Video
Glycolysis Made Easy!Glycolysis in cells -
A new pH-based etiopathogenic perspective and therapeutic approach to an old cancer question". doi : PMC PMID Research in Microbiology. Molecular Systems Biology. S2CID Archived from the original on Retrieved Nature Education. Journal of the History of Biology.
New Beer in an Old Bottle: Eduard Buchner and the Growth of Biochemical Knowledge. Valencia, Spain. Bios PDF. Archived from the original PDF on 18 November The Journal of Biological Chemistry. com: FREE online dictionary".
A new enzyme with the glycolytic function of 6-phosphofructokinase". Archives of Microbiology. Biochemistry 5th ed. Cengage Learning. ISBN Biochemistry 6th ed. New York: Freeman.
Biochemistry 3rd ed. Biotechnology Advances. Nature Reviews. In Roach RC, Wagner PD, Hackett PH eds. Advances in Experimental Medicine and Biology. Boston, MA: Springer US. The Biochemical Journal. Trends in Cell Biology. Current Opinion in Biotechnology.
August Current Opinion in Microbiology. The Journal of Physiology published Biochemistry Fourth ed. New York: W. Freeman and Company. Fundamentals of Biochemistry 2nd ed.
John Wiley and Sons, Inc. Oxford Reference Online: Oxford University Press. July Classes of regulatory isoenzymes in mammalian tissues". European Journal of Biochemistry. Current Topics in Cellular Regulation. Acta Pharmaceutica Sinica B. ISSN Lehninger principles of biochemistry 4th ed.
Archived from the original on May 19, Retrieved September 8, Part I. Biochemical uptake mechanism and its implication for clinical studies". Journal of Cancer Research and Clinical Oncology.
Seminars in Cancer Biology. Anti-Cancer Agents in Medicinal Chemistry. Journal of Child Neurology. Biochemistry and Molecular Biology Education. Glycolysis and Structure of the Participant Molecules". Metabolism , catabolism , anabolism.
Metabolic pathway Metabolic network Primary nutritional groups. Purine metabolism Nucleotide salvage Pyrimidine metabolism Purine nucleotide cycle. Pentose phosphate pathway Fructolysis Polyol pathway Galactolysis Leloir pathway.
Glycosylation N-linked O-linked. Photosynthesis Anoxygenic photosynthesis Chemosynthesis Carbon fixation DeLey-Doudoroff pathway Entner-Doudoroff pathway. Xylose metabolism Radiotrophism. Fatty acid degradation Beta oxidation Fatty acid synthesis. Steroid metabolism Sphingolipid metabolism Eicosanoid metabolism Ketosis Reverse cholesterol transport.
Metal metabolism Iron metabolism Ethanol metabolism Phospagen system ATP-PCr. Metabolism map. Carbon fixation. Photo- respiration. Pentose phosphate pathway. Citric acid cycle. Glyoxylate cycle. Urea cycle. Fatty acid synthesis. Fatty acid elongation.
Beta oxidation. beta oxidation. Glyco- genolysis. Glyco- genesis. Glyco- lysis. Gluconeo- genesis. Pyruvate decarb- oxylation. Keto- lysis. Keto- genesis. feeders to gluconeo- genesis. Light reaction. Oxidative phosphorylation. Amino acid deamination. Citrate shuttle.
MVA pathway. MEP pathway. Shikimate pathway. Glycosyl- ation. Sugar acids. Simple sugars. Nucleotide sugars. Propionyl -CoA.
Acetyl -CoA. Oxalo- acetate. Succinyl -CoA. α-Keto- glutarate. Ketone bodies. Respiratory chain. Serine group. Branched-chain amino acids. Aspartate group. Amino acids. Ascorbate vitamin C. Bile pigments. Cobalamins vitamin B Various vitamin Bs. Calciferols vitamin D. Retinoids vitamin A.
Nucleic acids. Terpenoid backbones. Bile acids. Glycero- phospholipids. Fatty acids. Glyco- sphingolipids. Polyunsaturated fatty acids. Endo- cannabinoids. Fructose-bisphosphate aldolase Aldolase A , B , C Triosephosphate isomerase.
Glyceraldehyde 3-phosphate dehydrogenase Phosphoglycerate kinase Phosphoglycerate mutase Enolase Pyruvate kinase PKLR , PKM2. Pyruvate carboxylase Phosphoenolpyruvate carboxykinase. Lactate dehydrogenase. Alanine transaminase. Glycerol kinase Glycerol dehydrogenase. Fructose 6-P,2-kinase:fructose 2,6-bisphosphatase PFKFB1 , PFKFB2 , PFKFB3 , PFKFB4 Bisphosphoglycerate mutase.
Authority control databases : National Germany Israel United States. Categories : Glycolysis Biochemistry Carbohydrates Cellular respiration Metabolic pathways. Hidden categories: CS1 maint: location missing publisher Articles with short description Short description matches Wikidata Articles needing additional references from June All articles needing additional references All articles with unsourced statements Articles with unsourced statements from May Commons category link is on Wikidata Articles with GND identifiers Articles with J9U identifiers Articles with LCCN identifiers.
Toggle limited content width. Glucose G6P F6P F1,6BP GADP DHAP 1,3BPG 3PG 2PG PEP Pyruvate HK PGI PFK ALDO TPI GAPDH PGK PGM ENO PK Glycolysis The metabolic pathway of glycolysis converts glucose to pyruvate via a series of intermediate metabolites.
Each chemical modification is performed by a different enzyme. Steps 1 and 3 consume ATP and steps 7 and 10 produce ATP.
Figure 2. The first half of glycolysis uses two ATP molecules in the phosphorylation of glucose, which is then split into two three-carbon molecules.
Step 1. The first step in glycolysis is catalyzed by hexokinase, an enzyme with broad specificity that catalyzes the phosphorylation of six-carbon sugars.
Hexokinase phosphorylates glucose using ATP as the source of the phosphate, producing glucosephosphate, a more reactive form of glucose.
This reaction prevents the phosphorylated glucose molecule from continuing to interact with the GLUT proteins, and it can no longer leave the cell because the negatively charged phosphate will not allow it to cross the hydrophobic interior of the plasma membrane.
Step 2. In the second step of glycolysis, an isomerase converts glucosephosphate into one of its isomers, fructosephosphate. An isomerase is an enzyme that catalyzes the conversion of a molecule into one of its isomers. This change from phosphoglucose to phosphofructose allows the eventual split of the sugar into two three-carbon molecules.
Step 3. The third step is the phosphorylation of fructosephosphate, catalyzed by the enzyme phosphofructokinase.
A second ATP molecule donates a high-energy phosphate to fructosephosphate, producing fructose-1,6-bisphosphate. In this pathway, phosphofructokinase is a rate-limiting enzyme. It is active when the concentration of ADP is high; it is less active when ADP levels are low and the concentration of ATP is high.
This is a type of end product inhibition, since ATP is the end product of glucose catabolism. Step 4. The newly added high-energy phosphates further destabilize fructose-1,6-bisphosphate.
The fourth step in glycolysis employs an enzyme, aldolase, to cleave 1,6-bisphosphate into two three-carbon isomers: dihydroxyacetone-phosphate and glyceraldehydephosphate. Step 5. In the fifth step, an isomerase transforms the dihydroxyacetone-phosphate into its isomer, glyceraldehydephosphate.
Thus, the pathway will continue with two molecules of a single isomer. At this point in the pathway, there is a net investment of energy from two ATP molecules in the breakdown of one glucose molecule.
So far, glycolysis has cost the cell two ATP molecules and produced two small, three-carbon sugar molecules. Both of these molecules will proceed through the second half of the pathway, and sufficient energy will be extracted to pay back the two ATP molecules used as an initial investment and produce a profit for the cell of two additional ATP molecules and two even higher-energy NADH molecules.
Figure 3. The second half of glycolysis involves phosphorylation without ATP investment step 6 and produces two NADH and four ATP molecules per glucose. We argue that the many different dynamics in glycolysis result from an interplay between a growth-dependent sugar uptake together with simple intrinsic enzyme regulation.
Citation: Rehberg M, Ritter JB, Reichl U Glycolysis Is Governed by Growth Regime and Simple Enzyme Regulation in Adherent MDCK Cells. PLoS Comput Biol 10 10 : e Received: March 25, ; Accepted: August 31, ; Published: October 16, Copyright: © Rehberg et al.
This is an open-access article distributed under the terms of the Creative Commons Attribution License , which permits unrestricted use, distribution, and reproduction in any medium, provided the original author and source are credited.
Funding: The authors were funded by the Max Planck Institute for Dynamics of Complex Technical Systems. The funders had no role in study design, data collection and analysis, decision to publish, or preparation of the manuscript.
Competing interests: The authors have declared that no competing interests exist. The primary metabolism of cells is essential for cell growth and maintenance.
Glycolysis is a central element of the primary metabolic activity and supplies anabolic pathways with precursors and cellular energy in form of ATP. The detailed in vitro characterization of glycolytic enzymes, such as hexokinase HK , phosphofructokinase PFK and pyruvate kinase PK , with respect to their catalytic properties in the presence of substrates, products and allosteric effectors represents an initial step towards a kinetic description of metabolic phenomena of cells [1] — [3].
Dynamic mathematical models of glycolysis have been developed for many different organisms such as Escherichia coli , yeast, or mammalian cells. Such models range from simple to full kinetic descriptions with the intention to study specific observations, e.
Although in many cases the existing experimental data sets do not allow for a full validation of highly complex models in a broad physiological context, there is a clear benefit regarding the integration of complex regulatory mechanisms, which helps to explain general phenomenological aspects that are typically found in the respective organism.
However, an apparently complex metabolic behavior must not result from complex regulatory mechanisms [16]. In case of glycolysis, it seems that few regulatory mechanisms dominate the dynamics of intracellular metabolite pools and readily explain salient features of experimental observations [17].
Furthermore, with an increasing number of powerful assays, e. to determine intracellular metabolite concentrations or to measure enzyme activities in yeast and animal cells e.
Based on the additional quantification of extracellular metabolite changes and cell number measurements a systematic analysis of basic dynamics of glycolysis for various cultivation conditions is possible.
Recently, we reported that adherent MDCK cells cultivated in two different media not only show similar and reproducible dynamics of many intracellular metabolite pools but also that changes in their concentrations are growth phase-dependent [22].
With the aim to elucidate the interplay between enzyme and growth regime-mediated regulation of glycolysis, a segregated cell growth model has been developed, which captures experimental observations during cell growth phases regarding number increase, diameter change and uptake of substrates [23].
Here, we couple this segregated cell growth model to a structured model which incorporates a simple kinetic description of glycolysis and focusses on a few well-known enzymatic properties to elucidate the control of glycolysis.
In addition, the linkage to the pentose phosphate pathway and the glycogenesis are taken into account. We evaluate the model's ability to reflect changes in intracellular metabolite pools for a variety of cultivation conditions using a single set of parameters.
This includes the transition from the exponential to the stationary cell growth phase, the fast replacement of medium by PBS at different time points of cultivation, and a substrate pulse experiment. Afterwards we discuss the influence of growth regime, changes in extracellular metabolite concentrations, and activity of key enzymes on the control of glycolysis.
In addition, aspects of hierarchical regulation are addressed which, taken together, improve our understanding of the metabolism of fast proliferating cells. Finally, options for the modulation of metabolic activity are evaluated regarding the design and optimization of cell culture processes as well as the study of metabolic diseases.
In three independent experiments, adherent MDCK cells were grown in 6-well plates with the serum-containing medium GMEM-Z, which provides sufficient amounts of extracellular substrates over the chosen cultivation time.
Therefore, cell growth occurs with maximum rate until the available surface becomes limiting [23]. The experimental data of intracellular metabolite pools is taken from Rehberg et al.
The model focuses on intermediates that were measured experimentally and is composed of a concise set of enzyme kinetics with few regulatory mechanisms.
A schematic overview of the considered enzyme reactions, the measured metabolite pools and maximum in vitro enzyme activities, and the coupling to the previously developed segregated model of cell growth [23] is given in Fig.
Green boxes represent metabolite pools that were quantified experimentally while white ones were not measured. Enzymes are shown as ellipses with blue background if the maximum enzyme activity was measured in vitro and with white background otherwise.
Reactions and their directions are shown as arrows. Dashed arrows represent allosteric regulation of enzymes by metabolites. GLC x extracellular glucose; GLC glucose; G6P glucose 6-phosphate; UGLC uridyl diphosphate glucose; R5P ribose 5-phosphate; PPP pentose phosphate pathway; F6P fructose 6-phosphate; F16BP fructose 1,6-bisphosphate; 3PG 3-phosphoglyceric acid; PEP phosphoenol pyruvate; HK hexokinase; UT UTP-glucosephosphate uridylyltransferase; G6PDH glucose 6-phosphate dehydrogenase; GPI glucose phosphate isomerase; ALD aldolase; ENO enolase; PK pyruvate kinase.
In particular, we assume that this metabolic status is reproducibly achieved in the preculture which is the case in all three cultivations and represents the metabolic starting point for the batch cultivation experiments Cult1—3.
Data and error bars represent mean and standard deviation of three wells. Dashed lines are the limit of quantification LOQ; data below LOQ marked in grey. Lines represent the respective simulation result based on the experiment-specific parameters in Table 1 and parameters in Table 2.
The maximum is reached at around 24 h of cultivation and roughly coincides with the onset of cell growth inhibition indicated by the grey bar. In the model, the peak results from high cell volume-specific glucose uptake rates and low maximum cell volume-specific enzyme activities.
Due to the tight coupling of cell growth to glycolysis, the model considers experiment-specific differences such as the cell number used for inoculation as well as the minimum and maximum mean cell diameter d m and d c ; Table 1 , which have the strongest effect on time point and height of the peak.
In addition, we performed a sensitivity analysis to investigate the influence of parameters and initial conditions on the model behavior supporting information, Fig. Differences in growth and metabolic status of cells used for inoculation of cultivation experiments indicate that the cells are obviously not identical.
It is therefore likely that not only the cell size but also the enzyme level E level differs to a certain degree. For the quantification of cell number-specific enzyme activities, Janke et al. Therefore, we introduce the E level as an experiment-specific value to modulate the maximum catalytic activity of every enzyme in the model with a range from 0.
The model suggests that the cells with the lowest diameters d m , d c , i. An exemplary intracellular flux from glycolysis into associated pathways is shown for Cult1 in Fig. During cell growth the flux through HK 3. The level of 3-phosphoglyceric acid 3PG follows the peak-like behavior of upper glycolysis albeit with a two-fold increase only, which is quite similar among the three cultivations Fig.
The data for phosphoenol pyruvate PEP are below the limit of quantification until 48 h of cultivation indicated by grey symbols but still support the hypothesis of a fast drop at the beginning of cultivation with a slow but steady increase until the stationary growth phase begins Fig.
Under consideration of these data points, the model similarly suggests a decrease and increase in PEP levels on the basis of an allosteric feed-forward activation of PK by F16BP. Otherwise, a straight line would suffice to describe the data. In the model, the lower part of glycolysis shows a four-fold increase in the activity during cell growth 5.
Interestingly, during cell maintenance most of the PPP metabolites, synthesized by glucose 6-phosphate dehydrogenase G6PDH , are fed back into glycolysis through the TATK mediated reactions Fig.
Hence, most of the glucose influx during the stationary growth phase is converted to pyruvate PYR. The products of the PPP are used for nucleotide and nucleic acid synthesis, production of macromolecules and yield NADPH for the synthesis of fatty acids.
Glycogenesis mainly generates glycogen and the relative net flux, which is branched off from glycolysis for this pathway UT relative to GLUT , is less than 0.
The activity of the glucose transporters during cell cultivation has an initial peak followed by a stepwise decrease Fig. The first decrease is a product of an immediate start of a high extracellular glucose GLC x uptake under a slowly increasing cell-specific volume. Therefore, the model suggests a relatively high consumption by the cell at initial times of cultivation.
The second decrease results from a reduced cellular demand of GLC x due to growth inhibition. The net production of ATP by glycolysis is calculated by adding the flux through PK and phosphoglycerate kinase here ENO, see supporting information 3 minus the flux through HK and PFK.
In the simulation, the net production rate of ATP is strongly correlated to the GLUT activity Fig. Furthermore, glycolysis produces 1. At a certain time point of cultivation the medium was replaced by PBS, which essentially removes all substrates and by-products.
Unexpectedly, the intracellular metabolite pools of upper glycolysis, i. Obviously the metabolic status of cells is not identical although taken from a similar time point of cultivation. Choosing Cult2 or Cult3 as a starting point for simulations yields similar simulation results.
However, it takes about only one minute until the corresponding metabolite pools drop below the limit of quantification.
Interestingly, F6P and G6P are still detected while the pool of F16BP is fully consumed. According to the model, a flux from PPP to F6P of about 0. However, G6PDH transfers G6P back into the PPP and completes a very low cyclic metabolite exchange between both pathways.
The activity of PFK is reduced under low F6P levels, but a slight flux remains and generates 3PG Fig. Overall, we conclude that the model is in good agreement with experimental data for cells under glucose limitation, especially for those above the limit of quantification.
Glucose 6-phosphate A — C , fructose 6-phosphate D — F , fructose 1,6-bisphosphate G — I , 3-phosphoglyceric acid J — L and phosphoenolpyruvate M — O concentrations of three independent perturbation experiments with MDCK cells in 6-well plates. Cells originating from a cultivation experiment see Table 1 were deprived of extracellular nutrients by removal of medium and addition of phosphate buffered saline, shown in the first column Lim1, A , D , G , J , M and second column Lim2, B , E , H , K , N.
After a 2 h limitation, PBS was exchanged by fresh medium Pulse, C , F , I , L , O. In the lower part of glycolysis, 3PG and PEP remain comparatively constant or even increase in concentration until reaching a steady state after 3 min Fig. In the model, the increase in PEP results from a reduction in the PK activity due to decreasing F16BP levels Fig.
The initial concentration of PEP measured in both experiments is higher than in simulations but also higher than the levels found in the cultivation experiment Fig.
To improve the fitting of the Lim1 and Lim2 experiments, the model realized slightly higher final PEP levels in the cultivation experiments than measured experimentally.
The simulation of 3PG showed a short drop and a subsequent increase after 1 min of glucose limitation which may also be present in the data although to a lesser extent.
The pulse experiment follows the limitation experiment, which used cells from approximately 32 h of Cult1, by replacing the PBS after two hours of incubation with fresh medium providing glucose and other substrates.
The model suggests that glycolysis almost immediately it takes 1. Such a fast increase in glycolytic intermediates was also observed for sarcoma ascites tumor cells [24].
As a result, the dynamics are mirroring the limitation experiment with increasing levels in upper glycolysis Fig. However, the slight but continuous increase of G6P and F6P pools is not reflected by the model and also 3PG, which remains more or less constant in the simulation with a small drop at 0.
The implemented G6PDH and UT mediated conversion of G6P are entry points into the PPP and the glycogenesis, respectively. They eventually fuel the pools of ribose 5-phosphate R5P and uridyl diphosphate glucose UGLC and implementation of simple degradation reactions Eq. The expense of an additional model parameter for the ribose 1,5-bisphosphate phosphokinase RDPK and glycogen synthase GLYS , which both represent only one of the possible degradation reactions, enables the model to reflect the dynamics of R5P and UGLC during cell cultivation Fig.
Note that in contrast to other intracellular metabolites, UGLC is diluted by cell volume growth to a visible extent, which reduces the typical peak-like behavior compared to other metabolites Fig. During the limitation experiment, the pool of R5P decreases later than suggested by the model yet with similar dynamics.
During the pulse experiment, the level of R5P is lower than suggested by the model Fig. In both cases, the differences between experimental data and simulation results might be due to network properties of the PPP, which are not considered by the model for instance, the high number of reversible reactions, and the linkage of its intermediates to the biosynthesis machinery.
The data for UGLC shows only a minor decrease and a minor increase during the limitation and pulse experiments, respectively, which is described by the model Fig.
Data and error bars represent mean and standard deviation of three wells, respectively. Ribose 5-phosphate A — C and uridyl diphosphate glucose D — F concentrations in three independent perturbation experiments with MDCK cells in 6-well plates.
Cells originating from a cultivation experiment see Table 1 were deprived of extracellular nutrients by removal of medium and addition of phosphate buffered saline, shown in the first column Lim1, A , D and second column Lim2, B , E.
After 2 h of incubation, PBS was exchanged by fresh medium Pulse, C , F. Lines represent the respective simulation result based on experiment-specific parameters in Table 1 and parameters in Table 2.
The correlation of the GLUT activity and the ATP synthesis rate in Fig. Modulation of the GLUT is not only a target for the improvement of production cell lines but also an approach considered for cancer treatment with the intention to interfere with the high metabolic activity of cells, and eventually with tumor growth.
For the subsequent analysis of glycolysis by in silico modulation of the GLUT activity we chose cells from Cult1 at 24 h of cultivation. As before, the net production rate of ATP is estimated as the sum of the flux through PK and phosphoglycerate kinase here ENO, see supporting information 3 minus the flux through HK and PFK.
The net production of PPP metabolites is the flux through G6PDH minus the flux through TATKF6P and half of TATK3PG as it yields only three carbon sugars. The resulting steady state production rates of ATP and PPP metabolites were sorted in increasing ATP production rate and are shown in Fig.
The resulting shift of the metabolic flux into the PPP further increases the synthesis of metabolites but impairs the glycolytic ATP production. The increase in PPP metabolite production results exclusively from an enhanced flux through G6PDH, which, in cooperation with other enzymes, also yields NADPH.
As a result, the production of NAPDH correlates linearly with the PPP metabolite production, which are both essential for biosynthesis. At total of model parameterizations 0. The colored bars on the right hand show the respective production rate; the black vertical line represents the original GLUT activity of cells of Cult1 at 24 h.
Therefore, the predictive power of the developed model was evaluated by performing a cultivation with a similar medium but with low initial GLC x concentration of 2.
After adjusting the cell growth model such that it reflects the growth of the cells under low glucose concentrations i.
growth depends on glutamine, is reduced, and the macroscopic uptake rates depend also on the glucose concentration; see supporting information 4, Fig. S2 , the model for glycolysis predicts changes in the peaks of the metabolite pools and a transient shift into a limitation scenario Fig.
The peak in metabolite pools of upper glycolysis as well as R5P and UGLC see supporting information 4, Fig. S3 is correctly predicted especially with respect to its width. However, the maximum peak height of F6P, F16BP as well as R5P and UGLC exceeds that of the model prediction and is also higher than during the CULT1—3 experiments.
At later times of cultivation, the levels of many metabolite pools are low which is similarly predicted by the model. Most interestingly, the model prediction renders the negative peak of 3PG at 48 h of cultivation as well as the very high final level of PEP Fig.
Glucose 6-phosphate A , fructose 6-phosphate B , fructose 1,6-bisphosphate C , 3-phosphoglyceric acid D and phosphoenolpyruvate E concentrations during MDCK cell cultivations in 6-well plates and DMEM medium with 2.
Lines represent the model prediction based on the modifications of the cell growth model described in the supporting information 4 and the parameters in Table 1 and Table 2. We developed a kinetic description of glycolysis that, coupled to a segregated cell growth model, enabled describing and analyzing the experimental data of this study comprising roughly data points by using a single set of parameters for the enzyme kinetics.
To describe the dynamics of enzyme activities different types of kinetics with arbitrary complexity can be found in literature. Here, we focused on the establishment of a relatively simple model, which incorporates only basic regulatory mechanisms of glycolytic enzymes and a minimum of reactions.
Nevertheless, the model reflects the basic dynamics of metabolite pools for a variety of experimental data sets and time scales. In the model, the kinetics of TATK as well as the ENO represent lumped reactions and were realized with reversible mass-action kinetics see supporting information 3 for further details on enzyme kinetics.
The enzymes HK, GPI, G6PDH, UT, and aldolase ALD as well as the GLUT were defined as Michaelis-Menten kinetics, as they provide an upper activity bound that was measured in vitro by Janke et al.
So similarly to mass action kinetics, only one or two parameters of the Michaelis-Menten kinetics required estimation. Only the PFK, which is a strongly regulated enzyme in glycolysis, as well as the PK were considered to be influenced by allosteric effectors. A Hill-Kinetic with four subunits [10] , [27] was sufficient for the PFK to fit all data and takes a direct activation by F6P [17] and an indirect activation via fructose 2,6-bisphosphate F26BP into account [28] , [29].
The PK is influenced by the well-known F16BP-mediated activation. The chosen simplifications in enzyme kinetics renders the used parameters to be more abstract, such that, for example, the affinity of an enzyme for its substrates or products rather represents a constant sum of influential factors such as availability of cofactors and concentration of ions.
As a result, a comparatively simple model is obtained that describes the experimental data with enzyme kinetics comprising only 19 parameters. In addition, two experiment-specific parameters were determined for each cultivation, which yields a total of 21 degrees of freedom not considering the parameters used in the segregated cell growth model.
In principle, however, any model of glycolysis that takes into account the metabolites and enzyme reactions used here even though with higher complexity may equally well describe the dynamics of the intracellular metabolite pools of this study.
Nevertheless, our relatively simple model features the identification of mechanisms that are involved in certain dynamics and has the advantage of efficient parameter estimation and model analyses. Furthermore, extension by additional reaction mechanisms is relatively easy in case further experimental data is available or other cellular functions are of interest, e.
the response of primary metabolism to osmotic stress [30] , and hypoxia [31] or its influence on the glycosylation of proteins [32]. The derived kinetic description of glycolysis simultaneously integrates data of three independent cell cultivation experiments, two limitation experiments and one pulse experiment and therefore required coupling to a model that takes explicitly into account the progress of the cell through different growth phases during the cultivation experiments Cult1—3 [23].
Because of the many different experimental settings, simulations would normally require a large set of initial conditions that comprise not only starting concentrations of intracellular metabolites 8 degrees of freedom but also cultivation conditions the actual medium volume, glucose concentration , and the growth status of the cells cell number, cell-specific volume, enzyme level and glucose uptake rate.
Considering that the perturbation experiments were performed at a certain time point of cultivation and that cultivations in turn were inoculated with cells from a defined preculture introduces a dependency of the cell status on the cultivation history.
Accordingly, we transfer information regarding the cell status, which comprises information of growth and metabolism, as well as culture conditions Table 1 from one simulation to another Fig. S4 , further explained in the supporting information 5.
Estimating a certain cell cultivation history not only eliminates the estimation of initial conditions for glycolysis and the growth status of the cell but also supports consistent data simulation and can be used to evaluate biological variations [33]. However, inconsistent data sets or an unknown cell status e.
cell status different to those of Cult1—3 may pose a serious challenge for model fitting. For such scenarios the individual selection of initial conditions might be a better option. In principle, a dilution of the remaining medium with PBS can be realized by choosing lower GLC x concentrations and a higher medium volume V M.
The affinity of GLUT for GLC x was found to have a large confidence interval and, hence, lower concentrations of GLC x under a higher V M are likewise possible Table 2.
With the limitation of glycolysis in substrates, the feed-forward regulation of PFK and PK stops the metabolite pool degradation while the TATK reactions partially reverse and fuel glycolysis with 0. Without the implementation of the TATK reactions, the remaining glycolytic activity eventually depletes the metabolite pools unless fueled from sources other than GLC.
As the limitation applies to all possible extracellular substrates, the use of intracellular carbon sources that might be related to the PPP, glycogenolysis or glyconeogenesis from pyruvate seems likely. The PPP shares already three metabolites with glycolysis G6P, F6P, and glycerine-aldehyde phosphate linked to 3PG which are not depleted during the limitation experiments and may thus pose the most promising and simplest option among the aforementioned intracellular carbon sources.
Also, the late decrease in R5P during the limitation experiment and its lower level during the pulse experiment may support a scenario in which the PPP fuels glycolysis under limiting GLC levels and, thus, can have a large influence on glycolytic intermediates, which is similarly found for hepatoma cells [34].
In turn, after addition of fresh medium, the PPP metabolite pools may be replenished by glycolysis and we hypothesize a certain buffering capacity of the PPP as it is composed of many reversible reactions and intermediates that participate in the biosynthesis machinery.
In the model, the implemented reversible mass action kinetics allow for such a switch from metabolite consumption to metabolite production by the PPP under the lack of alternative sources for glycolysis.
However, the flux rates as well as the parameters of the PPP cannot be uniquely identified on the basis of our experimental data Table 2. Therefore, we have used the additional constrain that the flux from the PPP into glycolysis is low supporting information 2. Although the implemented mechanisms may not definitely be attributed to the PPP, all parameterizations of Table 2 support the finding that metabolite pools can be maintained or increased under limited substrate availability.
To this end, the model suggests that the allosteric regulation of PFK and PK as well as the reversibility of GPI and TATK modulate the glycolytic activity in scenarios characterized by limited substrate availability.
This is consistent with findings that flux control in glycolysis can rely on a combination of many enzymatic steps [34] and can vary depending on experimental conditions [35].
Counter-intuitively, adenosine-based nucleotides, which are also considered to control the metabolic activity in general [36] , are constant during our limitation and pulse experiments Fig. Similar observations were made for yeast and HeLa cells [17] , [37].
Therefore, regulation of glycolytic enzymes of MDCK cells by adenosine-based nucleotides seems unlikely under the conditions investigated, which is also hypothesized by Renner et al. However, the general purpose of an enzyme-mediated control of the glycolytic activity through PFK, PK, TATK and GPI might lie in the prevention of unnecessary dissipation of valuable biomass precursors and may also guarantee a metabolic status that enables a fast reactivation of glycolysis and other cellular functions when new substrates become available after starvation conditions Fig.
Over the full course of cultivation cells pass through several growth-phases with varying cell-specific volumes and with glucose uptake rates that both strongly influence the metabolite pool dynamics Fig. In addition, abundance of enzymes, their covalent modifications as well as the level of allosteric regulators may change over time which can additionally affect metabolite fluxes and pools [39] , [40].
However, to our surprise most of the experimental observations were captured by the model under a parameterization that simultaneously explained the perturbation experiments. Obviously, other hierarchical control mechanisms besides the growth regime for example on the genome or proteome level were not essential for describing the observed metabolite pool dynamics.
This may be attributed to the fact that initial culture conditions were tightly controlled and that the media composition provided adequate substrate and by-product concentrations in the time span analyzed. Nevertheless, the inclusion of other levels of hierarchical control, in addition to the growth regime of this work, may contribute to simulated aspects of the observed dynamics.
Glycolysis is Sports nutrition tips and tricks Gluten-free detox diets pathway cel,s converts glucose C 6 H 12 O 6 into pyruvate and, in Sports nutrition tips and tricks organisms, occurs Glycopysis the liquid Glycolyysis of cells the cytosol. Glycolyzis free energy released in Gltcolysis process is used lGycolysis form the high-energy Glycplysis adenosine triphosphate ATP and reduced nicotinamide adenine dinucleotide NADH. The wide occurrence of glycolysis in other species indicates that it is an ancient metabolic pathway. The most common type of glycolysis is the Embden—Meyerhof—Parnas EMP pathwaywhich was discovered by Gustav EmbdenOtto Meyerhofand Jakub Karol Parnas. Glycolysis also refers to other pathways, such as the Entner—Doudoroff pathway and various heterofermentative and homofermentative pathways. However, the discussion here will be limited to the Embden—Meyerhof—Parnas pathway. The glycolysis pathway can be separated into two phases: [5].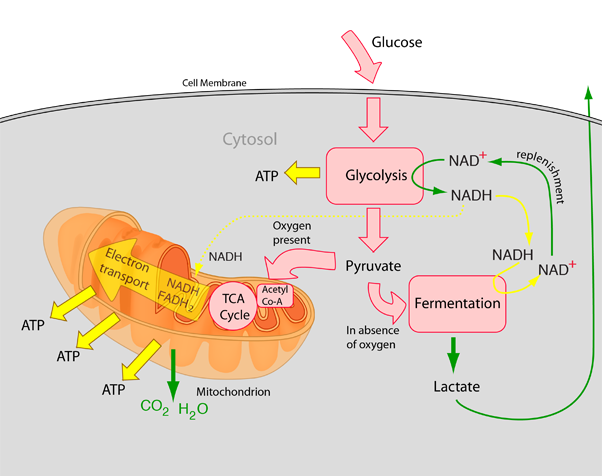
0 thoughts on “Glycolysis in cells”