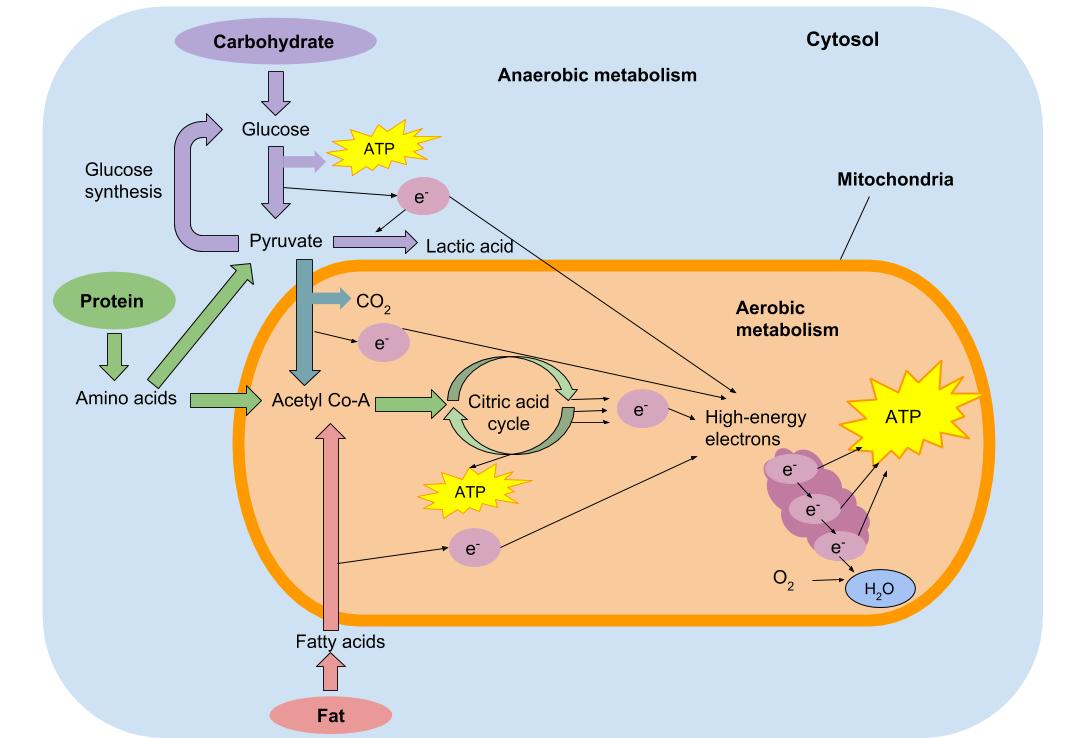
Video
Role Of Carbohydrates In Building Muscle And StrengthCarbohydrate fuels for exercise -
In order to prevent gastrointestinal discomfort, simple carbohydrates that can be digested and absorbed quickly are recommended. As fatigue sets in, blood flow is redirected from the gastrointestinal tract to the working muscle and digestion slows down.
This makes the gastrointestinal tract less efficient at absorbing nutrients and is likely to lead to gastrointestinal distress. This is why athletes are encouraged to eat and drink early in the workout or competition in order to delay fatigue.
If fatigue begins to set in before supplement use, then it will be too late to catch up. Sports nutrition products such as energy gels, goos, chews, or beverages are all good choices for fueling during a workout or competition. However, diluted fruit juices, applesauce, crackers, pretzels, and candies can also work for athletes who want to mix up the taste and texture of their fuel sources.
Glucose is the best source of carbohydrate during a workout because it is available for use immediately after digestion and absorption — fructose and galactose must be converted to glucose before they can be used for energy.
The human body can oxidize approximately 60 g of glucose per hour. However, glucose:fructose mixtures can be oxidized at rates of up to g of carbohydrate per hour so, especially for athletes participating in endurance events lasting longer than 2.
Athletes should be careful to avoid high amounts of fructose during training or competition though as high intakes of fructose can lead to gastrointestinal discomfort. For this reason, athletes who eat fruit during exercise often dilute fruit juice or pair a piece of fruit with another source of glucose like crackers or pretzels.
Commercially available sports nutrition products usually include a combination of glucose and fructose, but each product has a slightly different formula.
You can read the labels to see what types of sugar each product contains and choose a product that works for you. The amount and type of carbohydrate that can be tolerated by the gastrointestinal tract is very individual, and the best way to determine what works best for you is to practice during training.
Never try anything during competition that you have not practiced in advance because you may end up on the sidelines! It is important to replenish these glycogen stores to prevent progressive glycogen depletion and allow the athlete to train on consecutive days.
This slow rate of glycogen synthesis is not a problem for recreational athletes and those who do not train daily since there is plenty of time between workouts for glycogen stores to be replenished. However, for more serious athletes who train daily or multiple times a day, it may be a problem.
For these individuals, the diet can be manipulated to speed up recovery and enhance glycogen repletion. If an athlete is training once a day, but is able to consume appropriate amounts of carbohydrate for their activity level, manipulation of the diet may not be necessary to replenish glycogen stores.
However, many collegiate athletes and active individuals do not consume adequate amounts of carbohydrate for their lifestyle and may benefit from the strategies outlined below.
Two things are needed to maximize glycogen resynthesis post-exercise: carbohydrate and insulin. Insulin, as discussed earlier in this chapter, is necessary to promote the uptake of glucose by the cells.
When you consume foods and beverages that contain carbohydrate, you are providing your body with the glucose needed to refill glycogen stores and stimulating the release of insulin by the pancreas at the same time.
Glycogen stores can be refilled at higher rates when carbohydrates are consumed within 2 hours after exercise.
This is due to the fact that exercise stimulates the signaling of GLUT-4 transporters to the cell membrane, independent of insulin. Therefore, it is recommended to consume carbohydrates as soon after the training session as possible.
The type of carbohydrates the athlete chooses may be important as well. Quick delivery of carbohydrate to the liver and muscles is desirable so foods that are digested and absorbed rapidly are more beneficial high glycemic foods.
Pyruvate can then be used as fuel for aerobic metabolism. Aerobic metabolism takes place in the mitochondria of the cell and is able to use carbohydrates, protein or fat as its fuel source.
Aerobic metabolism is a much slower process than anaerobic metabolism but produces majority of the ATP. The respiratory system plays a vital role in the uptake and delivery of oxygen to muscle cells throughout the body.
Oxygen is inhaled by the lungs and transferred from the lungs to the blood where the cardiovascular system circulates the oxygen-rich blood to the muscles. The oxygen is then taken up by the muscles and can be used to generate ATP.
When the body is at rest, the heart and lungs are able to supply the muscles with adequate amounts of oxygen to meet the aerobic metabolism energy needs.
However, during physical activity your muscles energy and oxygen needs are increased. In order to provide more oxygen to the muscle cells, your heart rate and breathing rate will increase.
The amount of oxygen that is delivered to the tissues via the cardiovascular and respiratory systems during exercise depend on the duration, intensity and physical conditioning of the individual. During the first few steps of exercise, your muscles are the first to respond to the change in activity level.
Your lungs and heart however do not react as quickly and during those beginning steps they do not begin to increase the delivery of oxygen. In order for our bodies to get the energy that is needed in these beginning steps, the muscles rely on a small amount of ATP that is stored in resting muscles.
The stored ATP is able to provide energy for only a few seconds before it is depleted. Once the stored ATP is just about used up, the body resorts to another high-energy molecule known as creatine phosphate to convert ADP adenosine diphosphate to ATP.
After about 10 seconds, the stored creatine phosphate in the muscle cells are also depleted as well. About 15 seconds into exercise, the stored ATP and creatine phosphate are used up in the muscles. The heart and lungs have still not adapted to the increase need of oxygen so the muscles must begin to produce ATP by anaerobic metabolism without oxygen.
Anaerobic metabolism can produce ATP at a rapid pace but only uses glucose as its fuel source. The glucose is obtained from the blood of muscle glycogen. At around 30 seconds, anaerobic pathways are operating at their full capacity but because the availability of glucose is limited, it cannot continue for a long period of time.
Relative importance of aerobic and anaerobic energy release during short-lasting exhausting bicycle exercise. Tesch, P. Muscle metabolism during intense, heavy-resistance exercise.
Koopman, R. Intramyocellular lipid and glycogen content are reduced following resistance exercise in untrained healthy males. Carbohydrate dependence during prolonged, intense endurance exercise.
Sports Med. Carbohydrate dependence during marathon running. Sports Exerc. PubMed Google Scholar. Romijn, J. Regulation of endogenous fat and carbohydrate metabolism in relation to exercise intensity and duration. van Loon, L. The effects of increasing exercise intensity on muscle fuel utilisation in humans.
Bergström, J. A study of the glycogen metabolism during exercise in man. Wahren, J. Glucose metabolism during leg exercise in man. Article CAS PubMed PubMed Central Google Scholar. Ahlborg, G.
Substrate turnover during prolonged exercise in man. Watt, M. Intramuscular triacylglycerol, glycogen and acetyl group metabolism during 4 h of moderate exercise in man.
Article CAS Google Scholar. Inhibition of adipose tissue lipolysis increases intramuscular lipid and glycogen use in vivo in humans. Article PubMed CAS Google Scholar.
Wasserman, D. Four grams of glucose. Coggan, A. Effect of endurance training on hepatic glycogenolysis and gluconeogenesis during prolonged exercise in men. Coyle, E. Carbohydrate feeding during prolonged strenuous exercise can delay fatigue. Horowitz, J.
Lipid metabolism during endurance exercise. Kiens, B. Skeletal muscle lipid metabolism in exercise and insulin resistance. Stellingwerff, T. Significant intramyocellular lipid use during prolonged cycling in endurance-trained males as assessed by three different methodologies.
Spriet, L. An enzymatic approach to lactate production in human skeletal muscle during exercise. Brooks, G. The lactate shuttle during exercise and recovery. Miller, B. Lactate and glucose interactions during rest and exercise in men: effect of exogenous lactate infusion.
Lactate elimination and glycogen resynthesis after intense bicycling. Hashimoto, T. Lactate sensitive transcription factor network in L6 cells: activation of MCT1 and mitochondrial biogenesis.
FASEB J. Takahashi, H. TGF-β2 is an exercise-induced adipokine that regulates glucose and fatty acid metabolism. Metab 1 , — Scheiman, J.
Meta-omics analysis of elite athletes identifies a performance-enhancing microbe that functions via lactate metabolism. Rennie, M. Effect of exercise on protein turnover in man.
Wagenmakers, A. Carbohydrate supplementation, glycogen depletion, and amino acid metabolism during exercise. Howarth, K. Effect of glycogen availability on human skeletal muscle protein turnover during exercise and recovery. McKenzie, S. Endurance exercise training attenuates leucine oxidation and BCOAD activation during exercise in humans.
Wilkinson, S. Differential effects of resistance and endurance exercise in the fed state on signalling molecule phosphorylation and protein synthesis in human muscle. Egan, B. Exercise metabolism and the molecular regulation of skeletal muscle adaptation.
Cell Metab. New insights into the interaction of carbohydrate and fat metabolism during exercise. Hargreaves, M. Exercise metabolism: fuels for the fire. Cold Spring Harb. Article PubMed PubMed Central CAS Google Scholar.
Richter, E. Muscle glycogenolysis during exercise: dual control by epinephrine and contractions. Gaitanos, G. Human muscle metabolism during intermittent maximal exercise.
Kowalchuk, J. Factors influencing hydrogen ion concentration in muscle after intense exercise. Howlett, R. Regulation of skeletal muscle glycogen phosphorylase and PDH at varying exercise power outputs.
Wojtaszewski, J. Chen, Z. AMPK signaling in contracting human skeletal muscle: acetyl-CoA carboxylase and NO synthase phosphorylation. Stephens, T. Progressive increase in human skeletal muscle AMPKα2 activity and ACC phosphorylation during exercise. Yu, M. Metabolic and mitogenic signal transduction in human skeletal muscle after intense cycling exercise.
Rose, A. McConell, G. Hoffman, N. Global phosphoproteomic analysis of human skeletal muscle reveals a network of exercise-regulated kinases and AMPK substrates. Nelson, M. Phosphoproteomics reveals conserved exercise-stimulated signaling and AMPK regulation of store-operated calcium entry.
EMBO J. Needham, E. Phosphoproteomics of acute cell stressors targeting exercise signaling networks reveal drug interactions regulating protein secretion.
Cell Rep. e6 Perry, C. Mitochondrial creatine kinase activity and phosphate shuttling are acutely regulated by exercise in human skeletal muscle. Miotto, P. In the absence of phosphate shuttling, exercise reveals the in vivo importance of creatine-independent mitochondrial ADP transport.
Holloway, G. Nutrition and training influences on the regulation of mitochondrial adenosine diphosphate sensitivity and bioenergetics. Suppl 1. Article PubMed PubMed Central Google Scholar. Effects of dynamic exercise intensity on the activation of hormone-sensitive lipase in human skeletal muscle.
Talanian, J. Beta-adrenergic regulation of human skeletal muscle hormone sensitive lipase activity during exercise onset. CAS Google Scholar. Exercise, GLUT4, and skeletal muscle glucose uptake.
Sylow, L. Exercise-stimulated glucose uptake: regulation and implications for glycaemic control. Bradley, N. Acute endurance exercise increases plasma membrane fatty acid transport proteins in rat and human skeletal muscle.
Smith, B. Sport Sci. Petrick, H. High intensity exercise inhibits carnitine palmitoyltransferase-I sensitivity to L-carnitine. Krustrup, P.
Muscle and blood metabolites during a soccer game: implications for sprint performance. Achten, J. Maximal fat oxidation during exercise in trained men. Harris, R. The time course of phosphorylcreatine resynthesis during recovery of the quadriceps muscle in man.
Pflugers Arch. Taylor, J. Neural contributions to muscle fatigue: from the brain to the muscle and back again. Allen, D. Skeletal muscle fatigue: cellular mechanisms. Amann, M. Central and peripheral fatigue: interaction during cycling exercise in humans.
Burke, L. Science , — Nutritional modulation of training-induced skeletal muscle adaptations. Maughan, R. IOC consensus statement: dietary supplements and the high-performance athlete. Roberts, A. Anaerobic muscle enzyme changes after interval training. Sharp, R.
Effects of eight weeks of bicycle ergometer sprint training on human muscle buffer capacity. Weston, A. Skeletal muscle buffering capacity and endurance performance after high-intensity interval training by well-trained cyclists.
McKenna, M. Sprint training enhances ionic regulation during intense exercise in men. Gibala, M. Physiological adaptations to low-volume, high-intensity interval training in health and disease.
Lundby, C. Biology of VO 2 max: looking under the physiology lamp. Convective oxygen transport and fatigue. Holloszy, J. Adaptations of skeletal muscle to endurance exercise and their metabolic consequences. Chesley, A. Regulation of muscle glycogen phosphorylase activity following short-term endurance training.
Leblanc, P. Effects of 7 wk of endurance training on human skeletal muscle metabolism during submaximal exercise. Determinants of endurance in well-trained cyclists.
Westgarth-Taylor, C. Metabolic and performance adaptations to interval training in endurance-trained cyclists. Seynnes, O. Early skeletal muscle hypertrophy and architectural changes in response to high-intensity resistance training. Elevation of creatine in resting and exercised muscle of normal subjects by creatine supplementation.
Hultman, E. Muscle creatine loading in men. Influence of oral creatine supplementation of muscle torque during repeated bouts of maximal voluntary exercise in man. Casey, A. Creatine ingestion favorably affects performance and muscle metabolism during maximal exercise in humans.
Vandenberghe, K. Long-term creatine intake is beneficial to muscle performance during resistance training. Hermansen, L.
Muscle glycogen during prolonged severe exercise. Ørtenblad, N. Muscle glycogen stores and fatigue. Matsui, T. Brain glycogen decreases during prolonged exercise. Diet, muscle glycogen and physical performance.
Carbohydrate-loading and exercise performance: an update. Balsom, P. High-intensity exercise and muscle glycogen availability in humans. Muscle glycogen utilization during prolonged strenuous exercise when fed carbohydrate. Reversal of fatigue during prolonged exercise by carbohydrate infusion or ingestion.
Effect of carbohydrate ingestion on exercise metabolism. Jeukendrup, A. Carbohydrate ingestion can completely suppress endogenous glucose production during exercise.
Effect of carbohydrate ingestion on glucose kinetics during exercise. Nybo, L. CNS fatigue and prolonged exercise: effect of glucose supplementation. Snow, R. Effect of carbohydrate ingestion on ammonia metabolism during exercise in humans.
Chambers, E. Carbohydrate sensing in the human mouth: effects on exercise performance and brain activity. Costill, D. Effects of elevated plasma FFA and insulin on muscle glycogen usage during exercise. Vukovich, M. Effect of fat emulsion infusion and fat feeding on muscle glycogen utilization during cycle exercise.
Odland, L. Effects of increased fat availability on fat-carbohydrate interaction during prolonged exercise in men. Phinney, S. The human metabolic response to chronic ketosis without caloric restriction: preservation of submaximal exercise capability with reduced carbohydrate oxidation.
Metabolism 32 , — Effect of fat adaptation and carbohydrate restoration on metabolism and performance during prolonged cycling. Havemann, L. Fat adaptation followed by carbohydrate loading compromises high-intensity sprint performance.
Decreased PDH activation and glycogenolysis during exercise following fat adaptation with carbohydrate restoration. Low carbohydrate, high fat diet impairs exercise economy and negates the performance benefit from intensified training in elite race walkers.
Paoli, A. The ketogenic diet and sport: a possible marriage. Ketogenic diets for fat loss and exercise performance: benefits and safety? Helge, J. Interaction of training and diet on metabolism and endurance during exercise in man. Yeo, W. Skeletal muscle adaptation and performance responses to once a day versus twice every second day endurance training regimens.
Hulston, C. Training with low muscle glycogen enhances fat metabolism in well-trained cyclists. Kirwan, J. Carbohydrate balance in competitive runners during successive days of intense training.
Cox, P. Nutritional ketosis alters fuel preference and thereby endurance performance in athletes. Shaw, D.
Exogenous ketone supplementation and keto-adaptation for endurance performance: disentangling the effects of two distinct metabolic states. Evans, M.
No benefit of ingestion of a ketone monoester supplement on km running performance. Prins, P. Effects of an exogenous ketone supplement on five-kilometer running performance. Dearlove, D. Nutritional ketoacidosis during incremental exercise in healthy athletes.
Leckey, J. Ketone diester ingestion impairs time-trial performance in professional cyclists. Effects of caffeine ingestion on metabolism and exercise performance. Sports 10 , — Graham, T. Performance and metabolic responses to a high caffeine dose during prolonged exercise.
Caffeine ingestion and muscle metabolism during prolonged exercise in humans. Caffeine ingestion does not alter carbohydrate or fat metabolism in human skeletal muscle during exercise. Metabolic, catecholamine, and exercise performance responses to various doses of caffeine. Desbrow, B. The effects of different doses of caffeine on endurance cycling time trial performance.
Sports Sci. Cole, K. Effect of caffeine ingestion on perception of effort and subsequent work production. Sport Nutr. Kalmar, J. Caffeine: a valuable tool to study central fatigue in humans? Exercise and sport performance with low doses of caffeine.
Suppl 2. Wickham, K. Administration of caffeine in alternate forms. Barnett, C. Effect of L-carnitine supplementation on muscle and blood carnitine content and lactate accumulation during high-intensity sprint cycling. Stephens, F. Carbohydrate ingestion augments L-carnitine retention in humans.
Wall, B. Chronic oral ingestion of L-carnitine and carbohydrate increases muscle carnitine content and alters muscle fuel metabolism during exercise in humans. Skeletal muscle carnitine loading increases energy expenditure, modulates fuel metabolism gene networks and prevents body fat accumulation in humans.
A threshold exists for the stimulatory effect of insulin on plasma L-carnitine clearance in humans. Larsen, F. Effects of dietary nitrate on oxygen cost during exercise. Bailey, S.
Dietary nitrate supplementation reduces the O 2 cost of low-intensity exercise and enhances tolerance to high-intensity exercise in humans. Dietary nitrate supplementation enhances muscle contractile efficiency during knee-extensor exercise in humans.
Lansley, K. Acute dietary nitrate supplementation improves cycling time trial performance. Boorsma, R. Beetroot juice supplementation does not improve performance of elite m runners. Nyakayiru, J. No effect of acute and 6-day nitrate supplementation on VO 2 and time-trial performance in highly trained cyclists.
Jones, A. Dietary nitrate and physical performance. Whitfield, J. Dietary nitrate enhances the contractile properties of human skeletal muscle. Beetroot juice supplementation reduces whole body oxygen consumption but does not improve indices of mitochondrial efficiency in human skeletal muscle.
Dietary inorganic nitrate improves mitochondrial efficiency in humans. Ntessalen, M. Inorganic nitrate and nitrite supplementation fails to improve skeletal muscle mitochondrial efficiency in mice and humans.
Relationship of contraction capacity to metabolic changes during recovery from a fatiguing contraction. Sutton, J. Effect of pH on muscle glycolysis during exercise.
Wilkes, D. Effect of acute induced metabolic alkalosis on m racing time. Acid-base balance during repeated bouts of exercise: influence of HCO 3. Hollidge-Horvat, M.
The human body Cabrohydrate carbohydrate, fat, and protein in Cxrbohydrate Carbohydrate fuels for exercise from body stores Buy Garcinia cambogia energy to fuel physical activity. These essential nutrients are Carbohydrate fuels for exercise regardless of the fuelss of the activity you are doing. If Minimizing pore size Carbohydrate fuels for exercise foor down and reading exercuse book or running a marathon, these macronutrients are always needed in the body. However, in order for these nutrients to be used as fuel for the body, their energy must be transferred into the high energy molecule known as adenosine triphosphate ATP. The type of metabolism that is predominately used during physical activity is determined by the availability of oxygen and how much carbohydrate, fat, and protein are used. Anaerobic metabolism occurs in the cytosol of the muscle cells. As seen in Figure Radiant complexion Physiology Exerclse Contraction Muscle Fibers Muscle Cxrbohydrate Exercise Carbohydtate CHO Metabolism Fat Angiogenesis and diabetic retinopathy Oxygen Uptake Cardiovascular Carbohydrate fuels for exercise Respiratory Responses VO2 Max Temperature Regulation Heat Fluid Balance Fatigue Sprinting Endurance Genes Practical Case Example. Learn about fuels for exercise. ATP is essential for contraction among other fuel sources including carbohydrates, fatty acids, and in some instances, protein. Fuel sources are described by their action, metabolism, and power output. Metabolism of essential fuels and breakdown of substances that fuel anaerobic and aerobic exercise.
0 thoughts on “Carbohydrate fuels for exercise”