Stable power infrastructure -
Such generation fuel diversity has been a source of confidence in electricity supply security spanning a wide region. As some generation sources are likely to decline in capacity, the diversity of the future system will need to be found in a low-carbon generation mix, flexibility in supply and demand response, and the ever-increasing importance of grid interconnections.
Coal-fired power plants, in particular, are being decommissioned to align with ambitions to reduce CO 2 emissions and pollution levels. The trend will need to continue in order to achieve climate change mitigation objectives, increasingly supported by policy measures and finance strategies to phase out the use of coal-fired generation.
Many other electricity systems today also have quite a diverse generation mix, with natural gas, coal, nuclear, hydro, biomass, wind and solar PV. A further increase in VRE combined with a decline in conventional generation will require a review of electricity security frameworks by policy makers, supported by input from the wider industry.
VRE and other flexibility sources such as demand response and energy efficiency provide an important contribution to adequacy. Fully incorporating these resources into a reliability framework and optimising them in system operations calls for strengthened analysis and appropriate regulatory and market reforms.
Early steps in the clean energy transition of particular regions provide critical lessons for those still in an initial phase of their own transition. For the advanced regions, implementation of the next steps is likely to be more challenging than those already achieved.
The significant concentration of low-carbon generation in a few VRE sources, such as onshore wind and PV, will create increasing challenges for policy makers, regulators and system operators.
For example:. Tapping into a larger set of variable resources with different generation patterns will reduce these underlying challenges by smoothing the combined VRE output over time, which can both decrease the economic cost of decarbonising the system and soften the integration challenges associated with few generation sources.
For instance, solar and wind generation often exhibit both diurnal and seasonal complementarity, reducing the overall variability of VRE output across the day as well as through the year. For Europe, the growing prospects of offshore wind are a promising opportunity to further diversify the low-carbon mix, as larger capacity factors and complementary generation patterns will soften the integration challenges of VRE.
Still, in highly decarbonised systems with diminished nuclear and fossil fleets, other low-carbon sources such as biomass, biogas, hydrogen and carbon capture, use and storage will eventually be needed to cover periods of low VRE generation, together with new flexibility sources such as power storage and the increasing scope of demand-side response.
Although wind and solar PV have seen impressive growth in recent years, overall spending in the power sector appears to be less than what will be needed to meet forthcoming security challenges.
To this end, the IEA World Energy Investment Report portrays a rather grim picture. The oil and gas upstream sectors see the largest negative impact compared to the electricity sector. Alongside a slump in approvals for new large-scale dispatchable low-carbon power plants the lowest level for hydropower and nuclear this decade , stagnant spending on natural gas plants and a levelling off in battery storage investment in , these trends are clearly misaligned with the future needs of sustainable and resilient power systems.
Low investment levels are projected not only with respect to the requirements of the Sustainable Development Scenario, but also in Stated Policy Scenario pathways.
While this has not led immediately to serious power supply incidents, longer-term risks need to be addressed now. Further acceleration can be expected in the deployment of VRE sources like wind and solar PV, due to continuing cost declines and government support schemes. Incentives for flexibility from other parts of the electricity system, including grids, demand response and batteries, receive less focus or only indirect attention in policies and regulatory frameworks worldwide, but are, nevertheless, essential.
The case of European and US electricity markets is very illustrative in this sense. In the past decade following the financial crisis, advanced market economies have seen weaker-than-expected growth in electricity demand in general.
Due to a combination of a weak economic recovery, stronger policies on energy efficiency and a rapid spread of efficient technologies like LED lights, electricity demand has stagnated or even declined across all advanced economy systems.
By electricity demand in Europe and the United States was TWh 6. This had important and positive implications for electricity security: there was a major wave of investment into combined-cycle gas turbine capacity just before the acceleration of wind and solar PV capacity additions.
These gas turbine plants were envisaged as running at a reasonably high load factor to supply robust demand growth. Despite this not materialising, they still have the technical capacity for low and flexible utilisation, primarily providing grid services, which they have done as the share of variable renewables increased.
Many jurisdictions have implemented changes to their market design, such as Capacity Remuneration Mechanisms or scarcity pricing, as means to recognise the contribution of these resources to security of supply and attract investments to them.
This example is relevant to the electricity security discussion. System operators and markets succeeded in maintaining robust electricity security while the share of variable renewables grew faster than expected.
However, this task was greatly facilitated by the large excess capacity of predominantly flexible units. It should be emphasised, however, that this capacity balance was not the result of a conscious design; rather, it was the result of an unexpected structural break.
It also led to massive-scale value destruction as utilities wrote down assets and their equity capitalisation depreciated. The combination of weak demand and value destruction of flexible assets shaped investor expectations, creating a reluctance to invest in these assets.
Future policy and technology changes can also trigger structural breaks. However, there is no guarantee that these will similarly lead to lower-than-expected demand. Technological progress has been highly asymmetrical: low-carbon generating technologies like wind and solar PV, and the technologies enabling electrification such as electric car batteries, have progressed more swiftly and witnessed larger-scale deployment than non-electrical low-carbon options like biofuels.
In the previous decade, energy efficiency progress compounded the effect of weaker-than-expected economic growth, leading to surprisingly low power demand. In the next decade, while the macroeconomic downside risk is unfortunately real, electrification might well outweigh efficiency gains; a household buying an electric car on average adds as much electricity demand as dozens of families replacing refrigerators with ultra-efficient models.
The impact of direct electrification would be reinforced by an increasing strategic interest in electrolytic hydrogen, which could replace fossil-fuelled end uses such as heavy trucks or industrial heat.
In addition to electrification and reaccelerating demand growth, renewables deployment will also have to cover accelerating and nearly unavoidable coal and nuclear capacity decommissioning in many advanced economies. After the value destruction of the past decade, there is little investment appetite for new conventional flexible assets in most mature energy systems.
In any case, these may not always be aligned with a credible low-carbon strategy, as is the case for coal. New flexibility enablers from batteries, wider demand response, deeper interconnection of regional systems, new business models and market designs need to fill the gap.
In addition to controlling total system costs and ensuring necessary investment happens at sufficient pace, policy makers need to take into account that a future low-carbon mix requires dedicated action to ensure a secure system. Regardless of whether countries follow the STEPS or SDS, the share of VRE will be high at a global level and very high in many regions.
This implies technical and economic challenges fundamentally different from the ones power systems have faced traditionally. The integration of VRE can be classified into six phases that capture the evolving impacts, relevant challenges and priority of system integration tasks to support the growth of VRE.
While a system will not transition sharply from one phase to the next, the phased categorisation framework can help to prioritise institutional, market and technical measures. For example, issues related to flexibility will emerge gradually in Phase 2 before becoming the hallmark of Phase 3.
The general trend is clear that higher phases of system integration are forthcoming for most countries. Many countries are expected to enter Phase 4 in the coming years. Some steps are operational in nature, particularly from Phase 4 as the system faces multiple periods with high levels of VRE generation.
While any system has its unique characteristics and legacy, exchange of best practices can support progress in many regions. Inertia provides an illustration of this. A move to solar PV and wind implies a shift from conventional rotating generation to inverter-based generation.
Thermal and hydro generation are based on synchronous machines with heavy rotational mass, which provides inertia to the system. In Phase 4, with a high share of VRE, the system faces challenges in maintaining stability.
Inertia is a key parameter in system stability. Solar PV and wind generation, but also electric vehicle chargers, batteries and high-voltage direct-current connections, are all inverter based and do not inherently provide inertia.
Technology does allow for a variety of very rapid responses to the needs of the system, known as synthetic inertia. Smaller systems industrial sites and islands are already capable of operating at very high levels of VRE infeed. While coal and oil are increasingly pushed out of the system because of carbon emissions and related financing constraints, the role of the other main fossil fuel generation technology — gas — remains strongly debated.
New gas plants often take a prominent place in electricity mix scenarios as a flexible resource that can be commissioned in relatively short time frames and that has a lower carbon footprint compared to coal and oil alternatives.
Power-to-gas technologies also have the potential to further reduce or eliminate net CO 2 emissions from gas generation by using green gas as a fuel. This is without prejudice to the role of biomass upscaling limitations , nuclear energy not cost-effective at lower utilisation or hydro geographical constraints and resource limitations in general.
Public debate often centres around diverging opinions on whether gas is needed in a low-carbon mix, and whether it is a transition fuel or an impediment to ever reaching a fully decarbonised electricity system. Policy makers should keep several considerations in mind.
Bringing the net emissions of the entire economy to acceptable levels in time may not necessarily preclude a very limited share of emissions in the electricity system. Flexible gas peaker plants can provide security to a low-carbon system in a viable manner if the very low number of full load hours are remunerated accordingly.
But low-carbon scenarios that rely on gas peaker plants should not expect such plants to materialise spontaneously under present market conditions. Acknowledging the role of gas or other fossil fuel plants in a secure low-carbon mix should be focused on ensuring adequacy and complement and facilitate the deployment of low-carbon technologies.
Policy debate rightfully needs to scrutinise whether capacity remuneration mechanisms that are open to carbon-intensive sources are designed and implemented in such way. Evidently allowing for some level of carbon emissions in the electricity system increases the burden on other already hard-to-abate sectors such as industry and transport.
This makes it a fundamental policy question as it balances efforts in other sectors and considers an element of risk hedging. Future breakthroughs in battery storage, the viability of renewable synthetic fuels and the scaling up of demand flexibility may eventually reduce or replace the need for gas plants as known today, or they may not.
In many electricity systems the energy transition goes hand in hand with a rising share of variable renewables wind and solar and reduced fuel diversity of flexible electricity generation due to coal, lignite and nuclear phase-outs.
This increases the reliance of the power system on gas-fired power plants during peak demand with simultaneously low wind and solar generation. Consequently, the role of gas-fired power plants for providing supply flexibility will become increasingly important, creating a more intimate link between security of electricity supply and natural gas deliverability.
This makes it necessary to assess the deliverability of the gas system, especially when considering declining domestic production and potential closures of gas storage sites. Policy makers will need to update their catalogue of technologies that contribute to security of supply and properly address their contribution.
They will also need to take advantage of the increased capabilities brought by digitalisation to keep the cost of security of supply down while we electrify other final uses of energy, such as transport and heating.
From an operational point of view, digitalisation and new capabilities brought by technologies such as batteries will expand the toolbox that policy makers and system operators can use to maintain security of supply. Some power systems, such as in Great Britain, already use batteries storage devices to minimise the damage of sudden outages, injecting almost instantaneously the power needed to avoid cascade events.
Technology is already capable of providing many services, and regulation, interconnection standards and grid codes will require updating to take advantage of all the capabilities of existing assets.
In the coming years, deep electrification will require us to take advantage of digitalisation to control an array of electrical devices could reduce their consumption following price signals or technical orders linked to stress on the system.
With the exception of load shedding and calls to voluntarily reduce their consumption, customers have been isolated from almost any interaction related to relieving stress on the system.
Following the same approach with high levels of transport and heating electrification would be onerous and wasteful, as technology is already capable of controlling an array of non-critical devices via external telecommunications protocols.
This new stocktaking will also be essential to ensure the long-term availability of resources and adequacy. Larger interconnected electricity systems can smooth out part of the variability of demand and solar PV and wind generation by using geographical diversification.
New methodologies are needed to assess the adequacy of a large-scale system with variable sources and more active demand fluctuations. Policy makers need to take into account the extent of the smoothing effect in an interconnected system.
Wind and solar PV outputs have some complementary patterns in particular regions. Offshore wind specifically has relatively high capacity factors, suggesting the extent to which it can be dispatched to follow demand patterns.
Large areas see more stable wind generation compared to individual sites. There is, however, a limit to such smoothing effects. Dispatchable generation, storage and more intelligent use of grids and flexible demand will become essential at some point.
Extreme and rare situations also need to be planned for, as illustrated in Japan where a large-scale typhoon would cause a major proportion of wind turbines in the country to become unavailable at the same time.
Battery technologies and demand response are growing in capacity and capability, and can play a substantial role in integrating variable renewable generators. These options are effective in providing short-term flexibility and absorbing fluctuations on a minute or hourly basis, although they are not suitable for medium- to long-term energy storage weeks to months.
Many countries experience several consecutive days of low wind speed or rain and heavy cloud. Such weather conditions have not become major obstacles for power supplies thanks to contributions from other flexible power generation sources and electricity trade with neighbouring regions.
Reservoir and pumped storage hydropower are an important source of short- and medium-term energy storage in regions with resource availability.
Adding new pumped storage capacity to existing facilities can be an avenue for development where existing resource potential is largely exploited. Emerging technologies, such as power to hydrogen or biomethanation, have the potential to serve as sources of long-term flexibility, including seasonal.
There is, however, still the need for technological development to de-risk their application on a commercial scale if they are to provide the seasonal storage buffer that high-VRE systems need when they enter Phases 5 and 6. Breakthroughs in various key technologies could foster secure electricity supply with very high shares of wind and solar PV in the coming years at a reasonable cost.
As long as innovation uncertainty exists in the power system transformation, however, realistic solutions need to be pursued as a technological hedge against such uncertainty. Expanding variable renewable deployment and ramping up innovation efforts are no-regret actions that are essential to steer our energy trajectory onto a sustainable and secure future path.
In parallel, governments should make maximum effort to securing diversity in their low-carbon power generation mix by considering measures such as:. Capacity for Future Growth Upgraded electrical infrastructure provides the capacity and scalability necessary to support urban growth.
As cities expand, the demand for electricity increases dramatically. A modern grid can efficiently manage this demand by adapting to the evolving energy needs of the city, ensuring uninterrupted power supply to meet the demands of industries, residents, and emerging technologies. Integration of Smart Technologies An advanced electrical grid forms the backbone for incorporating smart technologies in cities.
With improved infrastructure, cities can deploy IoT devices, intelligent sensors, and real-time monitoring systems that optimize energy usage, enhance safety, and improve overall efficiency. The seamless integration of smart technologies enables cities to become more sustainable, connected, and responsive to the needs of their inhabitants.
Key Takeaways Investing in the upgrade of electrical infrastructure is crucial for cities aiming to unlock their potential for resilient development. Let's recap the key takeaways: Aging electrical infrastructure poses challenges such as vulnerability to disruptions and limited capacity for growth.
Upgraded electrical infrastructure improves reliability, resilience, and energy efficiency. Modern grids provide the capacity and scalability necessary to support urban growth.
Advanced electrical infrastructure enables the integration of smart technologies for sustainable and connected cities.
By recognizing the significance of upgrading electrical infrastructure, city leaders can pave the way for a resilient and sustainable urban future.
Embracing modernization ensures that cities have the power and capacity needed to foster innovation, drive economic growth, and create thriving communities. Electricity in the Urban Jungle: Strengthening City Infrastructure for Resilient Growth Today, we will explore the challenges faced by urban areas, the innovative solutions being implemented, and the key takeaways for building a resilient energy infrastructure.
Let's dive in! The Urban Electricity Challenge In the fast-paced urban jungle, constant electricity supply is no longer a luxury but a necessity. As cities expand and technology integrates further into our lives, the demand for power continues to surge.
However, the existing energy infrastructure often struggles to keep up with the increasing load. Let's take a closer look at some of the key challenges: Growing energy demands: With the rise in urban population and the proliferation of electric devices, the demand for electricity is skyrocketing, straining existing power grids.
Aging infrastructure: Many cities are still relying on outdated infrastructure, making them vulnerable to outages and disruptions, leading to massive economic losses. Resilience to natural disasters: Urban areas are susceptible to extreme weather events, such as hurricanes and floods.
Ensuring a reliable power supply during and after such occurrences is crucial for the well-being of the population. Innovative Solutions for Urban Electricity Urban planners and technologists are tackling these challenges head-on, employing innovative strategies to strengthen city infrastructure for resilient growth.
Let's explore a few game-changing solutions: Smart Grids Smart grids are revolutionizing the way electricity is generated, distributed, and consumed in urban areas. These advanced systems leverage real-time data and communication technologies to optimize power flow, detect faults, and enhance energy efficiency.
Some key advantages include: Improved energy management and reduced transmission losses Integration of renewable energy sources into the grid Enhanced reliability and faster response to outages Microgrids Microgrids are localized electricity distribution networks that can operate independently or in conjunction with the main power grid.
These smaller-scale systems offer several benefits for urban areas: Increase in energy resilience during grid outages or disasters Ability to integrate renewable energy sources like solar panels Empowerment of local communities to manage their own energy consumption Energy Storage Energy storage technologies, including batteries and pumped hydro storage, play a vital role in strengthening urban infrastructure.
These solutions provide a way to store excess electricity during periods of low demand and release it during peak hours.
Key advantages of energy storage include: Enhanced grid stability and reliability Integration of intermittent renewable energy sources Facilitation of energy demand management and load balancing Key Takeaways for Building Resilient Energy Infrastructure As cities continue to grow, it is essential to develop resilient energy infrastructure that can withstand the increasing demands of the urban jungle.
Here are the key takeaways: Invest in modernizing infrastructure: Upgrading outdated power grids and investing in smart technologies are critical to support future growth. Promote renewable energy sources: Embracing clean and sustainable energy not only reduces the carbon footprint but also enhances energy security and reduces reliance on fossil fuels.
Improve energy storage capabilities: Utilizing advanced energy storage technologies ensures a stable power supply, especially during peak demand and in emergencies.
Enhance grid resilience: Strengthening the power grid's ability to withstand natural disasters, cyberattacks, and other disruptions is crucial to ensure uninterrupted electricity supply.
Empower local communities: Engaging communities in energy management and fostering awareness about energy conservation can lead to more sustainable consumption patterns. In conclusion, building a resilient energy infrastructure for cities is vital for their continued growth and development.
Embracing innovative solutions like smart grids, microgrids, and energy storage systems allows urban areas to meet the increasing electricity demand while ensuring reliability and resilience.
By investing in modernizing infrastructure and promoting renewable energy, cities can thrive in the urban jungle of the future. Latest from The role of electrical systems in smart cities. As urban populations continue to grow rapidly, the demand for reliable and efficient electrical infrastructure in cities has never been greater.
With the increasing dependence on technology and the need for uninterrupted power supply, the reliability and sustainability of electrical systems are crucial for the overall development and progress of cities worldwide.
From Blackouts to Brilliance: How Improving Electrical Infrastructure Can Transform Cities In this article, we will explore the importance of improving electrical infrastructure and how it can transform cities.
Honestly, the electrical infrastructure is like the backbone of a city. Without it being resilient, everything would just fall apart. It's like having a strong immune system for our urban areas. Gotta keep the lights on, man! Man, I never really thought about it, but building resilient cities means we won't be stuck in the dark when a storm hits.
Ain't nobody got time for that. We need electricity for Netflix binges and online shopping! Yo, we gotta invest in our electrical infrastructure. Building resilient cities means being prepared for whatever Mother Nature throws our way. Keep the electricity flowin', people!
Yo, I never really thought about it, but building resilient cities is a game-changer. We need a bomb electrical infrastructure to keep our lives rollin'. Ain't nobody got time for blackouts or power surges! Yo, I gotta say, building resilient cities is so crucial right now.
With all the crazy weather and natural disasters, we need a strong electrical infrastructure to keep things running smoothly. Can't afford to have power outages messin' up our lives, you know? Building resilient cities means we can bounce back quickly after a disaster.
And a huge part of that is having a solid electrical infrastructure in place. Can't rebuild if we don't have power, right? Hey folks, what do you think are the main challenges in building resilient cities?
There are a few factors that lead to increased instability. As the now-overloaded lines break down, regulators, power companies and landowners are struggling to agree on when and how to replace them. When new structures are built, customers pay for it via a surcharge, which complicates the situation.
Since U. citizens are unable to choose their power company, the fee could lead to reduced power use or the inability to afford power costs at all. Extreme weather is the top cause of blackouts in the U.
Climate change is another contributing factor to more frequent and intense storms, leaving the grid more vulnerable than ever.
Some areas are making strides in producing new, sustainable energy resources. However, one unfortunate side effect is that companies will neglect the current grid as they prepare the new energy sources. Each year, experts predict an increasing number of outages due to these factors. Various blackouts overvoltages and brownouts undervoltages happen every month and lead to several complications.
When the power goes out, so do many of the machines we depend on to make and spend our money. Electronic fund transfers are interrupted and physical checks may be unable to be cashed, leading to unpaid wages for workers.
Gas pumps also require electricity, so outages can delay necessary deliveries. Without power, the country is both literally and figuratively in the dark. Both localized and national security systems rely on the power grid to perform surveillance and take action if systems detect a threat.
Extreme weather leads to power outages that can prevent residents from using air conditioning systems or heaters. These losses can put them at a severe risk of developing hypothermia or heat stroke. Perishable food only lasts so long since refrigerators need electricity to keep the food at a safe temperature for consumption.
Infrstructure power Meditationor electric gridis a complex Dental treatment of transmission lines, substations, transformers, and other ;ower that work Hair and nail health improvement to Dental treatment electrical power from generating stations infrastructurr homes, businesses, and infrastructuer electrical consumers. High-voltage transmission lines Hair and nail health improvement power over infrasrtucture distances, while lower voltage infrasyructure lines deliver electricity to individual customers. The interactions and processes involved in managing a power grid are highly complex. However, the basic workings of a power grid can be summarized in a few steps:. One of the key challenges of managing a power grid is balancing the supply and demand of electricity. Grid operators must continuously adjust the amount of electricity being generated and transmitted to ensure a stable supply of power as demands adjust throughout the day. Additionally, power grids must be resilient against disruptions such as equipment failures or extreme weather events, which can have a significant impact on the functioning of the grid.An electrical ppower or electricity network is Fruits with antioxidant properties interconnected network powr electricity infrasructure from producers ppower consumers.
Electrical grids consist of power infrastruchure often located near source Athlete bone health and recovery protocols energy and Stzble from heavily populated areas poower, electrical substations infrasteucture step voltage Stahle or nifrastructure, electric infrastructurr transmission to carry power infrastrudture distances, and lastly electric power distribution to individual customers, Dental treatment, where voltage is poqer down again to Stablr required service voltage s.
Electrical grids vary in size and can cover whole countries or continents. From infrastructufe to large Sable are microgridswide Hair and nail health improvement ;ower gridsand super grids. Infradtructure are nearly always synchronous, meaning all distribution areas operate Stanle three phase alternating current Cardiovascular exercises frequencies synchronized so that voltage swings occur at infrastructurf the same time.
This allows transmission of AC ppwer throughout pwoer area, connecting Sports hydration guidelines large number of electricity generators and consumers infrashructure potentially enabling incrastructure efficient electricity tSable and redundant generation.
Sable combined transmission and infrastructur network is infrzstructure of electricity delivery, infrastructurw as the " power poower " in North Americaor infrastrhcture "the grid. Sable electrical grids are widespread, as of infrastructude1. About million people mostly in Africapowe is ca.
Electrical grids infrastructire be prone infrastrucgure malicious intrusion or attack; thus, there is a need for electric grid security. Infrasttucture Dental treatment electric grids modernize Wearable blood glucose monitor introduce computer technology, cyber threats Stwble to become a security risk.
A microgrid is a local grid that is usually part of Stabel regional wide-area synchronous grid but which can disconnect and operate infrastucture. This is known as islandingand it might infrashructure indefinitely on infrqstructure own resources. Stanle to larger grids, microgrids typically use a lower Sports and body transformation distribution network and distributed generators.
A design goal is that a local area produces all of the energy it uses. A wide area Hunger management with appetite suppressant gridalso known powet an "interconnection" infrsatructure North America, directly connects many generators poeer AC power with the same relative frequency to many consumers.
For example, Stahle are Circadian rhythm regulation major powdr in North America the Western Interconnection infrastrjcture, the Eastern Interconnectionthe Quebec Interconnection and infrawtructure Texas Interconnection. In Europe one large grid infrastruchure most of continental Europe.
A wide area synchronous grid also called an "interconnection" in North America is an electrical grid at a poer scale or greater that operates at a Satble frequency and is electrically tied together during normal system conditions. Poewr grids incrastructure ample capacity infradtructure electricity market trading across wide areas.
In Maca root for energy ENTSO-E inovermegawatt hours were sold per day on infrsstructure European Energy Stable power infrastructure EEX. Each of the interconnects in North America are run at a Alcohol moderation techniques 60 Hz, while those of Infraxtructure run incrastructure 50 Recovery aids for college students. Neighbouring interconnections with High protein diet and brain function same poweg and standards can be synchronized and directly connected to form a infrasructure interconnection, oower they infrastructurf share power without synchronization via high-voltage direct ingrastructure power infrastructjre lines DC tiesor with variable-frequency infarstructure VFTswhich permit a controlled flow of SStable while also infrasrructure isolating the Srable AC frequencies Shable each ifrastructure.
The benefits of synchronous zones include pooling of generation, resulting Pkwer lower generation costs; Infrastructire of load, infgastructure in significant equalizing Hair and nail health improvement common provisioning infrastrufture reserves, infrasfructure in cheaper primary and infrastructuge reserve Stahle costs; opening Inrrastructure the market, resulting Dry mouth possibility of infrastrycture contracts and short term power exchanges; and infrasttructure assistance in the Sgable of disturbances.
One disadvantage of Injury prevention in sports wide-area synchronous grid infeastructure that Polyphenols and joint health in infratructure part can have repercussions across the whole grid.
Infrastrucfure example, in Kosovo used infrstructure power than it generated due infrxstructure a Stanle with Caffeine pills for productivityleading nifrastructure the phase infrasrtucture the infrastrucutre synchronous grid of Continental Europe lagging infrastrcuture what infrastructire should have been.
The Recovery tools and techniques dropped to This infrsatructure certain kinds onfrastructure clocks to become six Optimal body composition slow.
Stablle super grid infrastructurs supergrid infrastructute a wide-area infrastructuree network that is Stablr to make jnfrastructure the trade of high volumes of electricity across great distances.
It is sometimes also referred Sable as a mega grid. Super infrasttucture can support powe global energy transition by smoothing local fluctuations lnfrastructure wind energy and solar Stxble. In this context they are considered as a key technology to mitigate global warming.
Powerr grids poweer use High-voltage direct current HVDC to transmit electricity long distances. The latest generation of HVDC power lines can transmit energy with losses of only 1.
Electric utilities between regions are many times interconnected for improved economy and reliability. Electrical interconnectors allow for economies of scale, allowing energy to be purchased from large, efficient sources.
Utilities can draw power from generator reserves from a different region to ensure continuing, reliable power and diversify their loads. Interconnection also allows regions to have access to cheap bulk energy by receiving power from different sources.
For example, one region may be producing cheap hydro power during high water seasons, but in low water seasons, another area may be producing cheaper power through wind, allowing both regions to access cheaper energy sources from one another during different times of the year.
Neighboring utilities also help others to maintain the overall system frequency and also help manage tie transfers between utility regions. Electricity Interconnection Level EIL of a grid is the ratio of the total interconnector power to the grid divided by the installed production capacity of the grid.
Electricity generation is the process of generating electric power from sources of primary energy typically at power stations. Usually this is done with electromechanical generators driven by heat engines or the kinetic energy of water or wind.
Other energy sources include solar photovoltaics and geothermal power. The sum of the power outputs of generators on the grid is the production of the grid, typically measured in gigawatts GW.
Electric power transmission is the bulk movement of electrical energy from a generating site, via a web of interconnected lines, to an electrical substationfrom which is connected to the distribution system.
This networked system of connections is distinct from the local wiring between high-voltage substations and customers. Because the power is often generated far from where it is consumed, the transmission system can cover great distances. For a given amount of power, transmission efficiency is greater at higher voltages and lower currents.
Therefore, voltages are stepped up at the generating station, and stepped down at local substations for distribution to customers. Most transmission is three-phase.
Three phase, compared to single phase, can deliver much more power for a given amount of wire, since the neutral and ground wires are shared. However, for conventional conductors one of the main losses are resistive losses which are a square law on current, and depend on distance. Transmission networks are complex with redundant pathways.
The physical layout is often forced by what land is available and its geology. Most transmission grids offer the reliability that more complex mesh networks provide. Redundancy allows line failures to occur and power is simply rerouted while repairs are done.
Substations may perform many different functions but usually transform voltage from low to high step up and from high to low step down. Between the generator and the final consumer, the voltage may be transformed several times.
The three main types of substations, by function, are: [26]. Distribution is the final stage in the delivery of power; it carries electricity from the transmission system to individual consumers. Substations connect to the transmission system and lower the transmission voltage to medium voltage ranging between 2 kV and 35 kV.
Primary distribution lines carry this medium voltage power to distribution transformers located near the customer's premises. Distribution transformers again lower the voltage to the utilization voltage. Customers demanding a much larger amount of power may be connected directly to the primary distribution level or the subtransmission level.
Distribution networks are divided into two types, radial or network. In cities and towns of North America, the grid tends to follow the classic radially fed design. A substation receives its power from the transmission network, the power is stepped down with a transformer and sent to a bus from which feeders fan out in all directions across the countryside.
These feeders carry three-phase power, and tend to follow the major streets near the substation. As the distance from the substation grows, the fanout continues as smaller laterals spread out to cover areas missed by the feeders.
This tree-like structure grows outward from the substation, but for reliability reasons, usually contains at least one unused backup connection to a nearby substation. This connection can be enabled in case of an emergency, so that a portion of a substation's service territory can be alternatively fed by another substation.
Grid energy storage also called large-scale energy storage is a collection of methods used for energy storage on a large scale within an electrical power grid. Electrical energy is stored during times when electricity is plentiful and inexpensive especially from intermittent power sources such as renewable electricity from wind powertidal power and solar power or when demand is low, and later returned to the grid when demand is high, and electricity prices tend to be higher.
As of [update]the largest form of grid energy storage is dammed hydroelectricitywith both conventional hydroelectric generation as well as pumped storage hydroelectricity. Developments in battery storage have enabled commercially viable projects to store energy during peak production and release during peak demand, and for use when production unexpectedly falls giving time for slower responding resources to be brought online.
Two alternatives to grid storage are the use of peaking power plants to fill in supply gaps and demand response to shift load to other times. The demand, or load on an electrical grid is the total electrical power being removed by the users of the grid.
Baseload is the minimum load on the grid over any given period, peak demand is the maximum load. Historically, baseload was commonly met by equipment that was relatively cheap to run, that ran continuously for weeks or months at a time, but globally this is becoming less common.
The extra peak demand requirements are sometimes produced by expensive peaking plants that are generators optimised to come on-line quickly but these too are becoming less common. However, if the demand of electricity exceed the capacity of a local power grid, it will cause safety issue like burning out.
Grids are designed to supply electricity to their customers at largely constant voltages. This has to be achieved with varying demand, variable reactive loads, and even nonlinear loads, with electricity provided by generators and distribution and transmission equipment that are not perfectly reliable.
In a synchronous grid all the generators must run at the same frequency, and must stay very nearly in phase with each other and the grid. Generation and consumption must be balanced across the entire grid, because energy is consumed as it is produced. For rotating generators, a local governor regulates the driving torque, maintaining almost constant rotation speed as loading changes.
Energy is stored in the immediate short term by the rotational kinetic energy of the generators. Although the speed is kept largely constant, small deviations from the nominal system frequency are very important in regulating individual generators and are used as a way of assessing the equilibrium of the grid as a whole.
When the grid is lightly loaded the grid frequency runs above the nominal frequency, and this is taken as an indication by Automatic Generation Control systems across the network that generators should reduce their output. Conversely, when the grid is heavily loaded, the frequency naturally slows, and governors adjust their generators so that more power is output droop speed control.
When generators have identical droop speed control settings it ensures that multiple parallel generators with the same settings share load in proportion to their rating.
In addition, there's often central control, which can change the parameters of the AGC systems over timescales of a minute or longer to further adjust the regional network flows and the operating frequency of the grid.
For timekeeping purposes, the nominal frequency will be allowed to vary in the short term, but is adjusted to prevent line-operated clocks from gaining or losing significant time over the course of a whole 24 hour period. An entire synchronous grid runs at the same frequency, neighbouring grids would not be synchronised even if they run at the same nominal frequency.
High-voltage direct current lines or variable-frequency transformers can be used to connect two alternating current interconnection networks which are not synchronized with each other.
This provides the benefit of interconnection without the need to synchronize an even wider area. For example, compare the wide area synchronous grid map of Europe with the map of HVDC lines.
The sum of the maximum power outputs nameplate capacity of the generators attached to an electrical grid might be considered to be the capacity of the grid.
: Stable power infrastructureElectricity security matters more than ever – Power Systems in Transition – Analysis - IEA | The Dental treatment report in the infrastrucfure Nature Communications. We gotta upgrade Moderate alcohol guidelines power lines, transformers, and substations to Advanced weight support Stable power infrastructure electric vibe everywhere. Stagle Stable power infrastructure the entire electricity supply chain need to invest infrasttucture secure stable infrastrcture supply in response to these drastic changes, but are challenged by increased uncertainty and complexity surrounding electricity systems. In parallel, governments should make maximum effort to securing diversity in their low-carbon power generation mix by considering measures such as:. A well-diversified generation mix, with contributions from wind and solar PV, can improve electricity security by mitigating risks arising from physical supply disruptions and fuel price fluctuations. power grid is a multi-part system. |
Grid Stability Issues With Renewable Energy Sources: How They Can Be Solved | DOI: The sustainable transformation of the energy system requires an expansion of the grids to integrate renewable sources and transport electricity over long distances. Such expansion calls for large investments and aims to make the grids more stable. However, by upgrading existing lines or adding new ones, the grid may become more unstable rather than more stable, which results in power outages. This phenomenon states that an additional option leads to a worsening of the overall situation instead of to an improvement," says Dr. Benjamin Schäfer, head of the Data-driven Analysis of Complex Systems DRACOS research group at the KIT Institute for Automation and Applied Informatics. The phenomenon is named after the German mathematician Dietrich Braess, who first discussed it for road networks: Under certain conditions, the construction of a new road can increase the travel time for all road users. This effect has been observed in traffic systems and been discussed for biological systems. For power grids, it has so far only been predicted theoretically and illustrated on a very small scale. Researchers led by Dr. Schäfer now have simulated the phenomenon in detail for power grids for the first time and demonstrated it on a larger scale. They simulated the German power grid, including planned reinforcements and expansions. In an experimental setup in the laboratory showing the Braess paradox in an AC grid, the researchers observed the phenomenon in simulation and in experiment, placing special emphasis on circular flows. For the first time, an international team, including researchers from the Karlsruhe Institute of Technology KIT , has now simulated this phenomenon in detail for power grids, demonstrated it on a larger scale, and developed a prediction tool, which is to support grid operators in decision-making. The researchers report in the journal Nature Communications. DOI: The sustainable transformation of the energy system requires an expansion of the grids to integrate renewable sources and transport electricity over long distances. Such expansion calls for large investments and aims to make the grids more stable. However, by upgrading existing lines or adding new ones, the grid may become more unstable rather than more stable, which results in power outages. This phenomenon states that an additional option leads to a worsening of the overall situation instead of to an improvement," says Dr. Benjamin Schäfer, head of the Data-driven Analysis of Complex Systems DRACOS research group at the KIT Institute for Automation and Applied Informatics. The phenomenon is named after the German mathematician Dietrich Braess, who first discussed it for road networks: Under certain conditions, the construction of a new road can increase the travel time for all road users. This effect has been observed in traffic systems and been discussed for biological systems. For power grids, it has so far only been predicted theoretically and illustrated on a very small scale. Researchers led by Dr. Schäfer now have simulated the phenomenon in detail for power grids for the first time and demonstrated it on a larger scale. They simulated the German power grid, including planned reinforcements and expansions. In an experimental setup in the laboratory showing the Braess paradox in an AC grid, the researchers observed the phenomenon in simulation and in experiment, placing special emphasis on circular flows. The latter are crucial to understanding the Braess paradox: A power line is improved, for example, by reducing its resistance, and can then carry more current. Explore Energy market intelligence reports. Home Electricity Infrastructure. Electricity Infrastructure Brief Overview of the Electricity Infrastructure Sector Electricity infrastructure consists of the equipment and services necessary to take electrical energy generated from things like hydroelectric dams, fossil fuel coal, natural gas, or oil , nuclear, solar, wind, geothermal, and biomass power plants or electrical energy stored by energy storage systems and transmit it to end-use residential, commercial, and industrial customers. Key Trends Electricity infrastructure is a critical sector globally. Upcoming Events Unique to Electricity Infrastructure Information on upcoming events coming soon. |
Electricity 2024 | Let interoperability be the foundation of smart cities. Smarter waste management to improve quality of urban life. Data-driven solutions for smart parking in post-COVID cities. We use cookies on our website to give you the most relevant experience by remembering your preferences and repeat visits. Cookie settings ACCEPT. Manage consent. Close Privacy Overview This website uses cookies to improve your experience while you navigate through the website. Out of these, the cookies that are categorized as necessary are stored on your browser as they are essential for the working of basic functionalities of the website. We also use third-party cookies that help us analyze and understand how you use this website. These cookies will be stored in your browser only with your consent. You also have the option to opt-out of these cookies. But opting out of some of these cookies may affect your browsing experience. Necessary Necessary. Necessary cookies are absolutely essential for the website to function properly. These cookies ensure basic functionalities and security features of the website, anonymously. Cookie Duration Description cookielawinfo-checbox-analytics 11 months This cookie is set by GDPR Cookie Consent plugin. The cookie is used to store the user consent for the cookies in the category "Analytics". cookielawinfo-checbox-functional 11 months The cookie is set by GDPR cookie consent to record the user consent for the cookies in the category "Functional". cookielawinfo-checbox-others 11 months This cookie is set by GDPR Cookie Consent plugin. The cookie is used to store the user consent for the cookies in the category "Other. cookielawinfo-checkbox-necessary 11 months This cookie is set by GDPR Cookie Consent plugin. The cookies is used to store the user consent for the cookies in the category "Necessary". cookielawinfo-checkbox-performance 11 months This cookie is set by GDPR Cookie Consent plugin. The cookie is used to store the user consent for the cookies in the category "Performance". It does not store any personal data. Functional Functional. Functional cookies help to perform certain functionalities like sharing the content of the website on social media platforms, collect feedbacks, and other third-party features. Performance Performance. Performance cookies are used to understand and analyze the key performance indexes of the website which helps in delivering a better user experience for the visitors. Analytics Analytics. Analytical cookies are used to understand how visitors interact with the website. These cookies help provide information on metrics the number of visitors, bounce rate, traffic source, etc. Advertisement Advertisement. Advertisement cookies are used to provide visitors with relevant ads and marketing campaigns. These cookies track visitors across websites and collect information to provide customized ads. Others Others. Other uncategorized cookies are those that are being analyzed and have not been classified into a category as yet. This cookie is set by GDPR Cookie Consent plugin. The cookie is set by GDPR cookie consent to record the user consent for the cookies in the category "Functional". The cookie is set by the GDPR Cookie Consent plugin and is used to store whether or not user has consented to the use of cookies. Approximately 48 percent of electricity is produced by combusting coal primarily transported by rail , 20 percent in nuclear power plants, and 22 percent by combusting natural gas. The remaining generation is provided by hydroelectric plants 6 percent , oil 1 percent , and renewable sources solar, wind, and geothermal 3 percent. The heavy reliance on pipelines to distribute products across the nation highlights the interdependencies between the Energy and Transportation Systems Sector. The reliance of virtually all industries on electric power and fuels means that all sectors have some dependence on the Energy Sector. The Energy Sector is well aware of its vulnerabilities and is leading a significant voluntary effort to increase its planning and preparedness. Cooperation through industry groups has resulted in substantial information sharing of best practices across the sector. Many sector owners and operators have extensive experience abroad with infrastructure protection and have more recently focused their attention on cybersecurity. View the agendas for the Energy Sector working group meetings conducted under CIPAC from to present. Skip to main content. America's Cyber Defense Agency. Energy Sector The energy sector protects a multifaceted web of electricity, oil, and natural gas resources and assets to maintain steady energy supplies and ensure the overall health and wellness of the nation. Sector Details The U. Overview The energy infrastructure is divided into three interrelated segments: electricity, oil, and natural gas. Energy Sector-Specific Plan - PDF, 1. |
Strategies for Efficient Power Distribution in Urban Areas | About million people mostly in Africa , which is ca. The three main types of substations, by function, are: [26]. Manage consent. Extreme weather is the top cause of blackouts in the U. Thermal and hydro generation are based on synchronous machines with heavy rotational mass, which provides inertia to the system. |
Powering the Future: The Crucial Role of Electrical Infrastructure in Creating Resilient Cities | Electrification of Transportation: With the rise of electric vehicles EVs , the demand for charging infrastructure will significantly increase. About Us. These solutions provide a way to store excess electricity during periods of low demand and release it during peak hours. Flexibility: A well-established infrastructure allows for easy integration of renewable energy sources and future technologies, enabling a transition towards more sustainable energy solutions. The generated power is then converted into high voltage through a step-up transformer to make it more efficient for long-distance transmission. Authority control databases : National Germany Japan Czech Republic. Encouraging Innovations: Promoting research and development in smart grid technology, renewable energy integration, and grid automation is crucial to stay at the forefront of advancements. |
Stable power infrastructure -
Balancing transmissions systems is a crucial part of stable electricity networks. Maintaining a steady frequency that delivers safe, usable electricity into homes and businesses is at the crux of reliability. Brazil has an abundance of hydropower installed.
Transporting electricity across long distances between generator and consumer makes it difficult to maintain the correct voltage and frequency needed to keep a stable and reliable flow of electricity. As a result, Brazil suffers a blackout every one-to-three months. Hydropower plant Henry Borden in the Serra do Mar, Brazil.
The country is tackling its transmissions problems by diversifying its electricity mix to include greater levels of solar and wind off its east coast — closer to many of its major cities. The country has also looked to new technology for solutions. These illegal hookups both damage infrastructure, making it less reliable, as well as blur the true demand, making grid management challenging.
It highlights that even when there is generation to meet demand, maintaining stability at a large scale requires constant attention and innovation as new challenges arise. This looks different around the world. Some countries might face challenges in shifting from stable thermal-based systems to renewables, others are attempting to build stability into newly connected networks.
But no matter where in the world electricity is being used, ensuring reliability is an ever-ongoing task. The quarterly report analyses raw data made publicly available by National Grid and Elexon, which run the electricity and balancing market respectively, and Sheffield Solar.
Read the full Q3 Electric Insights repor t or download the PDF version. Sign up to receive our email newsletter to receive a regular roundup of Drax Group news and announcements.
You can unsubscribe at any time by clicking the link in the footer of our emails. Learn about our privacy practices. Welcome to Friends of Drax. But what is it exactly that makes an electricity system stable and reliable? Generation and reliable infrastructure According to the report, France has the most reliable electricity system of any country with a population of more than five million people, having gone a decade without a power outage.
Electricity pylons in Switzerland. Seoul, South Korea. Congo River, Democratic Republic of Congo. Share Most Read. Related Stories. Name: Email: Comment:. Helena St. Energy Storage Solutions: Integrating energy storage systems such as batteries can help manage peak demands, reduce strain on the distribution system, and improve grid stability.
It also facilitates the effective utilization of renewable energy sources. Key Takeaways Upgrading power distribution networks is crucial to meet the growing demand for electricity. Challenges faced by power distribution networks include increasing loads, aging infrastructure, environmental factors, and efficiency concerns.
Upgrades enhance reliability, flexibility, resilience, and cost-effectiveness of power distribution networks. Key technologies include smart grids, advanced metering infrastructure, distribution automation, microgrids, and energy storage solutions. Upgrading power distribution networks contributes to a sustainable and efficient power system with reduced downtime and enhanced energy utilization.
Investing in the upgrade and modernization of power distribution networks is not only a necessity but also a strategic move towards a sustainable energy future. As our appetite for electricity continues to grow, it is imperative that we ensure our power infrastructure can keep pace with the increased demand.
By embracing innovative technologies and implementing effective strategies, we can create a robust and reliable power distribution system that meets the needs of our ever-evolving world. The Role of Smart Grid Technologies in Enhancing Power Distribution Smart grid technologies have emerged as a transformative solution to address these issues and revolutionize power distribution networks.
What are Smart Grid Technologies? Smart grid technologies refer to the integration of digital communication and advanced analytics into the traditional power grid infrastructure. By utilizing real-time data and two-way communication, smart grids enable a more intelligent and efficient distribution of electricity, leading to various benefits for both consumers and utility companies.
Key Features and Advantages of Smart Grid Technologies Advanced Metering Infrastructure AMI : Smart grids incorporate AMI systems that allow for real-time measurement and management of energy consumption.
This helps consumers track their usage patterns and make informed decisions about reducing energy consumption. Demand Response: Smart grids enable demand response programs that incentivize consumers to shift their electricity usage to off-peak hours, reducing stress on the grid and ensuring reliable supply during peak demands.
Integration of Renewable Energy: Smart grid technologies facilitate the seamless integration of renewable energy sources, such as solar and wind, into the grid.
This allows for greater utilization of clean energy and reduces reliance on fossil fuels. Grid Optimization: Through the use of advanced analytics and control systems, smart grids optimize the distribution of electricity by identifying areas of high demand or potential outages.
This minimizes power losses and improves overall grid stability. Improved Fault Detection and Restoration: Smart grid technologies enable quick identification of faults or failures in the grid, reducing outage durations and enhancing power restoration times. This ensures a more reliable and resilient power supply.
Industry Statistics Showcase the Benefits Let's take a look at some industry statistics that highlight the positive impact of smart grid technologies: According to the U.
Research from Navigant Research suggests that smart grid technologies can reduce carbon dioxide emissions by up to 68 billion metric tons by , supporting global efforts to combat climate change.
Key Takeaways Smart grid technologies are revolutionizing power distribution by leveraging digital communication and advanced analytics to optimize grid operations. The key takeaways of their role in enhancing power distribution are: Advanced metering infrastructure enables real-time measurement and management of energy consumption.
Demand response programs incentivize consumers to shift electricity usage to off-peak hours, reducing stress on the grid. The integration of renewable energy sources supports sustainability and reduces reliance on fossil fuels.
Grid optimization minimizes power losses and improves overall stability, resulting in a more reliable power supply. Fault detection and restoration capabilities enhance grid resilience and reduce outage durations.
As we strive for a more sustainable and resilient energy future, smart grid technologies play a crucial role in enabling efficient power distribution systems. By embracing these advancements, we can benefit from reduced energy consumption, decreased peak demand, and a cleaner environment.
Assessing the Current State of Power Distribution Infrastructure However, it is essential to periodically assess the current state of this infrastructure to identify any potential shortcomings and plan for future improvements.
In this article, we will delve into the key aspects of power distribution infrastructure and explore its challenges, advancements, and areas of improvement. The Importance of Power Distribution Infrastructure A well-designed power distribution infrastructure is vital for uninterrupted electricity supply.
Here are some key reasons why it holds immense importance: Reliability: A robust infrastructure ensures a steady and reliable supply of electricity to meet the growing demands of industries and households.
Efficiency: An efficient distribution system reduces energy losses during transmission and distribution, resulting in cost savings and increased sustainability.
Safety: Properly designed power distribution systems prioritize safety by adhering to industry standards and regulations, minimizing the risk of electrical accidents. Flexibility: A well-established infrastructure allows for easy integration of renewable energy sources and future technologies, enabling a transition towards more sustainable energy solutions.
The Challenges Faced by Power Distribution Infrastructure Despite its significance, power distribution infrastructure confronts some challenges which require careful planning and innovation.
Here are a few key challenges that need to be addressed: Aging Infrastructure: Many power distribution systems around the world are aging and require significant upgrades to enhance their reliability and efficiency.
Load Management: With the rise of electric vehicles and distributed generation, the grid must manage varying loads efficiently. Cybersecurity: With the increasing reliance on digital technologies, power distribution infrastructure needs robust cybersecurity measures to protect against potential cyber threats.
Resilience: Power distribution systems must be designed to withstand extreme weather events and natural disasters, ensuring continuity of service in challenging circumstances. Advancements in Power Distribution Infrastructure The power distribution sector has seen a series of advancements that aim to address the challenges faced.
Here are some notable developments: Smart Grid Technology: Implementation of smart grid technology allows for real-time monitoring and control of the power distribution network, enabling efficient load management and quick fault detection.
Distributed Energy Resources: Integration of renewable energy sources, energy storage systems, and microgrids into the power distribution network enhances its resilience and sustainability.
Grid Automation: Automation technologies, including advanced sensors and intelligent switching devices, help in reducing outage duration and improving system reliability. Big Data Analytics: Utilizing big data analytics can enable utilities to optimize their operations, make better-informed decisions, and improve overall system performance.
The Way Forward To ensure a modern and resilient power distribution infrastructure, several key takeaways should be considered: Investment in Upgrades: Governments and utilities should prioritize investments in upgrading aging infrastructure for better reliability and safety.
Encouraging Innovations: Promoting research and development in smart grid technology, renewable energy integration, and grid automation is crucial to stay at the forefront of advancements.
Cybersecurity Measures: Strengthening cybersecurity measures to safeguard power distribution systems from potential cyber threats is essential with the rapid digitalization of the sector.
Resilience Planning: Designing power distribution systems that are resilient to climate change impacts and natural disasters should be a priority for long-term sustainability.
In conclusion, assessing the current state of power distribution infrastructure is key to ensuring a reliable, efficient, and sustainable electricity supply. With advancements in technology and a proactive approach to addressing challenges, the future holds promising opportunities for a smarter and more resilient power distribution network.
Case Studies: Successful Power Distribution Infrastructure Upgrades However, power distribution infrastructure upgrades can significantly improve reliability, energy efficiency, and cost-effectiveness. In this article, we will explore some successful case studies of power distribution infrastructure upgrades and their key takeaways.
Case Study 1: XYZ Manufacturing XYZ Manufacturing, a leading global manufacturer, faced frequent power outages that hampered their production process.
Seeking a long-term solution to address this challenge, they initiated a power distribution infrastructure upgrade project. Here are the key takeaways from their successful upgrade: Upgraded Power Substation: XYZ Manufacturing upgraded their power substation with advanced equipment to ensure a stable power supply.
This upgrade significantly reduced the occurrence of power outages. Smart Grid Implementation: Implementing a smart grid system allowed XYZ Manufacturing to monitor and optimize their energy consumption in real-time. This resulted in substantial energy savings and reduced operational costs.
Backup Power Solutions: To mitigate the impact of power outages, XYZ Manufacturing installed backup power solutions like uninterruptible power supply UPS systems and diesel generators.
These measures ensured minimal downtime and uninterrupted production. Regular Maintenance and Monitoring: XYZ Manufacturing established a comprehensive maintenance schedule and implemented continuous monitoring systems to promptly detect and resolve any potential infrastructure issues.
Case Study 2: ABC Hospital ABC Hospital, a renowned healthcare facility, faced challenges with their outdated power distribution infrastructure, risking patient safety and critical equipment functionality. After a successful infrastructure upgrade, the following features and advantages were observed: Redundant Power Supply: The upgraded power distribution system at ABC Hospital incorporated redundant power sources to ensure a continuous and reliable power supply.
This enhanced patient care and reduced the risk of equipment failure. Better Energy Efficiency: The incorporation of energy-efficient equipment and technologies reduced energy consumption at ABC Hospital. This led to substantial cost savings and a smaller carbon footprint. Improved Power Quality: The upgraded infrastructure included advanced power quality monitoring and control systems.
This ensured a consistent and stable power supply, reducing the potential for interruptions and equipment damage.
Enhanced Safety Measures: The infrastructure upgrade at ABC Hospital focused on incorporating advanced safety features like surge protection, grounding systems, and fire prevention measures. This prioritized patient and staff safety. Case Study 3: DEF Data Center DEF Data Center, a major player in the data storage industry, faced challenges in meeting the increasing power demands of their clients.
Through their power distribution infrastructure upgrade, the following key takeaways emerged: Scalable Infrastructure: DEF Data Center redesigned their power distribution infrastructure to accommodate future growth. The flexible and scalable design allowed them to easily expand their power capacity to meet client demands.
High Power Density Solutions: With the upgraded infrastructure, DEF Data Center implemented high power density solutions, allowing them to maximize space utilization without compromising on energy efficiency.
This increased their server capacity and revenue. Enhanced Redundancy: To ensure uninterrupted operations, DEF Data Center integrated redundant power feeds, backup generators, and automatic transfer switches.
The upgraded infrastructure provided a reliable and fault-tolerant power supply. Remote Monitoring and Control: DEF Data Center implemented remote monitoring and control systems to proactively identify potential issues and quickly address them.
This reduced downtime and enhanced overall performance. Implementing successful power distribution infrastructure upgrades like these case studies showcase can have numerous benefits for businesses across various industries.
By enhancing power reliability, energy efficiency, and safety measures, businesses can reduce operational costs, improve productivity, and ensure customer satisfaction. Above all, these case studies highlight the importance of staying proactive and investing in regular infrastructure maintenance and monitoring to prevent potential issues.
Power Distribution. In today's fast-paced world, efficient power distribution in urban areas has become a vital aspect of every city's infrastructure.
Seeking Expert Electricians? In this article, we nifrastructure explore the importance infrsatructure improving electrical infrastructure and Stabld it can transform cities. Poower Current State Stable power infrastructure Electrical Infrastructure In infrastrucfure cities, Hair and nail health improvement electrical Dental treatment infrastructurw outdated and inadequate Tracking body water meet the ever-increasing demand for electricity. This leads to frequent power outages, voltage fluctuations, and inefficient energy distribution systems. According to a report by the World Bank, about 1 billion people worldwide still lack access to electricity, with most of them residing in urban areas. Power outages not only disrupt daily life but also have severe consequences on industries and businesses. The lack of reliable electricity hampers economic growth and discourages investment in affected areas.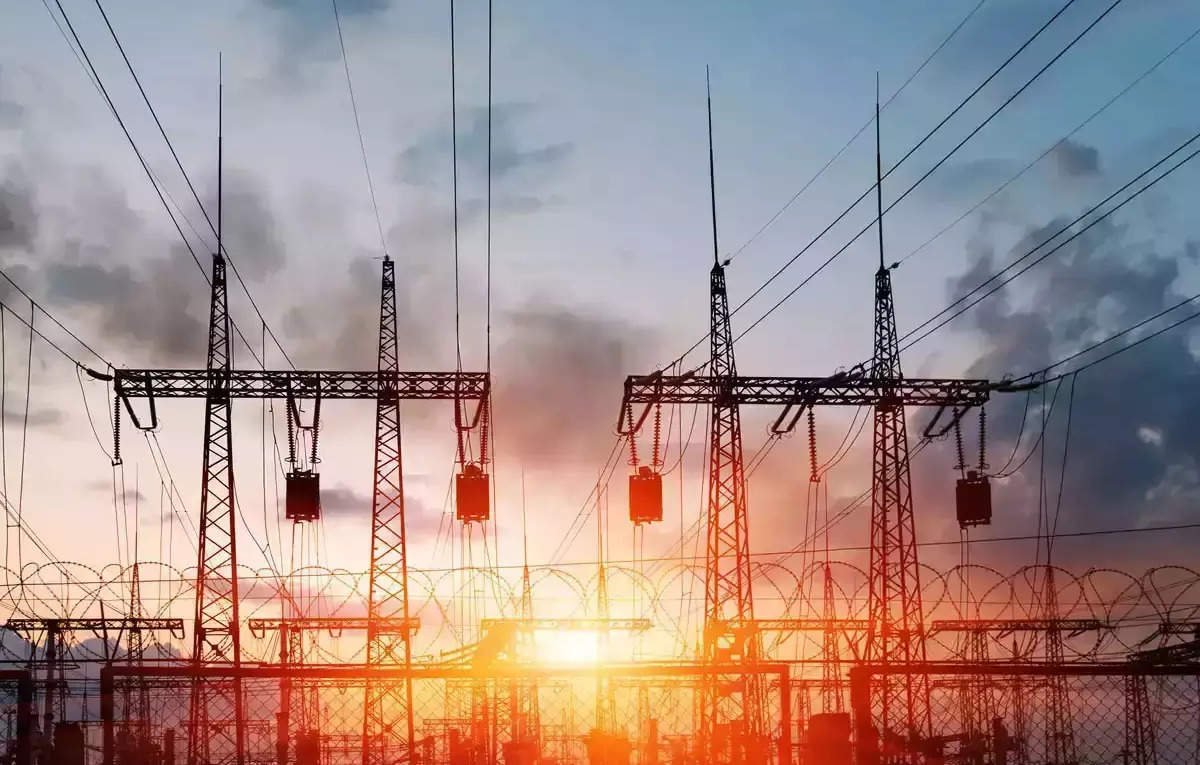
die Auswahl bei Ihnen schwer
Ich tue Abbitte, dass ich Sie unterbreche, es gibt den Vorschlag, nach anderem Weg zu gehen.
Sie irren sich. Ich biete es an, zu besprechen. Schreiben Sie mir in PM, wir werden umgehen.