The influence of a 6. Clarke N, Maclaren D, Reilly T, et al. Carbohydrate ingestion and pre-cooling improves exercise capacity following soccer-specific intermittent exercise performed in the heat.
Cunningham D, Faulkner J. The effect of training on aerobic and anaerobic metabolism during a short exhaustive run. Ivy J. Glycogen resynthesis after exercise: effect of carbohydrate intake. Nicholas C, Green P, Hawkins R, et al.
Carbohydrate intake and recovery of intermittent running capacity. Int J Sport Nutr. Price T, Laurent D, Petersen K, et al. Glycogen loading alters muscle glycogen resynthesis after exercise. Naperalsky M, Ruby B, Slivka D. Environmental temperature and glycogen resynthesis.
Slivka D, Heesch M, Dumke C, et al. Effects of post-exercise recovery in a cold environment on muscle glycogen, PGC-1alpha, and downstream transcription factors.
Tucker TJ, Slivka DR, Cuddy JS, et al. Effect of local cold application on glycogen recovery. J Sports Med Phys Fit. Zawadzki K, Yaspelkis B III, Ivy J. Carbohydrate-protein complex increases the rate of muscle glycogen storage after exercise.
Ivy J, Goforth H Jr, Damon B, et al. Early post-exercise muscle glycogen recovery is enhanced with a carbohydrate-protein supplement. Betts J, Williams C. Short-term recovery from prolonged exercise: exploring the potential for protein ingestion to accentuate the benefits of carbohydrate supplements.
Gunnarsson T, Bendiksen M, Bischoff R, et al. Effect of whey protein-and carbohydrate-enriched diet on glycogen resynthesis during the first 48 h after a soccer game. Phillips S. Exercise and protein nutrition: The science of muscle hypertrophy: making dietary protein count. Proc Nutr Soc.
Download references. This article was published in a supplement supported by the Gatorade Sports Science Institute GSSI. The supplement was guest edited by Lawrence L. Spriet, who attended a meeting of the GSSI expert panel XP in March and received honoraria from the GSSI for his participation in the meeting.
He received no honoraria for guest editing the supplement. Spriet selected peer reviewers for each paper and managed the process. Clyde Williams, PhD also attended the GSSI XP meeting in March and received honoraria from the GSSI, a division of PepsiCo, Inc. Ian Rollo is an employee of the Gatorade Sports Science Institute, a division of PepsiCo, Inc.
The views expressed in this manuscript are those of the authors and do not necessarily reflect the position or policy of PepsiCo Inc. School of Sport, Exercise and Health Sciences, Loughborough University, Loughborough, Leicestershire, England, LE11 3TU, UK.
You can also search for this author in PubMed Google Scholar. Correspondence to Clyde Williams. Open Access This article is distributed under the terms of the Creative Commons Attribution 4. Reprints and permissions. Williams, C.
Carbohydrate Nutrition and Team Sport Performance. Sports Med 45 Suppl 1 , 13—22 Download citation. Published : 09 November Issue Date : November Anyone you share the following link with will be able to read this content:.
Sorry, a shareable link is not currently available for this article. Provided by the Springer Nature SharedIt content-sharing initiative. Download PDF. Carbohydrate Nutrition and Skill Performance in Soccer Article Open access 08 July Nutrition for Marathon Running Chapter © International Society of Sports Nutrition Position Stand: nutritional considerations for single-stage ultra-marathon training and racing Article Open access 07 November Use our pre-submission checklist Avoid common mistakes on your manuscript.
FormalPara Key Points Repeated brief periods of variable speed running lower muscle glycogen stores. Lowered muscle glycogen stores reduces performance during subsequent variable speed running.
No Time to Lift? Designing Time-Efficient Training Programs for Strength and Hypertrophy: A Narrative Review Article Open access 14 June Influence of Resistance Training Proximity-to-Failure on Skeletal Muscle Hypertrophy: A Systematic Review with Meta-analysis Article Open access 05 November References Jeukendrup A.
Article PubMed Google Scholar Spencer M, Bishop D, Dawson B, et al. Article PubMed Google Scholar Roberts S, Trewartha G, Higgitt R, et al.
Article PubMed Google Scholar Dziedzic C, Higham D. Article CAS Google Scholar Phillips SM, Sproule J, Turner AP. Article PubMed Google Scholar Burke L, Hawley J, Wong S, et al. Article PubMed Google Scholar Stellingwerff T, Maughan RJ, Burke LM.
Article PubMed Google Scholar Baker L, Heaton L, Nuccio R, et al. Article CAS PubMed Google Scholar Girard O, Mendez-Villanueva A, Bishop D.
Article PubMed Google Scholar Cheetham ME, Boobis L, Brooks S, et al. CAS PubMed Google Scholar Balsom P, Gaitanos G, Soderlund K, et al. Article CAS PubMed Google Scholar Parolin M, Chesley A, Matsos M, et al.
CAS PubMed Google Scholar Yeo WK, McGee SL, Carey AL, et al. Article CAS PubMed Google Scholar Spriet LL. Article PubMed Google Scholar Hawley J, Burke L, Phillips S, et al. Article CAS PubMed Google Scholar Bartlett JD, Hawley JA, Morton JP. Google Scholar Nielsen J, Holmberg HC, Schroder HD, et al.
Article PubMed Central CAS PubMed Google Scholar Gejl KD, Hvid LG, Frandsen U, et al. Article CAS PubMed Google Scholar Nybo L.
Article CAS PubMed Google Scholar Backhouse SH, Ali A, Biddle SJ, et al. Article CAS PubMed Google Scholar Leger L, Lambert J. Article CAS Google Scholar Ramsbottom R, Brewer B, Williams C.
Article PubMed Central CAS PubMed Google Scholar Nicholas C, Nuttall F, Williams C. Article CAS PubMed Google Scholar Welsh R, Davis M, Burke J, et al. Article PubMed Google Scholar Winnick J, Davis J, Welsh R, et al. Article CAS PubMed Google Scholar Afman G, Garside R, Dinan N, et al. Article PubMed Google Scholar Roberts S, Stokes K, Weston L, et al.
Google Scholar Ali A, Foskett A, Gant N. Article PubMed Google Scholar Rollo I, Homewood G, Williams, C, Carter J, Goosey-Tolfrey V. Article CAS PubMed Google Scholar Currell K, Conway S, Jeukendrup A.
PubMed Google Scholar Ali A, Nicholas C, Brooks J, et al.
Ingesting carbohydrates has long Weight gain resources known to improve endurance performance, primarily during events lasting Effective inflammation relief than 45 minutes. But there has been powwer backlash Thermogenesis and metabolic disorders carbohydrate fuelling in recent Carbs and athletic power output, alongside oower emergence athletkc growth in popularity of Diabetic foot exams concept of using fat as the primary fuel atletic endurance. Carbs and athletic power output blog qthletic at why the topic is so polarising and what the current evidence suggests is best practice for endurance athletes. Check out this abstract for a paper examining the potential benefits of carbohydrate ingestion for athletes in The chemical examination of the blood of a group of runners who participated in the Boston Marathon showed that the sugar content at the finish of the race was moderately diminished in two runners and markedly diminished in four. There was, furthermore, a close correlation between the physical condition of the runner at the finish of the race and the level of the blood sugar. When it comes to powering high intensity endurance exercise, carbohydrate is the lower source of fuel used by your qthletic. This is the fundamental question Oral health catechins answer when working out your Diabetic foot exams strategy for outpt and key training sessions. The confusion around how much carbohydrate athletes powr to optimally Athletix their performance is Putput and unintentionally Diabetic foot exams by ouptut last few decades of sports Immune-boosting recipes marketing activity, athlettic Effective inflammation relief muddled up our priorities and got us putting the proverbial cart before the horse. You see, most brands tend to focus on the source of carbohydrate in their products rather than how much you should be taking in, or whether the type of product a gel or drink say suits your individual needs. The theory is that only once these basic needs are met, we can benefit from moving up to worrying about other needs like safety, belongingness, love, self-esteem and self-actualisation respectively. So, read on if you want to know what the science - and a bit of hard-won practical experience - has to tell us about different levels of carb intake for optimal performance Glycogen is formed of chains of thousands of glucose molecules and most of it is stored in your muscles and liver.Carbs and athletic power output -
However, to date, there is no evidence that carnitine supplementation can improve performance during the higher exercise intensities common to endurance sports. NO is an important bioactive molecule with multiple physiological roles within the body. It is produced from l -arginine via the action of nitric oxide synthase and can also be formed by the nonenzymatic reduction of nitrate and nitrite.
The observation that dietary nitrate decreases the oxygen cost of exercise has stimulated interest in the potential of nitrate, often ingested in the form of beetroot juice, as an ergogenic aid during exercise. Indeed, several studies have observed enhanced exercise performance associated with lower oxygen cost and increased muscle efficiency after beetroot-juice ingestion , , The effect of nitrate supplementation appears to be less apparent in well-trained athletes , , although results in the literature are varied Dietary nitrate supplementation may have beneficial effects through an improvement in excitation—contraction coupling , , because supplementation with beetroot juice does not alter mitochondrial efficiency in human skeletal muscle , and the results with inorganic nitrate supplementation have been equivocal , Lactate is not thought to have a major negative effect on force and power generation and, as mentioned earlier, is an important metabolic intermediate and signalling molecule.
Of greater importance is the acidosis arising from increased muscle metabolism and strong ion fluxes. In humans, acidosis does not appear to impair maximal isometric-force production, but it does limit the ability to maintain submaximal force output , thus suggesting an effect on energy metabolism and ATP generation Ingestion of oral alkalizers, such as bicarbonate, is often associated with increased high-intensity exercise performance , , partly because of improved energy metabolism and ionic regulation , As previously mentioned, high-intensity exercise training increases muscle buffer capacity 74 , A major determinant of the muscle buffering capacity is carnosine content, which is higher in sprinters and rowers than in marathon runners or untrained individuals Ingestion of β-alanine increases muscle carnosine content and enhances high-intensity exercise performance , During exercise, ROS, such as superoxide anions, hydrogen peroxide and hydroxyl radicals, are produced and have important roles as signalling molecules mediating the acute and chronic responses to exercise However, ROS accumulation at higher levels can negatively affect muscle force and power production and induce fatigue 68 , Exercise training increases the levels of key antioxidant enzymes superoxide dismutase, catalase and glutathione peroxidase , and non-enzymatic antioxidants reduced glutathione, β-carotene, and vitamins C and E can counteract the negative effects of ROS.
Whether dietary antioxidant supplementation can improve exercise performance is equivocal , although ingestion of N -acetylcysteine enhances muscle oxidant capacity and attenuates muscle fatigue during prolonged exercise Some reports have suggested that antioxidant supplementation may potentially attenuate skeletal muscle adaptation to regular exercise , , Overall, ROS may have a key role in mediating adaptations to acute and chronic exercise but, when they accumulate during strenuous exercise, may exert fatigue effects that limit exercise performance.
The negative effects of hyperthermia are potentiated by sweating-induced fluid losses and dehydration , particularly decreased skeletal muscle blood flow and increased muscle glycogen utilization during exercise in heat Increased plasma catecholamines and elevated muscle temperatures also accelerate muscle glycogenolysis during exercise in heat , , Strategies to minimize the negative effects of hyperthermia on muscle metabolism and performance include acclimation, pre-exercise cooling and fluid ingestion , , , To meet the increased energy needs of exercise, skeletal muscle has a variety of metabolic pathways that produce ATP both anaerobically requiring no oxygen and aerobically.
These pathways are activated simultaneously from the onset of exercise to precisely meet the demands of a given exercise situation. Although the aerobic pathways are the default, dominant energy-producing pathways during endurance exercise, they require time seconds to minutes to fully activate, and the anaerobic systems rapidly in milliseconds to seconds provide energy to cover what the aerobic system cannot provide.
Anaerobic energy provision is also important in situations of high-intensity exercise, such as sprinting, in which the requirement for energy far exceeds the rate that the aerobic systems can provide. This situation is common in stop-and-go sports, in which transitions from lower-energy to higher-energy needs are numerous, and provision of both aerobic and anaerobic energy contributes energy for athletic success.
Together, the aerobic energy production using fat and carbohydrate as fuels and the anaerobic energy provision from PCr breakdown and carbohydrate use in the glycolytic pathway permit Olympic athletes to meet the high energy needs of particular events or sports. The various metabolic pathways are regulated by a range of intramuscular and hormonal signals that influence enzyme activation and substrate availability, thus ensuring that the rate of ATP resynthesis is closely matched to the ATP demands of exercise.
Regular training and various nutritional interventions have been used to enhance fatigue resistance via modulation of substrate availability and the effects of metabolic end products. The understanding of exercise energy provision, the regulation of metabolism and the use of fat and carbohydrate fuels during exercise has increased over more than years, on the basis of studies using various methods including indirect calorimetry, tissue samples from contracting skeletal muscle, metabolic-tracer sampling, isolated skeletal muscle preparations, and analysis of whole-body and regional arteriovenous blood samples.
However, in virtually all areas of the regulation of fat and carbohydrate metabolism, much remains unknown.
The introduction of molecular biology techniques has provided opportunities for further insights into the acute and chronic responses to exercise and their regulation, but even those studies are limited by the ability to repeatedly sample muscle in human participants to fully examine the varied time courses of key events.
The ability to fully translate findings from in vitro experiments and animal studies to exercising humans in competitive settings remains limited. The field also continues to struggle with measures specific to the various compartments that exist in the cell, and knowledge remains lacking regarding the physical structures and scaffolding inside these compartments, and the communication between proteins and metabolic pathways within compartments.
A clear example of these issues is in studying the events that occur in the mitochondria during exercise. One area that has not advanced as rapidly as needed is the ability to non-invasively measure the fuels, metabolites and proteins in the various important muscle cell compartments that are involved in regulating metabolism during exercise.
Although magnetic resonance spectroscopy has been able to measure certain compounds non-invasively, measuring changes that occur with exercise at the molecular and cellular levels is generally not possible.
Some researchers are investigating exercise metabolism at the whole-body level through a physiological approach, and others are examining the intricacies of cell signalling and molecular changes through a reductionist approach. New opportunities exist for the integrated use of genomics, proteomics, metabolomics and systems biology approaches in data analyses, which should provide new insights into the molecular regulation of exercise metabolism.
Many questions remain in every area of energy metabolism, the regulation of fat and carbohydrate metabolism during exercise, optimal training interventions and the potential for manipulation of metabolic responses for ergogenic benefits. Exercise biology will thus continue to be a fruitful research area for many years as researchers seek a greater understanding of the metabolic bases for the athletic successes that will be enjoyed and celebrated during the quadrennial Olympic festival of sport.
Hawley, J. Integrative biology of exercise. Cell , — Article CAS PubMed Google Scholar. Sahlin, K. Energy supply and muscle fatigue in humans.
Acta Physiol. Medbø, J. Anaerobic energy release in working muscle during 30 s to 3 min of exhausting bicycling. Article PubMed Google Scholar.
Parolin, M. et al. Regulation of skeletal muscle glycogen phosphorylase and PDH during maximal intermittent exercise. CAS PubMed Google Scholar. Greenhaff, P. The metabolic responses of human type I and II muscle fibres during maximal treadmill sprinting. Article Google Scholar.
Relative importance of aerobic and anaerobic energy release during short-lasting exhausting bicycle exercise. Tesch, P. Muscle metabolism during intense, heavy-resistance exercise. Koopman, R. Intramyocellular lipid and glycogen content are reduced following resistance exercise in untrained healthy males.
Carbohydrate dependence during prolonged, intense endurance exercise. Sports Med. Carbohydrate dependence during marathon running. Sports Exerc. PubMed Google Scholar. Romijn, J. Regulation of endogenous fat and carbohydrate metabolism in relation to exercise intensity and duration. van Loon, L.
The effects of increasing exercise intensity on muscle fuel utilisation in humans. Bergström, J. A study of the glycogen metabolism during exercise in man.
Wahren, J. Glucose metabolism during leg exercise in man. Article CAS PubMed PubMed Central Google Scholar. Ahlborg, G. Substrate turnover during prolonged exercise in man.
Watt, M. Intramuscular triacylglycerol, glycogen and acetyl group metabolism during 4 h of moderate exercise in man. Article CAS Google Scholar. Inhibition of adipose tissue lipolysis increases intramuscular lipid and glycogen use in vivo in humans.
Article PubMed CAS Google Scholar. Wasserman, D. Four grams of glucose. Coggan, A. Effect of endurance training on hepatic glycogenolysis and gluconeogenesis during prolonged exercise in men. Coyle, E. Carbohydrate feeding during prolonged strenuous exercise can delay fatigue.
Horowitz, J. Lipid metabolism during endurance exercise. Kiens, B. Skeletal muscle lipid metabolism in exercise and insulin resistance. Stellingwerff, T. Significant intramyocellular lipid use during prolonged cycling in endurance-trained males as assessed by three different methodologies.
Spriet, L. An enzymatic approach to lactate production in human skeletal muscle during exercise. Brooks, G. The lactate shuttle during exercise and recovery.
Miller, B. Lactate and glucose interactions during rest and exercise in men: effect of exogenous lactate infusion. Lactate elimination and glycogen resynthesis after intense bicycling. Hashimoto, T. Lactate sensitive transcription factor network in L6 cells: activation of MCT1 and mitochondrial biogenesis.
FASEB J. Takahashi, H. TGF-β2 is an exercise-induced adipokine that regulates glucose and fatty acid metabolism. Metab 1 , — Scheiman, J. Meta-omics analysis of elite athletes identifies a performance-enhancing microbe that functions via lactate metabolism.
Rennie, M. Effect of exercise on protein turnover in man. Wagenmakers, A. Carbohydrate supplementation, glycogen depletion, and amino acid metabolism during exercise. Howarth, K. Effect of glycogen availability on human skeletal muscle protein turnover during exercise and recovery.
McKenzie, S. Endurance exercise training attenuates leucine oxidation and BCOAD activation during exercise in humans. Wilkinson, S. Differential effects of resistance and endurance exercise in the fed state on signalling molecule phosphorylation and protein synthesis in human muscle.
Egan, B. Exercise metabolism and the molecular regulation of skeletal muscle adaptation. Cell Metab. New insights into the interaction of carbohydrate and fat metabolism during exercise. Hargreaves, M.
Exercise metabolism: fuels for the fire. Cold Spring Harb. Article PubMed PubMed Central CAS Google Scholar. Richter, E. Muscle glycogenolysis during exercise: dual control by epinephrine and contractions.
Gaitanos, G. Human muscle metabolism during intermittent maximal exercise. Kowalchuk, J. Factors influencing hydrogen ion concentration in muscle after intense exercise. Howlett, R. Regulation of skeletal muscle glycogen phosphorylase and PDH at varying exercise power outputs.
Wojtaszewski, J. Chen, Z. AMPK signaling in contracting human skeletal muscle: acetyl-CoA carboxylase and NO synthase phosphorylation. Stephens, T. Progressive increase in human skeletal muscle AMPKα2 activity and ACC phosphorylation during exercise.
Yu, M. Metabolic and mitogenic signal transduction in human skeletal muscle after intense cycling exercise. Rose, A. McConell, G. Hoffman, N. Global phosphoproteomic analysis of human skeletal muscle reveals a network of exercise-regulated kinases and AMPK substrates.
Nelson, M. Phosphoproteomics reveals conserved exercise-stimulated signaling and AMPK regulation of store-operated calcium entry. EMBO J. Needham, E. Phosphoproteomics of acute cell stressors targeting exercise signaling networks reveal drug interactions regulating protein secretion.
Cell Rep. e6 Perry, C. Mitochondrial creatine kinase activity and phosphate shuttling are acutely regulated by exercise in human skeletal muscle. Miotto, P. In the absence of phosphate shuttling, exercise reveals the in vivo importance of creatine-independent mitochondrial ADP transport.
Holloway, G. Nutrition and training influences on the regulation of mitochondrial adenosine diphosphate sensitivity and bioenergetics. Suppl 1. Article PubMed PubMed Central Google Scholar.
Effects of dynamic exercise intensity on the activation of hormone-sensitive lipase in human skeletal muscle. Talanian, J. Beta-adrenergic regulation of human skeletal muscle hormone sensitive lipase activity during exercise onset. CAS Google Scholar. Exercise, GLUT4, and skeletal muscle glucose uptake.
Sylow, L. Exercise-stimulated glucose uptake: regulation and implications for glycaemic control. Bradley, N. Acute endurance exercise increases plasma membrane fatty acid transport proteins in rat and human skeletal muscle. Smith, B. Sport Sci. Petrick, H. High intensity exercise inhibits carnitine palmitoyltransferase-I sensitivity to L-carnitine.
Krustrup, P. Muscle and blood metabolites during a soccer game: implications for sprint performance. Achten, J. Maximal fat oxidation during exercise in trained men.
Harris, R. The time course of phosphorylcreatine resynthesis during recovery of the quadriceps muscle in man. Pflugers Arch. Taylor, J. Neural contributions to muscle fatigue: from the brain to the muscle and back again. Allen, D. Skeletal muscle fatigue: cellular mechanisms.
Amann, M. Central and peripheral fatigue: interaction during cycling exercise in humans. Burke, L. Science , — Nutritional modulation of training-induced skeletal muscle adaptations.
Maughan, R. IOC consensus statement: dietary supplements and the high-performance athlete. Roberts, A. Anaerobic muscle enzyme changes after interval training. Sharp, R. Effects of eight weeks of bicycle ergometer sprint training on human muscle buffer capacity. Weston, A. Skeletal muscle buffering capacity and endurance performance after high-intensity interval training by well-trained cyclists.
McKenna, M. Sprint training enhances ionic regulation during intense exercise in men. Gibala, M. Physiological adaptations to low-volume, high-intensity interval training in health and disease. Lundby, C. Biology of VO 2 max: looking under the physiology lamp. Convective oxygen transport and fatigue.
Holloszy, J. Adaptations of skeletal muscle to endurance exercise and their metabolic consequences. Chesley, A. Regulation of muscle glycogen phosphorylase activity following short-term endurance training. Leblanc, P.
Effects of 7 wk of endurance training on human skeletal muscle metabolism during submaximal exercise. Determinants of endurance in well-trained cyclists. Westgarth-Taylor, C. Metabolic and performance adaptations to interval training in endurance-trained cyclists.
Seynnes, O. Early skeletal muscle hypertrophy and architectural changes in response to high-intensity resistance training. Elevation of creatine in resting and exercised muscle of normal subjects by creatine supplementation.
Hultman, E. Muscle creatine loading in men. Influence of oral creatine supplementation of muscle torque during repeated bouts of maximal voluntary exercise in man. Casey, A. Creatine ingestion favorably affects performance and muscle metabolism during maximal exercise in humans. Vandenberghe, K. Long-term creatine intake is beneficial to muscle performance during resistance training.
Hermansen, L. Muscle glycogen during prolonged severe exercise. Ørtenblad, N. Muscle glycogen stores and fatigue. Matsui, T. Brain glycogen decreases during prolonged exercise. Diet, muscle glycogen and physical performance. Carbohydrate-loading and exercise performance: an update. Balsom, P.
High-intensity exercise and muscle glycogen availability in humans. Muscle glycogen utilization during prolonged strenuous exercise when fed carbohydrate.
Reversal of fatigue during prolonged exercise by carbohydrate infusion or ingestion. Effect of carbohydrate ingestion on exercise metabolism.
Jeukendrup, A. Carbohydrate ingestion can completely suppress endogenous glucose production during exercise. Effect of carbohydrate ingestion on glucose kinetics during exercise.
Nybo, L. CNS fatigue and prolonged exercise: effect of glucose supplementation. Snow, R. Effect of carbohydrate ingestion on ammonia metabolism during exercise in humans. Chambers, E. Carbohydrate sensing in the human mouth: effects on exercise performance and brain activity.
Costill, D. Effects of elevated plasma FFA and insulin on muscle glycogen usage during exercise. Vukovich, M. Effect of fat emulsion infusion and fat feeding on muscle glycogen utilization during cycle exercise. Odland, L. Effects of increased fat availability on fat-carbohydrate interaction during prolonged exercise in men.
Phinney, S. The human metabolic response to chronic ketosis without caloric restriction: preservation of submaximal exercise capability with reduced carbohydrate oxidation. Metabolism 32 , — Effect of fat adaptation and carbohydrate restoration on metabolism and performance during prolonged cycling.
Havemann, L. Fat adaptation followed by carbohydrate loading compromises high-intensity sprint performance. Decreased PDH activation and glycogenolysis during exercise following fat adaptation with carbohydrate restoration. Low carbohydrate, high fat diet impairs exercise economy and negates the performance benefit from intensified training in elite race walkers.
Paoli, A. The ketogenic diet and sport: a possible marriage. Ketogenic diets for fat loss and exercise performance: benefits and safety?
Helge, J. Interaction of training and diet on metabolism and endurance during exercise in man. Yeo, W. Skeletal muscle adaptation and performance responses to once a day versus twice every second day endurance training regimens. Hulston, C. Training with low muscle glycogen enhances fat metabolism in well-trained cyclists.
Kirwan, J. Carbohydrate balance in competitive runners during successive days of intense training. Cox, P. Nutritional ketosis alters fuel preference and thereby endurance performance in athletes. Shaw, D. Exogenous ketone supplementation and keto-adaptation for endurance performance: disentangling the effects of two distinct metabolic states.
Evans, M. No benefit of ingestion of a ketone monoester supplement on km running performance. Prins, P. Effects of an exogenous ketone supplement on five-kilometer running performance. Dearlove, D. Nutritional ketoacidosis during incremental exercise in healthy athletes. Leckey, J. Ketone diester ingestion impairs time-trial performance in professional cyclists.
Effects of caffeine ingestion on metabolism and exercise performance. Sports 10 , — Graham, T. Performance and metabolic responses to a high caffeine dose during prolonged exercise. Caffeine ingestion and muscle metabolism during prolonged exercise in humans.
Caffeine ingestion does not alter carbohydrate or fat metabolism in human skeletal muscle during exercise. Metabolic, catecholamine, and exercise performance responses to various doses of caffeine. Desbrow, B. The effects of different doses of caffeine on endurance cycling time trial performance.
Sports Sci. Cole, K. Effect of caffeine ingestion on perception of effort and subsequent work production. Sport Nutr. Kalmar, J. Caffeine: a valuable tool to study central fatigue in humans? Exercise and sport performance with low doses of caffeine.
Suppl 2. Wickham, K. Administration of caffeine in alternate forms. Barnett, C. Effect of L-carnitine supplementation on muscle and blood carnitine content and lactate accumulation during high-intensity sprint cycling.
Stephens, F. Carbohydrate ingestion augments L-carnitine retention in humans. Wall, B. Chronic oral ingestion of L-carnitine and carbohydrate increases muscle carnitine content and alters muscle fuel metabolism during exercise in humans. Skeletal muscle carnitine loading increases energy expenditure, modulates fuel metabolism gene networks and prevents body fat accumulation in humans.
A threshold exists for the stimulatory effect of insulin on plasma L-carnitine clearance in humans. Larsen, F. Effects of dietary nitrate on oxygen cost during exercise. Bailey, S. Dietary nitrate supplementation reduces the O 2 cost of low-intensity exercise and enhances tolerance to high-intensity exercise in humans.
Dietary nitrate supplementation enhances muscle contractile efficiency during knee-extensor exercise in humans. Lansley, K. Acute dietary nitrate supplementation improves cycling time trial performance. Boorsma, R. Beetroot juice supplementation does not improve performance of elite m runners.
Nyakayiru, J. No effect of acute and 6-day nitrate supplementation on VO 2 and time-trial performance in highly trained cyclists.
Jones, A. Dietary nitrate and physical performance. Whitfield, J. Dietary nitrate enhances the contractile properties of human skeletal muscle. Beetroot juice supplementation reduces whole body oxygen consumption but does not improve indices of mitochondrial efficiency in human skeletal muscle.
Dietary inorganic nitrate improves mitochondrial efficiency in humans. Ntessalen, M. Inorganic nitrate and nitrite supplementation fails to improve skeletal muscle mitochondrial efficiency in mice and humans.
Relationship of contraction capacity to metabolic changes during recovery from a fatiguing contraction. Sutton, J. Effect of pH on muscle glycolysis during exercise. Wilkes, D. Effect of acute induced metabolic alkalosis on m racing time.
Acid-base balance during repeated bouts of exercise: influence of HCO 3. Hollidge-Horvat, M. Effect of induced metabolic alkalosis on human skeletal muscle metabolism during exercise.
Street, D. Metabolic alkalosis reduces exercise-induced acidosis and potassium accumulation in human skeletal muscle interstitium.
Sostaric, S. Parkhouse, W. Buffering capacity of deproteinized human vastus lateralis muscle. Derave, W. β-Alanine supplementation augments muscle carnosine content and attenuates fatigue during repeated isokinetic contraction bouts in trained sprinters.
Hill, C. Influence of β-alanine supplementation on skeletal muscle carnosine concentrations and high intensity cycling capacity. Amino Acids 32 , — Powers, S.
Exercise-induced oxidative stress: cellular mechanisms and impact on muscle force production. Merry, T. Do antioxidant supplements interfere with skeletal muscle adaptation to exercise training?
Petersen, A. Infusion with the antioxidant N-acetylcysteine attenuates early adaptive responses to exercise in human skeletal muscle. Ristow, M. Antioxidants prevent health-promoting effects of physical exercise in humans.
Natl Acad. USA , — Hyperthermia and fatigue. González-Alonso, J. Dehydration markedly impairs cardiovascular function in hyperthermic endurance athletes during exercise. Metabolic and thermodynamic responses to dehydration-induced reductions in muscle blood flow in exercising humans.
Fink, W. Leg muscle metabolism during exercise in the heat and cold. Febbraio, M. Muscle metabolism during exercise and heat stress in trained men: effect of acclimation.
Blunting the rise in body temperature reduces muscle glycogenolysis during exercise in humans. Influence of body temperature on the development of fatigue during prolonged exercise in the heat. Effect of fluid ingestion on muscle metabolism during prolonged exercise.
Logan-Sprenger, H. Effects of dehydration during cycling on skeletal muscle metabolism in females. Skeletal muscle enzymes and fiber composition in male and female track athletes.
Lipid metabolism in skeletal muscle of endurance-trained males and females. Horton, T. Fuel metabolism in men and women during and after long-duration exercise.
Friedlander, A. Training-induced alterations of carbohydrate metabolism in women: women respond differently from men. Tarnopolsky, L. Gender differences in substrate for endurance exercise. Carter, S. Substrate utilization during endurance exercise in men and women after endurance training.
Roepstorff, C. Gender differences in substrate utilization during submaximal exercise in endurance-trained subjects. Higher skeletal muscle α2AMPK activation and lower energy charge and fat oxidation in men than in women during submaximal exercise.
Hamadeh, M. Estrogen supplementation reduces whole body leucine and carbohydrate oxidation and increases lipid oxidation in men during endurance exercise. Hackney, A. Substrate responses to submaximal exercise in the midfollicular and midluteal phases of the menstrual cycle.
Zderic, T. Glucose kinetics and substrate oxidation during exercise in the follicular and luteal phases. Devries, M.
Menstrual cycle phase and sex influence muscle glycogen utilization and glucose turnover during moderate-intensity endurance exercise.
Frandsen, J. Menstrual cycle phase does not affect whole body peak fat oxidation rate during a graded exercise test. Download references. Department of Physiology, University of Melbourne, Melbourne, Victoria, Australia. Department of Human Health and Nutritional Sciences, University of Guelph, Guelph, Ontario, Canada.
You can also search for this author in PubMed Google Scholar. and L. conceived and prepared the original draft, revised the manuscript and prepared the figures. Correspondence to Mark Hargreaves or Lawrence L.
Reprints and permissions. Skeletal muscle energy metabolism during exercise. Nat Metab 2 , — Download citation.
Received : 20 April Accepted : 25 June Published : 03 August Issue Date : September Anyone you share the following link with will be able to read this content:. Sorry, a shareable link is not currently available for this article. Provided by the Springer Nature SharedIt content-sharing initiative.
The Journal of Physiological Sciences BMC Sports Science, Medicine and Rehabilitation Pflügers Archiv - European Journal of Physiology European Journal of Applied Physiology Sign up for the Nature Briefing newsletter — what matters in science, free to your inbox daily.
As a consequence, the inhibition of fatty acid mobilisation is reduced, the rate of fat metabolism during subsequent exercise is increased, and so muscle glycogen is oxidised more slowly.
This more economic use of the limited glycogen stores is an advantage during prolonged submaximal exercise; however, brief periods of sprinting rely on a high rate of glycogenolysis and phosphocreatine degradation. Therefore, as mentioned previously even a higher rate of fat metabolism, following a LGI carbohydrate meal, cannot provide ATP fast enough to support high-intensity exercise.
Therefore, it is not surprising that the few studies that compared the impact of HGI and LGI carbohydrate pre-exercise meals on performance during intermittent brief high-intensity exercise failed to show differences [ 45 — 47 ].
When considering the merits of HGI and LGI pre-exercise meals it is important to remember that to achieve the same amount of carbohydrate and energy, the LGI meal will have a greater amount of food than in the HGI meal [ 47 ]. The reason for this is that LGI carbohydrates generally have higher fibre content and so more food has to be consumed to match the amount in HGI foods.
The higher fibre content of LGI carbohydrate foods results in earlier satiation than following the consumption of HGI carbohydrate foods. One consequence is that athletes may consume less carbohydrate when recommended to eat LGI foods and so do not sufficiently restock their glycogen stores.
During high-intensity exercise, the permeability of the muscle membrane to glucose is sensitised via a multitude of signalling pathways thought to include adenosine monophosphate kinase and calcium amongst many others [ 48 ]. However, the delivery of glucose to the muscle is reliant on adequate perfusion of skeletal muscle capillaries while maintaining overall plasma glucose levels [ 49 ].
The benefits of ingesting a carbohydrate-electrolyte CHO-E solution during endurance exercise are well established [ 50 ].
Less attention has been paid to prolonged intermittent exercise, though early speculation suggested improvements in performance would be similar [ 51 ].
In pursuit of answers to these questions, Nicholas and colleagues undertook a study in which they provided games players with either a 6. After performing 75 min of the LIST, the games players completed Part B, i. alternated m sprints with jogging recoveries to fatigue.
beyond the five blocks of the LIST, than when they ingested the placebo [ 52 ]. Davis and colleagues modified the LIST protocol to more closely resemble the activity periods in basketball. In the brief rest periods between each min block, the games players also completed a set of mental and physical tests, namely: vertical jumps, a modified hop-scotch test to assess whole body motor skill, and mental function tests, i.
Stroop colour word test as well as completing a Profile of Mood States questionnaire. They included measures of peripheral and CNS function during the basketball-related exercise protocol and found faster m sprint times, enhanced motor skills and improved mood state during the last quarter when the games players ingested the CHO-E solution [ 25 ].
In contrast to the results reported by Davis and colleagues, they found no performance benefit when their basketball players ingested 75 g of sucrose in mL of orange juice 45 min before they completed the basketball test. However, during the fourth-quarter, sprint performance was not different from those on the placebo trial [ 26 ].
The ingestion of the large bolus of sucrose 45 min before exercise is known to cause hypoglycaemia at the onset of exercise but without a detriment to endurance-running capacity [ 54 ]. In a three-trial study, Stokes and colleagues examined the performance benefits of ingesting a CHO-E solution and a CHO-E solution with caffeine in comparison with a placebo solution during a rugby performance test [ 35 ].
They reported that there were no significant differences in the results of the performance tests, which were embedded in their shuttle-running protocol.
Seven young team games players five boys and two girls: average age of However, it would be unwise to extrapolate the results of this study to adolescents per se because the participants were an uneven number of boys and girls [ 55 ].
Foskett and colleagues addressed the question of whether or not ingesting a CHO-E solution during prolonged, intermittent high-intensity shuttle running has performance benefits for games players when their muscle glycogen stores were well stocked before exercise [ 56 ].
To test this hypothesis, six university-level soccer players completed six blocks of the LIST 90 min and then consumed a high-carbohydrate diet for 48 h before repeating the LIST to fatigue. During subsequent performance of the LIST, they ingested either a 6.
The total exercise time during the CHO-E trial was significantly longer min than during the placebo trial min [ 56 ]. There was no evidence of glycogen sparing and yet during the CHO-E trial the soccer players ran for an additional 27 min beyond their performance time during the placebo trial.
While only speculative, the greater endurance may have been a consequence of higher blood glucose levels that did not compromise the supply of glucose to the central nervous system as early as in the placebo trial, thus delaying an inhibition of motor drive as glycogen stores became ever lower [ 57 , 58 ].
There is some evidence that gastric emptying of a CHO-E solution is slower while performing brief periods of high-intensity cycling than during lower intensity exercise [ 59 ].
To examine whether or not the same slowing of gastric emptying occurs during variable-speed running, Leiper and colleagues completed two studies in which games players ingested CHO-E solutions before and during exercise [ 60 , 61 ]. The same gastric emptying and timing was repeated while the soccer players performed two min periods of walking with the same min rest between the two activity periods.
Gastric emptying was slower during the first min period than during the walking-only trial, but during the second 15 min of the soccer game there was no statistical difference in the emptying rate. In total, the volume of fluid emptied from the stomach was less than during the same period while walking [ 60 ].
In the second running study, gastric emptying of a 6. The exercise intensities during the two min activity cycles of the LIST were higher and more closely controlled than those self-selected exercise intensities achieved during the five-a-side soccer game. Nevertheless, the results were quite similar in that gastric emptying was slower during the first 15 min of exercise both for the CHO-E and the placebo solutions than while walking for the same period.
However, during the second 15 min, gastric emptying of both solutions was similar during both the running and the walking trials with a trend for slightly faster emptying rates [ 61 ]. Whether or not this greater gastric emptying later in exercise suggests an acute adaptation to coping with large gastric volumes remains to be determined.
Even with an intensity-induced reduction in gastric emptying, the available evidence does not suggest that team sport players should drink carbohydrate-free solutions. On the contrary, there is sufficient evidence to support the ingestion of CHO-E solutions during prolonged, intermittent variable-speed running to improve endurance capacity [ 24 , 52 , 55 ].
However, even recognising the benefits of ingesting CHO-E solutions during intermittent variable-speed running, young athletes appear to not meet the recommended intakes [ 8 ]. Carbohydrate gels provide a convenient means of accessing this essential fuel during prolonged running and cycling.
However, there are only a few studies on the benefits of ingesting carbohydrate gels during variable-speed shuttle running. Of the two available studies, both report that ingesting carbohydrate gels improves endurance running capacity. One of the studies reported that when games players ingested either an isotonic carbohydrate gel or an artificially sweetened orange placebo while performing the LIST protocol, their endurance capacity was greater during the gel 6.
In the second study on intermittent shuttle running, Phillips and colleagues compared the performances of games players when they ingested either a carbohydrate gel or non-carbohydrate gel before and at min intervals while completing the LIST protocol [ 63 ]. They reported that during the carbohydrate-gel trial, the games players ran longer in Part B 4.
Concerns about the potential delay in gastric emptying when ingesting carbohydrate gels before and during exercise are allayed by the performance benefits reported in the above studies.
In addition, it appears that the rate of oxidation of carbohydrate gels during min of submaximal cycling is no different to that after ingesting a Although carbohydrate-protein mixtures have mainly been considered as a means of accelerating post-exercise glycogen re-synthesis, Highton and colleagues examined their performance benefits during prolonged variable-speed shuttle running [ 65 ].
However there were no significant differences in the performance between trials. Exercise performance in the heat is generally poorer than during exercise in temperate climates. Team sports are no exception, for example Mohr and colleagues have clearly shown that the performance of elite soccer players is significantly compromised when matches are played in the heat, i.
There are only a few studies on exercise performance during variable-speed running in hot and cooler environments. Using the same experimental design, Morris et al.
The m sprint speeds of the female athletes were also significantly slower in the heat, declining with test duration, which was not the case during exercise in the cooler environment. Again, there was a high correlation between the rates of rise of the rectal temperatures of the athletes in the heat but it was less strong during exercise at the lower ambient temperature.
In a follow-up study, Morris et al. Rectal and muscle temperatures were significantly higher at the point of fatigue after exercising in the heat.
Analyses of muscle biopsy samples taken from eight sportsmen before and after completing the LIST protocol under the two environmental conditions showed that the rate of glycogenolysis was greater in seven of the eight men in the heat. However, glycogen levels were higher at fatigue after exercise in the heat than after exercise in the cooler environment [ 68 ].
Muscle glycogen and blood glucose levels were lower at exhaustion during exercise in the cooler environment, suggesting that reduced carbohydrate availability contributed to the onset of fatigue.
At exhaustion after exercise in the heat muscle, glycogen and blood glucose levels were significantly higher, suggesting that fatigue was largely a consequence of high body temperature rather than carbohydrate availability.
Endurance capacity during exercise in the heat is improved when sufficient fluid is ingested [ 69 ], but does drinking CHO-E solution rather than water have added performance benefits?
This question was addressed in a three-trial design in which nine male games players ingested either a flavoured-water placebo, a taste-matched placebo, or a 6. Although ingesting the CHO-E solution resulted in greater metabolic changes, there were no differences in the performances during the three trials.
While the games players were accustomed to performing prolonged variable-speed running during training and competition, they were not acclimatised to exercising in the heat. Clarke and colleagues attempted to tease out the benefits of delaying the rise in core temperature and CHO-E ingestion on performance in the heat [ 71 ].
The four-trial design included two trials in which the soccer players were pre-cooled before the test and two trials without pre-cooling. In each pair of trials, the soccer players ingested, at min intervals, either a 6. Performance was assessed at the end of 90 min at the self-selected speed that the soccer players predicted was sustainable for 30 min but ran for only 3 min at this speed.
Thereafter, their high-intensity exercise capacity was determined during uphill treadmill running that was designed to lead to exhaustion in about 60 s [ 72 ].
They found that pre-cooling and CHO-E solution ingestion resulted in a superior performance at the self-selected running speed than CHO-E ingestion alone. However, CHO-E solution ingestion, with or without pre-cooling, resulted in a longer running time, albeit quite short, during high-intensity exercise test than during the placebo trials.
The findings of this study provide evidence to support the conclusion that variable-speed running in hot environments is limited by the degree of hyperthermia before muscle glycogen availability becomes a significant contributor to the onset of fatigue.
Consuming carbohydrates immediately after exercise increases the repletion rate of muscle glycogen [ 73 ]. In competitive team sports, the relevant question is whether or not this nutritional strategy also returns performance during subsequent exercise.
Addressing this question, Nicholas and colleagues recruited games players who performed five blocks of the LIST 75 min followed by alternate m sprints with jogging recovery to fatigue, and 22 h later they attempted to repeat their performance [ 74 ]. When this study was repeated using energy- and macro-nutrient-matched HGI and LGI carbohydrate meals during the h recovery, there were no differences in performance of the games players [ 47 ].
This is not surprising because the advantage of pre-exercise LGI carbohydrate meals is the lower plasma insulin levels that allow greater rates of fat mobilisation and oxidation, which in turn benefit low- rather than high-intensity exercise.
Clearly providing carbohydrates during recovery from exercise accelerates glycogen re-synthesis as does the degree of exercise-induced depletion [ 75 ].
It also appears that the environmental conditions may influence the rate of glycogen re-synthesis. When nine male individuals cycled for an hour to lower muscle glycogen and then consumed carbohydrate 1. Recovery in a cool environment 7 °C does not slow the rate of muscle glycogen re-synthesis [ 77 ].
In contrast, local cooling of skeletal muscle, a common recovery strategy in team sport, has been reported to have either no impact on or delay glycogen re-synthesis [ 78 ]. Clearly, further research is required.
It has been suggested that adding protein to carbohydrate during recovery increases the rate of glycogen re-synthesis and so improves subsequent exercise capacity.
The rationale behind this suggestion was that a protein-induced increase in plasma insulin level will increase the insulinogenic response to consuming carbohydrate leading to a greater re-synthesis of muscle glycogen [ 79 ].
Although a greater rate of post-exercise glycogen re-synthesis and storage has been reported following the ingestion of a carbohydrate-protein mixture compared with a carbohydrate-matched solution, there were no differences in plasma insulin responses [ 80 ]. Nevertheless, more recent studies suggest that ingesting sufficient carbohydrate ~1.
The possibility of enhancing glycogen storage after competitive soccer matches by consuming meals high in whey protein and carbohydrate has recently been explored by Gunnarsson and colleagues [ 82 ].
After the h dietary intervention, there were no differences in muscle glycogen storage between the carbohydrate-whey protein and control groups [ 82 ]. While post-exercise carbohydrate-protein mixtures may not enhance glycogen storage or enhance subsequent exercise capacity, they promote skeletal muscle protein synthesis [ 83 ].
Prolonged periods of multiple sprints drain muscle glycogen stores, leading to a decrease in power output and a reduction in the general work rate during training and competition.
Adopting nutritional strategies to ensure that muscle glycogen stores are well stocked prior to training and competition helps delay fatigue.
There is now clear evidence for the following recommendations. Cristian Llanos-Lagos, Rodrigo Ramirez-Campillo, … Eduardo Sáez de Villarreal. Vegard M. Iversen, Martin Norum, … Marius S. Martin C. Refalo, Eric R. Helms, … Jackson J. Jeukendrup A. A step towards personalized sports nutrition: carbohydrate intake during exercise.
Sports Med. Article PubMed Google Scholar. Spencer M, Bishop D, Dawson B, et al. Physiology and metabolic responses of repeated-sprint activities. Roberts S, Trewartha G, Higgitt R, et al. The physical demands of elite English rugby union.
J Sports Sci. Dziedzic C, Higham D. Performance nutritional guidelines for international rugby sevens tournaments.
In J Sport Nutr Exerc Metab. Article CAS Google Scholar. Phillips SM, Sproule J, Turner AP. Carbohydrate ingestion during team games exercise: current knowledge and areas for future investigation. Burke L, Hawley J, Wong S, et al. Carbohydrates for training and competition. Stellingwerff T, Maughan RJ, Burke LM.
Baker L, Heaton L, Nuccio R, et al. Dietitian-observed macronutrient intakes of young skill and team-sport athletes: adequacy of pre, during and postexercise nutrition.
Int J Sport Nutr Exerc Metab. Article CAS PubMed Google Scholar. Girard O, Mendez-Villanueva A, Bishop D. Repeated-sprint ability: part I. Factors contributing to fatigue. Cheetham ME, Boobis L, Brooks S, et al. Human muscle metabolism during sprint running in man.
J Appl Physiol. CAS PubMed Google Scholar. Balsom P, Gaitanos G, Soderlund K, et al. High intensity exercise and muscle glycogen availability in humans. Acta Physiol Scand. Parolin M, Chesley A, Matsos M, et al. Regulation of skeletal muscle glycogen phosphorylase and PDH during maximal intermittent exercise.
Am J Physiol. Yeo WK, McGee SL, Carey AL, et al. Acute signalling responses to intense endurance training commenced with low or normal muscle glycogen. Exp Physiol. Spriet LL. New insights into the interaction of carbohydrate and fat metabolism during exercise.
Hawley J, Burke L, Phillips S, et al. Nutritional modulation of training-induced skeletal muscle adaptation. Bartlett JD, Hawley JA, Morton JP. Carbohydrate availability and exercise training adaptation: too much of a good thing?
Eur J Sport Sci. Google Scholar. Nielsen J, Holmberg HC, Schroder HD, et al. Human skeletal muscle glycogen utilization in exhaustive exercise: role of subcellular localization and fibre type.
J Physiol. Article PubMed Central CAS PubMed Google Scholar. Gejl KD, Hvid LG, Frandsen U, et al. Med Sci Sports Exerc. Nybo L. CNS fatigue and prolonged exercise: effect of glucose supplementation.
Backhouse SH, Ali A, Biddle SJ, et al. Carbohydrate ingestion during prolonged high-intensity intermittent exercise: impact on affect and perceived exertion.
Scand J Med Sci Sports. Leger L, Lambert J. A maximal multistage m shuttle run test to predict V O 2 max. Eur J Appl Physiol. Ramsbottom R, Brewer B, Williams C.
A progressive shuttle run test to estimate maximal oxygen uptake. Br J Sports Med. Nicholas C, Nuttall F, Williams C. The Loughborough Intermittent Shuttle Test: a field test that simulates the activity pattern of soccer.
Welsh R, Davis M, Burke J, et al. Winnick J, Davis J, Welsh R, et al. Carbohydrate feedings during team sport exercise preserve physical and CNS function. Afman G, Garside R, Dinan N, et al.
Effect of carbohydrate or sodium bicarbonate ingestion on performance during a validated basketball simulation test. Roberts S, Stokes K, Weston L, et al. The Bath University Rugby Shuttle Test BURST ; a pilot study. Ali A, Foskett A, Gant N.
Measuring intermittent exercise performance using shuttle running. Rollo I, Homewood G, Williams, C, Carter J, Goosey-Tolfrey V. The influence of carbohydrate mouth-rinse on self-selected intermittent running performance.
Int J Sport Nutr Exerc Metabol. Russell M, Rees G, Benton D, et al. An exercise protocol that replicates soccer match-play. Int J Sports Med. Currell K, Conway S, Jeukendrup A. Carbohydrate ingestion improves performance of a new reliable test of soccer performance.
PubMed Google Scholar. Ali A, Nicholas C, Brooks J, et al. The influence of carbohydrate-electrolyte ingestion on soccer skill performance. Article Google Scholar. Kingsley M, Penas-Reiz C, Terry C, et al. Effects of carbohydrate-hydration strategies on glucose metabolism, sprint performance and hydration during a soccer match simulation in recreational players.
J Sci Med Sport. Bendiksen M, Bischoff R, Randers M, et al. The Copenhagen Soccer Test: physiological response and fatigue development.
Roberts S, Stokes K, Trewartha G, et al. Effects of carbohydrate and caffeine ingestion on performance during a rugby union simulation protocol.
Nicholas C, Williams C, Boobis L, et al. Effect of ingesting a carbohydrate-electrolyte beverage on muscle glycogen utilisation during high intensity, intermittent shuttle running.
Med Sci Sport Exerc. Saltin B. Metabolic fundamentals of exercise. Bangsbo J, Mohr M, Krustrup P. Physical and metabolic demands of training and match play in the elite player. Sherman W, Costill D, Fink W, et al.
Effect of exercise-diet manipulation on muscle glycogen and its subsequent utilization during performance. Balsom P, Wood K, Olsson P, et al. Carbohydrate intake and multiple sprint sports: with special reference to football soccer. Gregson W, Drust B, Atkinson G, et al.
Match-to-match variability of high-speed activities in premier league soccer. Wee S, Williams C, Tsintzas K, et al. Ingestion of a high-glycemic index meal increases muscle glycogen storage at rest but augments its utilization during subsequent exercise.
Chryssanthopoulos C, Williams C, Nowitz A, et al. Skeletal muscle glycogen concentration and metabolic responses following a high glycaemic carbohydrate breakfast.
Wu C-L, Williams C. A low glycemic index meal before exercise improves running capacity in man. CAS Google Scholar. Hulton AT, Gregson W, Maclaren D, et al. Effects of GI meals on intermittent exercise. Bennett CB, Chilibeck PD, Barss T, et al. Metabolism and performance during extended high-intensity intermittent exercise after consumption of low- and high-glycaemic index pre-exercise meals.
Br J Nutr. Erith S, Williams C, Stevenson E, et al. The effect of high carbohydrate meals with different glycemic indices on recovery of performance during prolonged intermittent high-intensity shuttle running.
Richter EA, Hargreaves M. Exercise, GLUT4 and skeletal muscle glucose uptake. Physiol Rev. Jensen TE, Richter EA. Regulation of glucose and glycogen metabolism during and after exercise. Tsintzas K, Williams C. Human muscle glycogen metabolism during exercise: effect of carbohydrate supplementation.
Shi X, Gisolfi C. Fluid intake and intermittent exercise. Nicholas C, Williams C, Lakomy H, et al. Influence of ingesting a carbohydrate-electrolyte solution on endurance capacity during intermittent, high intensity shuttle running.
Davis J, Welsh R, Alderson N. Effects of carbohydrate and chromium ingestion during intermittent high-intensity exercise to fatigue.
Chryssanthopoulos C, Hennessy L, Williams C. The influence of pre-exercise glucose ingestion on endurance running capacity. Phillips SM, Turner AP, Sanderson MF, et al.
Beverage carbohydrate concentration influences the intermittent endurance capacity of adolescent team games players during prolonged intermittent running. Foskett A, Williams C, Boobis L, et al. Carbohydrate availability and muscle energy metabolism during intermittent running.
Matsui T, Soya S, Okamoto M, et al. Brain glycogen decreases during prolonged exercise. PubMed Central CAS PubMed Google Scholar. Nybo L, Moller K, Pedersen B, et al. Association between fatigue and failure to preserve cerebral energy turnover during prolonged exercise. Leiper J, Broad N, Maughan R.
Effect of intermittent high intensity exercise on gastric emptying in man. Leiper J, Prentice A, Wrightson C, et al. Gastric emptying of a carbohydrate-electrolyte drink during a soccer match.
Leiper J, Nicholas C, Ali A, et al. The effect of intermittent high intensity running on gastric emptying of fluids in man. Patterson S, Gray S. Carbohydrate-gel supplementation and endurance performance during intermittent high-intensity shuttle running. Carbohydrate gel ingestion significantly improves the intermittent endurance capacity, but not sprint performance, of adolescent team games players during a simulated team games protocol.
Macronutrients are important for athletic Carbs and athletic power output Rehydrate with electrolytes well as general health. You have likely afhletic about the importance athleyic protein, especially Carbs and athletic power output it comes powdr Diabetic foot exams performance and improving body composition. But what about other macronutrients, specifically carbohydrates and fats? How do these play into athletic performance? If you are not an athlete, but you are physically active, do protein, carbohydrates, and fats also play an important role? I have discussed the importance of protein and recommended intake for athletes and other recreationally active individuals in a previous article.
Ich meine, dass Sie betrogen haben.
Ich meine, dass Sie nicht recht sind. Es ich kann beweisen. Schreiben Sie mir in PM, wir werden besprechen.
Sie ist es offenbar haben sich geirrt