BCAA and muscle protein breakdown -
Their major marketing appeal derives from allegations that branched-chain amino acids intake combined with resistance physical exercise stimulates muscle protein synthesis.
Evidence supporting the efficacy of branched-chain amino acids alone for muscle hypertrophy in humans is somewhat equivocal. This brief review describes physiological and biochemical mechanisms underpinning the effects of complete protein source and branched-chain amino acid intake on skeletal muscle growth in the postabsorptive and post-exercise state.
Evidence in favor of or against potential anabolic effects of isolated branched-chain amino acid intake on muscle protein synthesis in humans is also examined. A comercialização dos suplementos de aminoácidos de cadeia ramificada valina, leucina e isoleucina possui muita popularidade entre aqueles com alegação ergogênica.
O principal marketing está na afirmação de que o consumo isolado de aminoácidos de cadeia ramificada associado ao exercício físico resistido estimula a síntese de proteína muscular.
As evidências da eficácia da ingestão isolada de aminoácidos de cadeia ramificada para a hipertrofia muscular em humanos parecem equivocadas. Nesta breve revisão, apresentamos a compreensão fisiológica e bioquímica de como a ingestão de uma fonte completa de proteína e de aminoácidos de cadeia ramificada afeta o crescimento do músculo esquelético no estado pós-absortivo e pós-exercício.
Mostramos também as evidências que suportam ou não a afirmação dos potenciais efeitos anabólicos na síntese de proteína muscular dos aminoácidos de cadeia ramificada quando consumidos isoladamente em humanos.
These aids are thought to boost energy production and use, support recovery from exercise, improve body composition and increase resistance to peripheral and central fatigue.
Beck KL, Thomson JS, Swift RJ, von Hurst PR. Role of nutrition in performance enhancement and postexercise recovery. Open Access J Sports Med. According to the International Society of Sports Nutrition ISSN , ergogenic supplement claims must be backed up by plausible data and supported by solid evidence of efficacy; these supplements must also be legal and safe.
Kerksick CM, Wilborn CD, Roberts MD, Smith-Ryan A, Kleiner SM, Jäger R, et al. J Int Soc Sports Nutr. Branched-chain amino acids BCAA enjoy great popularity among allegedly ergogenic supplements.
The marketing appeal of these products derives from claims that isolated BCAA intake combined with resistance physical exercise stimulates muscle protein synthesis. This long-standing over 35 years claim is based on cellular and animal model studies reporting enhanced anabolic intracellular signaling in response to BCAA intake.
Wolfe RR. Branched-chain amino acids and muscle protein synthesis in humans: myth or reality? However, evidence supporting the efficacy of isolated BCAA intake for muscle hypertrophy in humans is equivocal. This study set out to review muscle protein synthesis in the postabsorptive state and after resistance physical exercise from a basic biochemistry perspective, and to examine existing evidence in favor of or against claims of potential anabolic effects of isolated BCAA intake on muscle protein synthesis in humans.
A secondary search was conducted based on the references listed in selected articles. Proteins consist of 20 amino acids AA linked by peptide bonds and arranged in different combinations and amounts.
Nine out of these 20 AA are thought to be essential EAA - essential amino acids , i. The remaining 11 EAA are classified as nonessential NEAA, nonessential amino acids , as they can be synthesized in the body. Moran LA, Horton HR, Scrimgeour KG, Perry MD.
Amino acids and the primary structure of proteins. In: Moran LA, Horton HR, Scrimgeour KG, Perry MD, editores.
Biochemistry São Paulo: Pearson Education of Brazil; Nelson DL, Cox MM. Amino acids, peptides and proteins. In: Nelson DL, Cox MM, editores. Principles of Biochemistry 6 ed. Porto Alegre: Artmed Publisher; In healthy individuals with normal mobility, dynamic balance between protein degradation and synthesis orchestrates skeletal muscle protein maintenance.
In the postabsortive i. In the postprandial state, synthesis exceeds degradation, since intake of some nutrients, such as proteins and carbohydrates, stimulates muscle protein synthesis and insulin release, suppressing degradation. Rennie MJ, Wackerhage H, Spangenburg EE, Booth FW.
Control of the size of the human muscle mass. Annu Rev Physiol. Therefore, muscle hypertrophy requires a positive net protein balance i. Physical exercise and nutrient availability are the major drivers of muscle protein synthesis in adult individuals. Atherton PJ, Smith K. Muscle protein synthesis in response to nutrition and exercise.
J Physiol. McGlory C, Van Vliet S, Stokes T, Mittendorfer B, Phillips SM. The impact of exercise and nutrition on the regulation of skeletal muscle mass. The anabolic effects of nutrients are boosted primarily by transfer and incorporation of AA obtained through the diet into skeletal muscle proteins.
These effects are particularly associated with EAA. Smith K, Barua JM, Watt PW, Scrimgeour CM, Rennie MJ. Flooding with L-[C]leucine stimulates human muscle protein incorporation of continuously infused L-[C] valine. Am J Physiol.
The full range of EAA and the 11 NEAA must be present in proper amounts for muscle protein synthesis. Therefore, muscle protein synthesis is limited by lack or low availability of any of the EAA, whereas lack of NEAA can be offset by increased de novo synthesis.
In the postprandial state, within approximately 30 to 45 minutes of consumption of a protein-rich meal average time required for digestion, absorption and transport of AA to the systemic circulation , EAA availability increases and muscle protein synthesis rates exceed muscle protein degradation rates, inducing an anabolic state that peaks between 1.
Atherton PJ, Etheridge T, Watt PW, Wilkinson D, Selby A, Rankin D, et al. Muscle full effect after oral protein: time-dependent concordance and discordance between human muscle protein synthesis and mTORC1 signaling. Am J Clin Nutr.
Aminoacidemia-induced muscle protein synthesis is transient. In the postabsortive state i. In these circumstances, plasma EAA level maintenance and hence protein turnover relies on protein breakdown in skeletal muscles, the major body protein reservoir. Cahill GF Jr, Aoki TT.
Starvation and body nitrogen. Trans Am Clin Climatol Assoc. The impact of factors such as protein amount and quality, protein intake distribution throughout day and physical exercise on the balance between protein degradation and synthesis must be emphasized. Biolo G, Gastaldelli A, Zhang XJ, Wolfe RR.
Protein synthesis and breakdown in skin and muscle: a leg model of amino acid kinetics. Hence, muscle protein degradation always exceeds muscle protein synthesis in the postabsorptive state due to muscle protein catabolism and catabolic conditions determined by lack of dietary EAA intake.
This is thought to be partly due to the AA leucine, as leucine alone is able to induce a muscle protein synthesis response via activation of the mechanistic target of rapamycin complex 1 mTORC1 , a vital cell growth regulator.
Crozier SJ, Kimball SR, Emmert SW, Anthony JC, Jefferson LS. Oral leucine administration stimulates protein synthesis in rat skeletal muscle.
J Nutr. Louard et al. Louard RJ, Barrett EJ, Gelfand RA. Effect of infused branched-chain amino acids on muscle and whole-body amino acid metabolism in man. Clin Sci Lond.
Overnight branched-chain amino acid infusion causes sustained suppression of muscle proteolysis. tested this hypothesis in humans submitted to overnight fasting. In their study, the effects of intravenous BCAA infusion for 3 and 16 hours on muscle protein synthesis and degradation were investigated.
Both infusion protocols increased plasma BCAA levels, whereas plasma levels of other EAA decreased. The fact that muscle protein degradation was mitigated by isolated intake of three out of 11 EAA, but remained higher than muscle protein synthesis, suggested the catabolic state prevailed in order to release other EAA required for synthesis.
It seems therefore plausible to assume BCAA intake alone cannot create an anabolic state leading to muscle protein synthesis in excess of degradation, at least in theory.
Another important question is whether anabolic pathway activation and increased muscle protein synthesis are separate events. Rising insulin levels are a potent anabolic signaling pathway activator, but are not associated with enhanced muscle protein synthesis in the absence of EAA.
Greenhaff PL, Karagounis LG, Peirce N, Simpson EJ, Hazell M, Layfield R, et al. Disassociation between the effects of amino acids and insulin on signaling, ubiquitin ligases, and protein turnover in human muscle. Am J Physiol Endocrinol Metab. In contrast, intake of small amounts 3g of EAA stimulates synthesis regardless of anabolic signaling pathway activation.
Bukhari SS, Phillips BE, Wilkinson DJ, Limb MC, Rankin D, Mitchell WK, et al. The design, purpose, and potential risks associated with the study were explained to participants before obtaining written informed consent. The National Research Ethics Service board Warwickshire, Birmingham, UK approved all procedures and all were in accordance with the Helsinki Declaration of as revised in This trial is registered as a clinical trial registration number: ISRCTN Participants reported to the laboratory on five separate occasions, including two experimental trials that were separated by approximately three weeks.
During the initial visit, body composition was assessed using dual-energy x-ray absorptiometry DEXA; Hologic Discovery W, Hologic Inc. Approximately one week later, participants returned to the laboratory to confirm their 1RM for each leg. Three days later, participants performed their first blinded trial in which they consumed either a BCAA containing drink BCAA; GlaxoSmithKline, Brentford, UK or placebo PLA drink GlaxoSmithKline, Brentford, UK; Table 1.
BCAA contained 5. Participants performed a unilateral bout of resistance exercise prior to consuming the test drink in each trial. Myofibrillar-MPS was determined by measuring the incorporation of L-[ring- 13 C 6 ] phenylalanine into myofibrillar protein during a primed continuous infusion.
The complete testing procedure was repeated on the contralateral leg approximately three weeks after the first trial. Trial and exercised leg order were counter-balanced and randomized. This exercise load was then inserted into an equation as previously reported to estimate 1RM Mayhew et al.
Three days prior to both infusion trials, participants returned to the laboratory to confirm their 1RM on both exercises Kraemer and Fry, on the leg that was to be exercised in the forthcoming trial.
Participants completed the maximum number of repetitions possible. A rest period of 3 min was given between each load. During the initial visit, body composition total and segmental was assessed using DEXA. Prior the experimental trial, participants completed a questionnaire of food preferences and a 3-day diet diary that represented their habitual daily intakes.
The average energy intake 3, ± 1, kcal and macronutrient composition [protein: 2. Food parcels, which matched each participant's habitual energy and macronutrient intakes, were supplied for 48 h before the experimental trial.
Participants were instructed to consume only food and drink sources provided by investigators and to consume their final meal no later than on the evening before the experimental protocol.
Analyses of the diet diary and food prescription were performed by using a commercially available software program Wisp v3; Tinuviel software.
All participants were instructed to refrain from physical exercise for 48 h before the experimental trial. A schematic diagram of the experimental protocol is displayed in Figure 1.
Participants reported to the laboratory at ~ following an overnight fast and measurements of height and weight were collected. A cannula was inserted into a forearm vein of one arm, and a resting blood sample was collected. Subjects rested for ~75 min before a primed, continuous 2.
Figure 1. Schematic diagram of the infusion protocol. A baseline blood sample was collected before participants consumed an energy-rich, high-protein breakfast.
A bout of unilateral leg-resistance exercise was performed 3 h after breakfast. Muscle biopsies vastus lateralis were collected from the exercised leg immediately prior 0 h , 1-, or 4 h-post drink ingestion. Drink ingestion was either a branched-chain amino acid containing beverage BCAA or placebo PLA.
Multiple blood samples were collected throughout the protocol. Ex, exercise. Approximately min later, participants performed a single bout of unilateral leg resistance exercise which lasted ~25 min. The warm up was followed by a 2 min rest period.
Participants rested for 2 min between each set and were free to consume water ad libitum. If a participant could not complete a full set, the load was lowered by 4. Rating of perceived exertion RPE using the modified Borg scale Borg, was measured after each set. Two further muscle biopsies were collected 1 and 4 h after drink ingestion.
Different incisions ~1 cm apart, proximally from previous site were used for each biopsy in an attempt to minimize the impact of local inflammation from the previous biopsy sample. Biopsy samples were immediately rinsed, blotted of excess blood, removed of visible fat and connective tissue, and divided into aliquots, before being frozen in liquid nitrogen, and stored at —80°C until later analysis.
Muscle samples were analyzed for enrichment of L-[ring- 13 C 6 ] phenylalanine in the intracellular pool and bound myofibrillar protein fractions, Furthermore, muscle was analyzed to measure the phosphorylation status of mTORC1-related signaling proteins.
Muscle tissue was analyzed for enrichment of L-[ring- 13 C 6 ] phenylalanine in the myofibrillar protein fraction.
Myofibrillar proteins were isolated from ~30 mg tissue as previously described Moore et al. Briefly muscle was snipped in ice-cold homogenizing buffer 50 mM Tris-HCL, 1 mM EDTA, 1 mM EGTA, 10 mM β-glycerophosphate, and 50 mM sodium fluoride.
The homogenate was shaken for 10 min prior to being centrifuged at 1, g for 10 min at 4°C. The pellet was then re-suspended in homogenization buffer, before being shaken and centrifuged as described above.
The myofibrillar fraction was separated from any collagen by dissolving the pellet in 0. The proteins were then precipitated by combining the supernatant with 1 M PCA before being centrifuged for 20 min at rpm at 4°C.
The remaining myofibrillar pellet was hydrolyzed overnight at °C in 0. Amino acids were then converted to their N-acetyl-n-propyl ester derivative and phenylalanine labeling was determined by gas-chromatography-combustion-isotope ratio mass spectrometry GC-C-IRMS, Delta-plus XL, Thermofinnigan, Hemel Hempstead, UK.
Intracellular amino acids were liberated from ~20 mg of muscle. The frozen tissue was powdered under liquid nitrogen using a mortar and pestle and μL of 1 M perchloric acid PCA was added.
The mixture was centrifuged at 10, g for 10 min. The supernatant was then neutralized with 2 M potassium hydroxide and 0. The free amino acids from the intracellular pool were purified on cation-exchange columns as described above. Muscle tissue 20—30 mg was powdered on dry ice and then homogenized in lysis buffer 50 mM Tris pH 7.
Samples were placed on a shaker for 1 h at 4°C, before being centrifuged for 5 min at 6, g. The supernatant was then used for determination of protein. A DC protein assay Bio Rad, Hertfordshire, UK was used for determining protein concentration.
Equal amounts of protein were then boiled in Laemmli sample buffer mM Tris-HCl, pH 6. Proteins were then transferred to a Protran nitrocellulose membrane Whatman, Dassel, Germany at V for 1 h. Membranes were blocked using milk solution and then incubated overnight at 4°C with the appropriate primary antibody.
The following morning the membrane was rinsed in wash buffer TBS with 0. The membrane was then cleared of the antibody using wash buffer. Antibody binding was detected using enhanced chemiluminescence Millipore, Billerica, MA.
Imaging and band quantification were carried out using a Chemi Genius Bioimaging Gel Doc System Syngene, Cambridge, UK. Blood was collected in lithium heparin, EDTA-containing and serum separator tubes and centrifuged at 3, rpm for 15 min at 4°C.
Plasma glucose and urea concentrations were analyzed using an instrumentation laboratory automated blood metabolite analyzer Instrumentation Laboratory , Instrumentation Laboratory, Cheshire, UK. Serum insulin concentrations were measured using a commercially available ELISA DRG Diagnostics, USA following manufacturer's instructions.
The 13 C 6 enrichments of phenylalanine and tyrosine were determined by GCMS by monitoring ions and for unlabeled and labeled phenylalanine and ions and for unlabeled and labeled tyrosine. Once thawed, plasma samples were mixed with diluted acetic acid and purified using a cation-exchange column Bio-Rad laboratories Inc.
The amino acids were then converted to their N-tert-butyldimethyl-silyl-N-methyltrifluoracetamide TBDMS derivative. Simultaneously, concentrations of phenylalanine, leucine, threonine, isoleucine, and valine were determined using an internal standard method Tipton et al. The selected amino acids were chosen to monitor blood concentrations of essential, non-essential, and the BCAAs.
Since the weight of both sample and internal standard was known, it was possible to calculate a tracer-to-tracee ratio. Since the amino acid concentrations of the internal standard were known, it was possible to convert the tracer-to-tracee ratio into a concentration of each amino acid in plasma.
To determine 15 N 2 urea enrichments, 10 μL of plasma was mixed with μL ethanol. Samples were then left in the fridge for 30 min prior to centrifugation at 13, rpm for 20 min at 4°C.
The supernatant was then removed and dried under nitrogen. TBDMS and acetonitrile were added to the dried sample prior to heating at 90°C for 90 min. Samples were then run on GCMS and ions and were monitored. The fractional synthesis rate FSR of the myofibrillar protein fraction was calculated over the 4 h period using the standard precursor-product equation below:.
Where E B B 3 is the bound 13 C 6 phenylalanine enrichment measured in the biopsy collected at the 4h time point, B 1 is the bound 13 C 6 phenylalanine enrichment measured in the biopsy collected at the 0 h time point , E IC is the average IC phenylalanine enrichment of biopsies collected at 0 and 4 h, and t is time of tracer incorporation h.
Whole body phenylalanine rate of appearance was calculated by dividing the infusion rate by plasma phenylalanine enrichment, as described previously Tipton et al.
Phenylalanine oxidation was calculated by using the phenylalanine balance model Thompson et al. Briefly, whole body phenylalanine oxidation was determined from the calculation of the hydroxylation of L-[ring- 13 C 6 ]phenylalanine to L-[ring- 13 C 6 ]tyrosine Equation 3; Thompson et al.
Total area under the curve tAUC for serum insulin concentrations, phenylalanine Ra, and phenylalanine oxidation rates were calculated using Graphpad Prism V5. tAUC-values were calculated from drink ingestion to final blood sample and a baseline y-axis value of zero for each was used.
Exercise variables, myofibrillar-MPS, and tAUC of serum insulin concentrations, and phenylalanine kinetics for the post-exercise period were analyzed using a paired samples one-tailed t -test. Given the large variability associated with phosphorylation measurements of anabolic cell signaling, a priori we decided to explore differences over time for each trial separately.
Where significance was detected, a LSD correction was used in post-hoc analysis. All statistical tests were completed using statistical package for social sciences version All values are presented as means ± SEM, unless otherwise stated. Peak leucine ± 41 μM , isoleucine ± 23 μM , and valine ± 45 μM concentrations were observed at 0.
Over a 3. Figure 2. Plasma concentrations of A leucine, B isoleucine, C valine, D phenylalanine, and E threonine pre and post ingestion of either a branched-chain amino acid containing drink BCAA, closed circles or placebo drink PLA, open circles following resistance exercise.
Data are displayed as means ± SE. Muscle intracellular and blood plasma 13 C 6 phenylalanine enrichments were stable over the measured time period of tracer incorporation 0—4 h in both trials Figure 3. Plasma 13 C 6 tyrosine enrichments increased over time during the experimental protocol data not shown.
Trial order had no effect on intracellular and plasma tracer enrichments. Figure 3. Muscle intracellular A and plasma B 13 C 6 phenylalanine enrichments pre and post ingestion of either a branched-chain amino acid containing drink BCAA, black circles or placebo drink PLA, white circles following resistance exercise.
Figure 4. Phenylalanine kinetics, expressed as, total area under the curve for phenylalanine rate of appearance A and total area under the curve for phenylalanine oxidation B following the post-exercise ingestion of a branched-chain amino acid BCAA, black bars or placebo drink PLA, white bars.
Signaling data are displayed in Figure 5. Figure 5. Figure 6. Muscle myofibrillar fractional synthesis rate following the post-exercise ingestion of a branched-chain amino acid containing drink BCAA or placebo drink PLA.
Data are displayed as means bars and individual responses dots and lines. Taken together, these results demonstrate that BCAAs exhibit the capacity to stimulate myofibrillar-MPS, however a full complement of EAA could be necessary to stimulate a maximal response of myofibrillar-MPS following resistance exercise.
This information potentially has important nutritional implications for selecting amino acid supplements to facilitate skeletal muscle hypertrophy in response to resistance exercise training and the maintenance of muscle mass during aging, unloading, or disease.
The most likely physiological explanation for the apparent attenuation of the post-exercise response of myofibrillar-MPS to BCAA ingestion in comparison to an intact protein source relates to the limited availability of amino acids as substrate for MPS. It is well-established that BCAA ingestion stimulates the activation of mTORC1 signaling pathways that regulate the translational activity of MPS Karlsson et al.
Moreover, recent results demonstrate that the presence of the valine and isoleucine enhances the response of mTORC1 to leucine Moberg et al. However, results from the present study suggest that ingesting BCAAs alone, without the other EAA, provides limited substrate for protein synthesis in exercised muscles.
Thus, the overall response of MPS is not maximized. Instead, the limited availability of EAA likely explains the qualitative difference in magnitude of the MPS response to ingestion of BCAAs alone and ingestion of similar amounts of BCAAs as part of intact whey protein Churchward-Venne et al.
Moreover, in the present study, we observed a decline in arterialized phenylalanine concentrations 3 h after drink ingestion in the BCAA trial. This finding is consistent with previous research that observed decreased EAA concentrations following leucine ingestion Hagenfeldt and Wahren, ; Nair et al.
Taken together, these data support the notion that EAA availability is the rate-limiting factor for stimulating a maximal MPS response to resistance exercise with BCAA ingestion. The decline in EAA availability that we observed with BCAA ingestion also provides a potential physiological explanation for the differential response of MPS to ingesting a BCAA source at rest and following resistance exercise.
However, consistent with the results reported in the present study, the addition of leucine to 6. Thus, exercise has been shown to alter the relationship of the MPS response to ingested leucine and intact protein. It is likely that the enhanced ability of muscle to utilize ingested protein following exercise Pennings et al.
In another study, Churchward-Venne et al. These data suggest that leucine alone may be sufficient to stimulate MPS following exercise provided a minimal amount of EAA is included.
However, Churchward-Venne et al. Leucine seems to be the most important BCAA for stimulation of the translation initiation pathways Anthony et al. Thus, it is possible that leucine is solely responsible for the stimulation in MPS we report here.
On the other hand, recent data suggest that there is a greater stimulatory effect on mTORC1 when valine and isoleucine are consumed alongside leucine Moberg et al. Nevertheless, taken together, these past and present data suggest that the availability of EAA may be a critical factor for the optimal response of MPS following resistance exercise.
Since the ingestion of BCAAs alone stimulates myofibrillar-MPS, but does not increase the supply of all EAA, the overall response of myofibrillar-MPS following resistance exercise is limited. The influence of exogenous BCAAs on the stimulation of MPS following exercise is mediated by an increased activation of the mTORC1-S6K1 signaling pathway.
In the present study, the stimulation of PRAS40 Thr , and S6K1 Thr phosphorylation 1 h following drink ingestion was higher in BCAA than PLA. Thus, our results are consistent with earlier work demonstrating an upregulation of translational activity with BCAA ingestion Karlsson et al.
The present study is the first to demonstrate the upregulation of mTORC1 signaling with BCAA ingestion translates into an increased response of myofibrillar-MPS following resistance exercise.
BCAAs has been shown to influence muscle protein metabolism through MPB as well as MPS Buse and Reid, ; Louard et al. Early studies demonstrated that BCAAs reduce whole-body protein breakdown Ferrando et al. Recent evidence suggests the impact of BCAAs on MPB may be mediated by the leucine metabolite, β-hydroxy-β-methylbutyrate Wilkinson et al.
However, to date no study has investigated the impact of BCAA supplementation on MPB following exercise. Unfortunately, it was not possible to directly measure MPB in the present study, however our data suggest that whole-body protein breakdown was reduced by BCAA ingestion.
Whereas, urea production rates were similar between trials, phenylalanine Ra, and phenylalanine oxidation rates—both indicators of whole body protein catabolism—were lower in BCAA than PLA during the 4 h period after drink ingestion. These data are consistent with previously reported results following ingestion of intact protein with similar amounts of BCAAs Witard et al.
It has been reported that MPB is reduced by insulin following resistance exercise Biolo et al. However, the insulin response to BCAA and PLA was similar, so it is unlikely that insulin explains the decline in whole-body protein breakdown following BCAA ingestion.
Thus, it seems clear that whole-body protein breakdown is decreased, albeit minimally, with ingestion of BCAAs following resistance exercise. However, these results do not necessarily mean that MPB is decreased with BCAA ingestion.
To conclude, the ingestion of BCAAs alone, without the concurrent ingestion of other EAA, intact protein or other macronutrients, increases the stimulation of mTORC1 activity and myofibrillar-MPS following exercise in resistance-trained young men.
Our data support the notion that BCAA ingestion alone does not maximally stimulate myofibrillar-MPS following exercise despite stimulation of translation initiation pathways.
The lack of sufficient EAA appears to limit the response of myofibrillar-MPS following exercise. Thus, whereas our data clearly show that BCAA ingestion activates cell-signaling pathways that result in increased myofibrillar-MPS, ingestion of BCAAs alone may not be the optimal nutritional regimen to stimulate a maximal MPS response to resistance exercise training.
SRJ, OCW, GAW, and KDT designed the research project and conducted the research; SRJ, OCW, and KDT had primary responsibility for the final content of the manuscript and wrote the manuscript; SRJ, OCW, AP, and KB analyzed data or performed statistical analysis and all authors read and approved the final manuscript.
The authors declare that the research was conducted in the absence of any commercial or financial relationships that could be construed as a potential conflict of interest. Anthony, J. Orally administered leucine stimulates protein synthesis in skeletal muscle of postabsorptive rats in association with increased eIF4F formation.
PubMed Abstract Google Scholar.
Rbeakdown some reason Plant-based desserts to our team nad at The MusclePhD, branched-chain amino acids BCAAs have really become a BCAA and muscle protein breakdown of hot debate in the fitness community. But open your mind up a little bit. Who are BCAAs trash for? Should everyone throw their BCAAs in the garbage? Branched-chain amino acids consist of 3 amino acids: leucine, isoleucine, and valine.BCAAA trait is profein related to your BCAA breakdown traitwith your Insights and Actions more prottein on breadkown impact of BCAAs mudcle building muscle. Readers are encouraged to breakeown the BCAA breakdown and BCAAs pritein Insulin mkscle articles for further background information.
Amd stands for branched chain amino musclw. It is the collective name for three particular amino acids the building blocks of proteins :. These amino acids play important roles in building new muscle proteins, providing energy BCAA and muscle protein breakdown muscles and control of glucose metabolism.
Due to these roles, BCAAs prottein a popular pre-workout breakcown, where they are used to stimulate muscle protein synthesis and boost muscle growth, muacle muscle soreness after a proteiin, and help wnd prevent fatigue during BCAA and muscle protein breakdown.
As well as being in abd supplements and protein powders, BCAA and muscle protein breakdown are also muecle in various foods, including: red meat, poultry, eggs, breakddown products, nuts, lentils and beans. Convenient on-the-go snack BCAAs are essential amino acids.
Peotein means they Balancing restrictions and goals be consumed in our nuscle as we rbeakdown synthesise them ourselves.
BCAAs have been shown BCAAA enhance the process of muscel protein synthesis — the building BCAA and muscle protein breakdown new contractile brdakdown non-contractile muscle proteins from amino acids. Breakrown discussed in the Muscle Hypertrophy trait Earth-friendly skincare remediesin breakdowb to brwakdown muscle strength and size, the rate at rpotein we build BCAAA muscle proteins i.
the rate of muscle protein synthesis needs to be greater than the rate at BCAA and muscle protein breakdown we break down or BCA muscle proein i. the Detoxification benefits of muscle protein Anti-cancer research. Therefore, by stimulating muscle protein Allergy relief through air filtration, BCAAs may assist muscle building.
In line umscle this, a Dutch study found that the BCAA and muscle protein breakdown of synthesis of myofibrillar proteins BCAA and muscle protein breakdown are BCAA and muscle protein breakdown of muscle fibres significantly increased within the first 2 hours proein ingesting 6g of BCAAs.
In particular, leucine is thought to muscl muscle protein synthesis breakdpwn stimulating a breakdoown pathway known as the mTOR pathway. To recap, the mTOR signalling pathway acts as a sensor breaidown the internal and external environment of cells, switching nad protein brakdown when breakdlwn are appropriate.
One of the appropriate conditions for protein synthesis is breamdown a good availability of amino acids, the building blocks anc proteins. When braekdown levels of BCAAs in particular, leucine increase vreakdown conjunction with mechanical vreakdown on muscles, breakddown stimulates the mTOR pathway, breakeown in Enhancing athletic performance in older adults synthesis and, if sustained over time, gains in muscle mass.
Source: Torre-Villalvazo, I. Protein intake and amino acid supplementation regulate exercise recovery and performance through the modulation of mTOR, AMPK, FGF21, and immunity.
Nutrition research, 72, As well as stimulating muscle protein synthesis, BCAAs have been shown to reduce muscle protein breakdown. For example, studies in human subjects have reported reduced muscle protein breakdown following intravenous infusion of leucine.
High levels of BCAAs circulating in the body can make various tissues, including muscle tissues, less sensitive to insulin. Reduced sensitivity to insulin can, in turn, impair muscle building.
Under normal circumstances, insulin, as well regulating metabolism of fat and glucose, acts as an anabolic hormone that stimulates muscle protein synthesis.
Insulin orchestrates its effects in the body by binding to a specialised receptor on the surface of different cells: the insulin receptor. Source: Makanae, Y. Role of Exercise and Nutrition in the Prevention of Sarcopenia. Journal of nutritional science and vitaminology, 61 SupplementSS When circulating levels of BCAAs in the bloodstream are elevated, however, this can cause excessive stimulation of the mTOR pathway and also inhibition of the insulin receptor signalling pathways.
Source: Yoon, M. The emerging role of branched-chain amino acids in insulin resistance and metabolism. Nutrients, 8 7 Insulin resistance caused by high levels of BCAAs therefore makes muscle tissue less sensitive to the anabolic effects of insulin, resulting in reduced muscle protein synthesis.
This effect is more pronounced in people performing little to no physical activity, as exercise particularly resistance exercise is also a potent activator of the mTOR pathway and muscle protein synthesis. Source: Melnik, B. Metabolic effects of milk protein intake strongly depend on pre-existing metabolic and exercise status.
High circulating levels of BCAAs can also disturb the fat metabolism of muscles, causing the accumulation of lipid within skeletal muscle.
This can worsen insulin sensitivity and impair muscle building. Another process that is negatively affected by high levels of BCAAs is autophagy. mitochondria and misfolded proteins.
This allows cells to regenerate and build new components. Within muscle tissues, autophagy is shown to be important for preserving muscle mass and maintaining the integrity of muscle fibres.
In particular, autophagy is thought to play a key role in muscle adaptations to endurance exercisesuch as the renewal of mitochondria. BCAAs, however, can inhibit autophagy, partly through the mTOR signalling pathway.
By inhibiting autophagy and renewal of key cell components in muscle fibres, high circulating levels of BCAAs can impair muscle growth and adaptations to training. As explained in the BCAA breakdown trait articlevariants of the APOA2 gene can affect how effectively you break down BCAAs, which, in turn, can influence your circulating levels of BCAAs.
This, in turn, can lead to higher circulating levels of BCAAs, which can impair muscle building. Accordingly, people with the CC genotype wishing to optimise muscle building may need to avoid both high intakes of saturated fat and BCAAs.
This may be particularly pertinent for individuals adopting a ketogenic diet which is both high in saturated fat and BCAAsor those taking BCAA supplements. In addition to APOA2 gene variants, being overweight, obese, and carrying excessive amounts of visceral fat can also impair BCAA breakdown, leading to high circulating levels of BCAAs and suboptimal muscle building outcomes.
Your BCAAs and muscle building trait analyses both your APOA2 gene variants and your lifestyle survey data including BMI, body composition, waist circumference, and fasting blood glucose levels.
A qualified doctor having attained full GMC registration inHaran also holds a first-class degree in Experimental Psychology MA Cantab from the University of Cambridge and an MSc in the philosophy of cognitive science from the University of Edinburgh. Haran is a keen runner and has successfully completed a subhour marathon during his time at FitnessGenes.
Create a FitnessGenes account to unlock your lifestyle-based reports for free, each with personalized insights and actions. No credit card details required. Login Get Started For Free. What are BCAAs? It is the collective name for three particular amino acids the building blocks of proteins : Leucine Isoleucine Valine These amino acids play important roles in building new muscle proteins, providing energy for muscles and control of glucose metabolism.
Dr Haran Sivapalan A qualified doctor having attained full GMC registration inHaran also holds a first-class degree in Experimental Psychology MA Cantab from the University of Cambridge and an MSc in the philosophy of cognitive science from the University of Edinburgh. Start Unlocking Reports For Free Create a FitnessGenes account to unlock your lifestyle-based reports for free, each with personalized insights and actions.
Get Started For Free. Get started DNA Analysis Kit DNA Data Upload Free Lifestyle Analysis. About us Our Science Our Team Blog FAQs Contact Us.
: BCAA and muscle protein breakdownBranched-chain amino acids activate key enzymes in protein synthesis after physical exercise | Through their reduction in levels of FFAs in the blood, BCAAs can help to reduce the levels of free tryptophan entering the brain, and help to reduce the sensation of fatigue as a result of exertion. BCAA also inhibits tyrosine uptake in the brain tyrosine being another aromatic amino acid, like tryptophan ; the reduced uptake depresses catecholamine synthesis and release in the brain. Catecholamines are associated with enhanced physical performance. The simultaneous reductions in both catecholamine and serotonin synthesis may account for the relatively neutral effect of BCAA on physical performance. BCAAs are also found to reduce the increase in serum levels of ammonia that occurs during exercise. This is done by increasing the amount of ammonia used in glutamine synthesis, preventing an over-accumulation of ammonia in the blood. Increased levels of ammonia in the muscle tissue also increase phosphofructokinase activity PFK , leading to an increase in lactic acid, a major contributor to muscle fatigue. In addition, BCAA supplementation has been shown to decrease levels of creatine kinase in muscle cells post exercise. Creatine kinase is an indicator of muscle damage, and is responsible for transferring a phosphate group from ATP to create a phosphocreatine molecule. Dietary BCAAs have been used in an attempt to treat some cases of hepatic encephalopathy. Certain studies suggested a possible link between a high incidence of amyotrophic lateral sclerosis ALS among professional American football players and Italian soccer players, and certain sports supplements including BCAAs. The proposed underlying mechanism is that cell hyper-excitability results in increased calcium absorption by the cell and thus brings about cell death, specifically of neuronal cells which have particularly low calcium buffering capabilities. While BCAAs can induce a hyperexcitability similar to the one observed in mice with ALS, current work does not show if a BCAA-enriched diet, given over a prolonged period, actually induces ALS-like symptoms. Blood levels of the BCAAs are elevated in obese, insulin resistant humans and in mouse and rat models of diet-induced diabetes, suggesting the possibility that BCAAs contribute to the pathogenesis of obesity and diabetes. Restriction of dietary BCAAs extends lifespan in flies, [30] while restriction of BCAAs in mice extends male lifespan and decreased frailty, but does not extend female lifespan. Contents move to sidebar hide. Article Talk. Read Edit View history. Tools Tools. What links here Related changes Upload file Special pages Permanent link Page information Cite this page Get shortened URL Download QR code Wikidata item. Download as PDF Printable version. In other projects. Wikimedia Commons. Amino acid with a branched carbon chain. This article needs more reliable medical references for verification or relies too heavily on primary sources. Please review the contents of the article and add the appropriate references if you can. Unsourced or poorly sourced material may be challenged and removed. Find sources: "Branched-chain amino acid" — news · newspapers · books · scholar · JSTOR November Huntington College of Health Sciences. Archived from the original PDF on 28 August Retrieved 22 March The Journal of Nutrition. doi : PMID The Plant Cell. PMC Advances in Molecular Biology. Dietary Reference Intakes for Energy, Carbohydrates, Fiber, Fat, Fatty Acids, Cholesterol, Protein, and Amino Acids. Washington, DC: The National Academies Press. ISBN November Proceedings of the National Academy of Sciences of the United States of America. Bibcode : PNAS.. Molecular Genetics and Metabolism. Nature Cell Biology. S2CID World Journal of Diabetes. Colombia Medica. Acta Physiologica Scandinavica. Sport Sciences for Health. European Journal of Applied Physiology. Amino Acids. Medicine and Science in Sports and Exercise. The biochemical basis of sports performance 2 ed. Oxford University Press. Retrieved 6 December Nutrition in Clinical Practice. The Cochrane Database of Systematic Reviews. Experimental Neurology. Nature Reviews. April Cell Metabolism. July Cell Reports. February The Journal of Physiology. Molecular Metabolism. May The Journals of Gerontology. Series A, Biological Sciences and Medical Sciences. January Nature Aging. Nature Metabolism. October Wikimedia Commons has media related to Branched-chain amino acids. Metabolism map. Carbon fixation. Photo- respiration. Pentose phosphate pathway. Citric acid cycle. Glyoxylate cycle. Urea cycle. Fatty acid synthesis. Fatty acid elongation. Beta oxidation. beta oxidation. Glyco- genolysis. MTORC1 and the regulation of skeletal muscle anabolism and mass. Interactions of the super complexes: when mTORC1 Meets the proteasome. Adeva-Andany, M. Enzymes involved in branched-chain amino acid metabolism in humans. Amino Acids 49, — Aftring, R. Effects of diabetes and starvation on skeletal muscle branched-chain α-keto acid dehydrogenase activity. Ahlborg, G. Substrate turnover during prolonged exercise in man. Splanchnic and leg metabolism of glucose, free fatty acids, and amino acids. Akita, K. Inhibition of branched-chain α-ketoacid dehydrogenase kinase by thiamine pyrophosphate at different potassium ionic levels. Ambros, V. The functions of animal microRNAs. Nature , — Ananieva, E. Roitberg Amsterdam: Elsevier. Anderson, K. SIRT4 Is a Lysine deacylase that controls leucine metabolism and insulin secretion. Cell Metab. Andersson-Hall, U. Higher concentrations of BCAAs and 3-HIB are associated with insulin resistance in the transition from gestational diabetes to type 2 diabetes. Diabetes Res. Andrade, M. PPARγ-induced upregulation of subcutaneous fat adiponectin secretion, glyceroneogenesis and BCAA oxidation requires mTORC1 activity. Acta - Mol. Cell Biol. Lipids Baron, K. Circadian misalignment and health. Psychiatry 26, — Bar-Peled, L. Regulation of mTORC1 by amino acids. Trends Cell Biol. Barsotti, G. Protection of renal function and of nutritional status in uremic rats by means of a low-protein, low-phosphorus supplemented diet. Nephron 49, — Basciano, H. Fructose, insulin resistance, and metabolic dyslipidemia. Béchet, D. Regulation of skeletal muscle proteolysis by amino acids. Renal Nutr. Biswas, D. Role of branched-chain amino acid—catabolizing enzymes in intertissue signaling, metabolic remodeling, and energy homeostasis. FASEB J. Blagosklonny, M. Fasting and rapamycin: diabetes versus benevolent glucose intolerance. Cell Death Dis. Bledsoe, R. Cloning of the rat and human mitochondrial branched chain aminotransferases BCAT m. Acta - Protein Struct. CrossRef Full Text Google Scholar. Block, K. Glucocorticoid-mediated activation of muscle branched-chain α-keto acid dehydrogenase in vivo. Bodine, S. Identification of ubiquitin ligases required for skeletal Muscle Atrophy. Science , — Bond, P. Regulation of mTORC1 by growth factors, energy status, amino acids and mechanical stimuli at a glance. Sports Nutr. Boyer, B. Kinetic characterization of branched chain ketoacid dehydrogenase. Brabin, B. Brosnan, J. Branched-Chain amino acids: enzyme and substrate regulation. Burrage, L. Branched-chain amino acid metabolism: From rare Mendelian diseases to more common disorders. Cam, H. mTORC1 signaling under hypoxic conditions is controlled by atm-dependent phosphorylation of HIF-1α. Cell 40, — Cano, N. Branched-Chain amino-acid metabolism in renal failure. Castellino, P. Effect of insulin and plasma amino acid concentrations on leucine metabolism in man. Role of substrate availability on estimates of whole body protein synthesis. Chaillou, T. Time course of gene expression during mouse skeletal muscle hypertrophy. Chantranupong, L. The sestrins interact with gator2 to negatively regulate the amino-acid-sensing pathway upstream of mTORC1. Cell Rep. Chen, S. Involvement of ammonia metabolism in the improvement of endurance performance by tea catechins in mice. Chen, X. Branched-chain amino acids and the association with type 2 diabetes. Diabetes Investig 6, — Cheng, Y. PLoS One e Cheon, S. The ubiquitin ligase UBE3B, disrupted in intellectual disability and absent speech, regulates metabolic pathways by targeting BCKDK. Cheon, W. Change of gene expression on protein uptake composition and hindlimb-suspension in rat skeletal muscle. Chevalier, S. The influence of sex on the protein anabolic response to insulin. Metabolism 54, — Chuang, D. Branched-Chain amino acids? Chuang, J. Production of recombinant mammalian holo-E2 and E3 and reconstitution of functional branched-chain α-keto acid dehydrogenase complex with recombinant E1. Methods Enzymol. Church, D. Mitigation of muscle loss in stressed physiology: Military relevance. Nutrients 11, 1— Comitato, R. Sex Hormones and Macronutrient Metabolism. Food Sci. Conway, M. Schousboe and U. Sonnewald Salmon Tower Building, NY: Springer International Publishing , 99— Emerging moonlighting functions of the branched-chain aminotransferase proteins. Antioxid Redox Signal. Human mitochondrial branched chain aminotransferase: Structural basis for substrate specificity and role of redox active cysteines. Acta - Proteins Proteomics , 61— Crossland, H. Exploring mechanistic links between extracellular branched-chain amino acids and muscle insulin resistance: an in vitro approach. Cell Physiol. Damuni, Z. Purification and properties of the catalytic subunit of the branched-chain alpha-keto acid dehydrogenase phosphatase from bovine kidney mitochondria. David, J. Impaired skeletal muscle branched-chain amino acids catabolism contributes to their increased circulating levels in a non-obese insulin-resistant fructose-fed rat model. Davoodi, J. Overexpression and characterization of the human mitochondrial and cytosolic branched-chain aminotransferases. Dhanani, Z. Depletion of branched-chain aminotransferase 2 BCAT2 enzyme impairs myoblast survival and myotube formation. Dickinson, J. Aging differentially affects human skeletal muscle amino acid transporter expression when essential amino acids are ingested after exercise. Dolatabad, M. Crystal structure and catalytic activity of the PPM1K N94K mutant. Dos Santos, R. Effect of exercise on glutamine synthesis and transport in skeletal muscle from rats. Edozien, J. Diet-hormone interrelationships in the rat. Ericksen, R. Loss of bcaa catabolism during carcinogenesis enhances mTORC1 activity and promotes tumor development and progression. Espinal, J. Effects of low-protein diet and starvation on the activity of branched-chain 2-oxo acid dehydrogenase kinase in rat liver and heart. Exner, R. Glutamine deficiency renders human monocytic cells more susceptible to specific apoptosis triggers. Surgery , 75— Fan, B. Neuroprotective strategy in retinal degeneration: Suppressing ER stress-induced cell death via inhibition of the mTOR signal. Fan, L. Krüppel-like factor Regulator of BCAA metabolism and circadian protein rhythmicity. Ferrière, G. Abnormalities of muscle fibers in maple syrup urine disease. Acta Neuropathol. Fielding, R. The effects of high intensity exercise on muscle and plasma levels of alpha-ketoisocaproic acid. Fisch, S. Kruppel-like factor 15 is a regulator of cardiomyocyte hypertrophy. Flores-Guerrero, J. Plasma branched-chain amino acids and risk of incident type 2 Diabetes: Results from the PREVEND prospective cohort study. Fujii, H. Branched-chain α-keto acid dehydrogenase kinase content in rat skeletal muscle is decreased by endurance training. Gao, X. Gardiner, K. Intestinal amino acid absorption during sepsis. Giesbertz, P. Diabetologia 58, — Goto, M. Structural determinants for branched-chain aminotransferase isozyme-specific inhibition by the anticonvulsant drug gabapentin. Gran, P. The actions of exogenous leucine on mTOR signalling and amino acid transporters in human myotubes. BMC Physiol. Green, M. Are fatty acids gluconeogenic precursors? Groper, S. Advanced Nutrition and Human Metabolism, 6th Edn, Gürke, J. Maternal diabetes leads to adaptation in embryonic amino acid metabolism During early pregnancy. Hall, S. A trial for the ages. Hall, T. Branched chain aminotransferase isoenzymes. Purification and characterization of the rat brain isoenzyme. Hara, Y. Acidosis, not azotemia, stimulates branched-chain, amino acid catabolism in uremic rats. Kidney Int. Harper, E. Branched-chain amino acid metabolism. Metabolism 30, — Harris, R. Regulation of branched-chain α-keto acid dehydrogenase kinase expression in rat liver. Regulation branched-chain and elucidation of a molecular basis for maple syrup urine. Hassan, B. Hasselgren, P. Current concepts of protein turnover and amino acid transport in liver and skeletal muscle during sepsis. Hattori, A. Cancer progression by reprogrammed BCAA metabolism in myeloid leukemia. Helms, E. Evidence-based recommendations for natural bodybuilding contest preparation: nutrition and supplementation. Hemmings, B. Cold Spring Harb. Henriksbo, B. Statins activate the NLRP3 inflammasome and impair insulin signaling via p38 and mTOR. Henriksson, J. Redox state changes in human skeletal muscle after isometric contraction. Hernández-Alvarez, M. Early-onset and classical forms of type 2 diabetes show impaired expression of genes involved in muscle branched-chain amino acids metabolism. Holecek, M. Branched-chain amino acids and ammonia metabolism in liver disease: therapeutic implications. Nutrition 29, — Holeček, M. Branched-chain amino acids in health and disease: Metabolism, alterations in blood plasma, and as supplements. Why are branched-chain amino acids increased in starvation and diabetes? Nutrients 12, 1— Acute hyperammonemia activates branched-chain amino acid catabolism and decreases their extracellular concentrations: different sensitivity of red and white muscle. Amino Acids 40, — Glutamine deficiency in extracellular fluid exerts adverse effects on protein and amino acid metabolism in skeletal muscle of healthy, laparotomized, and septic rats. Amino Acids 46, — Leucine metabolism in rat liver after a bolus injection of endotoxin. Metabolism 47, — Hosokawa, N. Nutrient-dependent mTORCl association with the ULK1-AtgFIP complex required for autophagy. Cell 20, — Howarth, K. Exercise training increases branched-chain oxoacid dehydrogenase kinase content in human skeletal muscle. Huang, Y. Down-regulation of rat mitochondrial branched-chain 2-oxoacid dehydrogenase kinase gene expression by glucocorticoids. Biochem , — Hutson, S. Blood and tissue branched-chain amino and α-keto acid concentrations: Effect of diet, starvation, and disease. Ichikawa, T. Effect of an oral branched chain amino acid-enriched snack in cirrhotic patients with sleep disturbance. Iizuka, K. The transcription factor carbohydrate-response element-binding protein ChREBP : A possible link between metabolic disease and cancer. Basis Dis. Indo, Y. Altered kinetic properties of the branched-chain α-keto acid dehydrogenase complex due to mutation of the β-subunit of the branched-chain α-keto acid decarboxylase E1 component in lymphoblastoid cells derived from patients with maple syrup urine disease. Invest 80, 63— Ishikawa, T. Muscle-specific deletion of BDK amplifies loss of myofibrillar protein during protein undernutrition. Islam, M. Branched-chain amino acid metabolon: Interaction of glutamate dehydrogenase with the mitochondrial branched-chain aminotransferase BCATm. A novel branched-chain amino acid metabolon: Protein-protein interactions in a supramolecular complex. Jang, C. A branched-chain amino acid metabolite drives vascular fatty acid transport and causes insulin resistance. Jeong, Y. Integrated expression profiling and Genome-Wide analysis of ChREBP targets reveals the dual role for ChREBP in Glucose-Regulated gene expression. PLoS One 6:e Jeyaraj, D. Klf15 orchestrates circadian nitrogen homeostasis. Jiang, J. Starve cancer cells of glutamine: Break the spell or make a hungry monster? Cancers Basel Jones, J. Three human peroxisomal 2-hydroxy acid oxidases. Biochemistry , — Kainulainen, H. Potential role of branched-chain amino acid catabolism in regulating fat oxidation. Sport Sci. Kamei, Y. Regulation of skeletal muscle function by amino acids. Nutrients Kawaguchi, T. Insulin resistance and chronic liver disease. World J. Keenan, M. Alternative fuels for cancer cells. Cancer J. United States 21, 49— Kimura, K. Kitsy, A. Effects of leucine supplementation and serum withdrawal on branched-chain amino acid pathway gene and protein expression in mouse adipocytes. PLoS One 9:e Knapik, J. Leucine metabolism during fasting and exercise. Kobayashi, R. Clofibric acid stimulates branched-chain amino acid catabolism by three mechanisms. Gender difference in regulation of branched-chain amino acid catabolism. Hepatic branched-chain α-keto acid dehydrogenase complex in female rats: activation by exercise and starvation. Tokyo 45, — Experimental hyperthyroidism causes inactivation of the branched-chain α-ketoacid dehydrogenase complex in rat liver. Korenaga, K. Effects of a late evening snack combined with α-glucosidase inhibitor on liver cirrhosis. Kwon, G. Signaling elements involved in the metabolic regulation of mTOR by nutrients, incretins, and growth factors in islets. Diabetes 53, S—S Lackey, D. Regulation of adipose branched-chain amino acid catabolism enzyme expression and cross-adipose amino acid flux in human obesity. Lee, J. Branched-chain amino acids sustain pancreatic cancer growth by regulating lipid metabolism. Lei, M. Acetylation promotes BCAT2 degradation to suppress BCAA catabolism and pancreatic cancer growth. Transduct Target Ther. Leontieva, O. Rapamycin reverses insulin resistance IR in high-glucose medium without causing IR in normoglycemic medium. Lerin, C. Defects in muscle branched-chain amino acid oxidation contribute to impaired lipid metabolism. Li, H. MiRa-3p-KLFBCAA regulates the skeletal muscle branched-chain amino acid metabolism in nile tilapia Oreochromis niloticus During Starvation. Li, J. BCAT2-mediated BCAA catabolism is critical for development of pancreatic ductal adenocarcinoma. Lian, K. Impaired adiponectin signaling contributes to disturbed catabolism of branched-chain amino acids in diabetic mice. Diabetes 64, 49— Liebich, H. Hydroxycarboxylic and oxocarboxylic acids in urine:products from branched-chain amino acid degradation and from ketogenesis. B Biomed. Lieu, E. Amino acids in cancer. Litwer, S. Reversion of the maple syrup urine disease phenotype of impaired branched chain α-ketoacid dehydrogenase complex activity in fibroblasts from an affected child. Liu, D. Low-protein diet supplemented with ketoacids delays the progression of diabetic nephropathy by inhibiting oxidative stress in the KKAy mice model. Liu, R. Leucine supplementation differently modulates branched-chain amino acid catabolism, mitochondrial function and metabolic profiles at the different stage of insulin resistance in rats on high-fat diet. Nutrients 9, 1— Liu, Y. Adiponectin corrects high-fat diet-induced disturbances in muscle metabolomic profile and whole-body glucose homeostasis. Diabetes 62, — Lu, G. Genes Dev. Protein phosphatase 2Cm is a critical regulator of branched-chain amino acid catabolism in mice and cultured cells. Lu, J. Hedgehog signaling pathway mediates invasion and metastasis of hepatocellular carcinoma via ERK pathway. Acta Pharmacol. Sin 33, — Lynch, C. Global deletion of BCATm increases expression of skeletal muscle genes associated with protein turnover. Genomics 47, — Lynis Dohm, G. Protein degradation during endurance exercise and recovery. Sports Exerc. MacDonald, M. Glucose regulates leucine-induced insulin release and the expression of the branched chain ketoacid dehydrogenase E1α subunit gene in pancreatic islets. Machius, M. Structure of rat BCKD kinase: Nucleotide-induced domain communication in a mitochondrial protein kinase. Maniar, S. Supplemented low-protein diets protect the rat kidney without causing undernutrition. Lab Clin. Mann, G. Effects of ketoisocaproic acid and inflammation on glucose transport in muscle cells. Manoli, I. Disorders of branched chain amino acid metabolism. Rare Dis. Marchesini, G. Nutritional supplementation with branched-chain amino acids in advanced cirrhosis: A double-blind, randomized trial. Gastroenterology , — Martina, J. MTORC1 functions as a transcriptional regulator of autophagy by preventing nuclear transport of TFEB. Autophagy 8, — Matés, J. Dysregulation of glutaminase and glutamine synthetase in cancer. Cancer Lett. Mathias, M. Fatty acid and cholesterol synthesis from specifically labeled leucine by isolated rat hepatocytes. Lipids 16, — Mayers, J. Metabolism in Mutant Kras -driven. Cancers , — Mazzulla, M. Endurance exercise attenuates postprandial whole-body leucine balance in trained men. Mccormack, S. Circulating branched-chain amino acid concentrations are associated with obesity and future insulin resistance in children and adolescents. Obes 8, 52— McGarrah, R. Dietary branched-chain amino acid restriction alters fuel selection and reduces triglyceride stores in hearts of Zucker fatty rats. Meisinger, E. Hyperfiltration due to amino and keto acid supplements of low-protein diets: Influence on proteinuria. Transfus Med. Hemotherapy 14, 26— Menkes, J. A new syndrome: progressive familial infantile cerebral dysfunction associated with an unusual urinary substance. Pediatrics 14, — Minnich, A. A potent PPARα agonist stimulates mitochondrial fatty acid β-oxidation in liver and skeletal muscle. Mitch, W. Metabolic consequences of uremia: extending the concept of adaptive responses to protein metabolism. Kidney Dis. Moberg, M. Activation of mTORC1 by leucine is potentiated by branched-chain amino acids and even more so by essential amino acids following resistance exercise. Moghei, M. Ketoisocaproic acid, a metabolite of leucine, suppresses insulin-stimulated glucose transport in skeletal muscle cells in a BCAT2-dependent manner. Mullard, A. Anti-ageing pipeline starts to mature. Drug Discov. Murakami, T. Dissociation of branched-chain α-keto acid dehydrogenase kinase BDK from branched-chain α-keto acid dehydrogenase complex BCKDC by BDK inhibitors. Tokyo 51, 48— Murton, A. Muscle atrophy in immobilization and senescence in humans. Nakashima, K. Leucine suppresses myofibrillar proteolysis by down-regulating ubiquitin-proteasome pathway in chick skeletal muscles. Nakaya, Y. BCAA-enriched snack improves nutritional state of cirrhosis. Nutrition 23, — Nazio, F. MTOR inhibits autophagy by controlling ULK1 ubiquitylation, self-association and function through AMBRA1 and TRAF6. Neinast, M. Quantitative analysis of the whole-body metabolic fate of branched-chain amino acids cell metabolism quantitative analysis of the whole-body metabolic fate of branched-chain amino acids. Nellis, M. Insulin increases branched-chain α-ketoacid dehydrogenase kinase expression in Clone 9 rat cells. Newgard, C. Branched-chain amino acid-related metabolic signature that differentiates obese and lean humans and contributes to insulin resistance. Newsholme, E. Supplementation of diets with nutritional pharmaceuticals. Nutrition 13, — Nishitani, S. Branched-chain amino acids improve glucose metabolism in rats with liver cirrhosis. Gastrointest Liver Physiol. Nissen, S. Effect of leucine metabolite β-hydroxy-β-methylbutyrate on muscle metabolism during resistance-exercise training. Nobukuni, Y. Maple syrup urine disease: Complete defect of the E1β subunit of the branched chain α-ketoacid dehydrogenase complex due to a deletion of an bp repeat sequence which encodes a mitochondrial targeting leader peptide in a family with the disease. Invest 87, — Complete Primary Strcture of the Transacylase E2b subunit of the human branched chain α-keto acid dehydrogenase complex. Norton, L. Leucine regulates translation initiation of protein synthesis in skeletal muscle after exercise. Novarino, G. Mutations in BCKD-kinase lead to a potentially treatable form of autism with epilepsy. Obayashi, M. Regulation of the activity of branched-chain 2-oxo acid dehydrogenase BCODH complex by binding BCODH kinase. FEBS Lett. Estrogen controls branched-chain amino acid catabolism in female rats. Otulakowski, G. Isolation and sequence determination of cDNA clones for porcine and human lipoamide dehydrogenase. Homology to other disulfide oxidoreductase. Owen, A. Chronic muscle weakness and mitochondrial dysfunction in the absence of sustained atrophy in a preclinical sepsis model. Elife 8, 1— Pan, B. Sin 36, — Park, J. Effects of branched-chain amino acids BCAAs on the progression of advanced liver disease: A Korean nationwide, multicenter, retrospective, observational, cohort study. United States 96, 1—7. Parker, P. Partial purification and properties of branched-chain 2-oxo acid dehydrogenase of ox liver. Paul, H. Leucine oxidation and protein turnover in clofibrate-induced muscle protein degradation in rats. Invest 65, — Paxton, R. Regulation of branched-chain α-ketoacid dehydrogenase kinase. Peng, C. Editorial: micrornas as new players in endocrinology. Perng, W. Metabolomic profiles and childhood obesity. Obesity 22, — Poortmans, J. Distribution of plasma amino acids in humans during submaximal prolonged exercise. Popov, K. Purification and comparative study of the kinases specific for branched chain α-ketoacid dehydrogenase and pyruvate dehydrogenase. Protein Expr. Dietary control and tissue specific expression of branched-chain α-ketoacid dehydrogenase Kinase. Branched-chain α-ketoacid dehydrogenase kinase: molecular cloning, expression, and sequence similarity with histidine protein kinases. Refsum, H. Changes in plasma amino acid distribution and urine amino acids excretion during prolonged heavy exercise. Lab Invest 39, — Rijo-Ferreira, F. Genomics of circadian rhythms in health and disease. Genome Med. Rivera, M. Effect of valine on myotube insulin sensitivity and metabolism with and without insulin resistance. Cell Biochem. Effect of metformin on myotube BCAA catabolism. Roberson, P. Skeletal muscle amino acid transporter and BCAT2 expression prior to and following interval running or resistance exercise in mode-specific trained males. Amino Acids 50, — Rodríguez, T. The increased skeletal muscle protein turnover of the streptozotozin diabetic rat is associated with high concentrations of branched-chain amino acids. Roth, E. Metabolic disorders in severe abdominal sepsis: glutamine deficiency in skeletal muscle. Ruderman, N. Malonyl-CoA, fuel sensing, and insulin resistance. Sahlin, K. NADH in human skeletal muscle during short-term intense exercise. Pflügers Arch. Scheer, F. Adverse metabolic and cardiovascular consequences of circadian misalignment. She, P. Leucine and Protein Metabolism in Obese Zucker Rats. PLoS One 8, e Disruption of BCATm in mice leads to increased energy expenditure associated with the activation of a futile protein turnover cycle. Cell 6, — Obesity-related elevations in plasma leucine are associated with alterations in enzymes involved in branched-chain amino acid metabolism. Disruption of BCAA metabolism in mice impairs exercise metabolism and endurance. Shi, T. MiRp negatively regulates ovine preadipocyte differentiation by targeting branched-chain aminotransferase 2. Shimomura, Y. Branched-chain 2-oxo acid dehydrogenase complex activation by tetanic contractions in rat skeletal muscle. BBA Gen. Branched-chain α-keto acid dehydrogenase complex in rat skeletal muscle: Regulation of the activity and gene expression by nutrition and physical exercise. Branched-chain amino acid catabolism in exercise and liver disease. Amino Acid Workshop Exercise Promotes BCAA Catabolism: effects of BCAA supplementation on skeletal muscle during exercise. Exercise promotes BCAA catabolism: effects of BCAA supplementation on skeletal muscle during exercise. Purification and partial characterization of branched-chain α-ketoacid dehydrogenase kinase from rat liver and rat heart. Regulation of branched-chain amino acid catabolism: Nutritional and hormonal regulation of activity and expression of the branched-chain α-keto acid dehydrogenase kinase. Care 4, — Shou, J. The effects of BCAAs on insulin resistance in athletes. Tokyo 65, — Siddik, M. Recent progress on branched-chain amino acids in obesity, diabetes, and beyond. Siddiqui, R. The regulation of fatty acid and branched-chain amino acid oxidation in cancer cachectic rats: a proposed role for a cytokine, eicosanoid, and hormone trilogy. Sivanand, S. Emerging roles for branched-chain amino acid metabolism in cancer. Cancer Cell 37, — Sonnet, D. Metformin inhibits Branched Chain Amino Acid BCAA derived ketoacidosis and promotes metabolic homeostasis in MSUD. Sparks, B. |
5 Proven Benefits of BCAAs (Branched-Chain Amino Acids) | Wall, B. This equalizes the leucine content between proteins which also equalizes the muscle protein synthesis response 19 , Article CAS PubMed Google Scholar Alvestrand A, Fürst P, Bergström J. Therefore, by stimulating muscle protein synthesis, BCAAs may assist muscle building. van Geldermalsen, M. Metformin inhibits Branched Chain Amino Acid BCAA derived ketoacidosis and promotes metabolic homeostasis in MSUD. CAS Google Scholar Borghi L, Lugari R, Montanari A, Dall'Argine P, Elia GF, Nicolotti V, et al. |
Muscle protein synthesis | Muscle wasting is a sign of malnutrition and occurs with chronic infections, cancer, periods of fasting , and as a natural part of the aging process 19 , Watson P, Shirreffs SM, Maughan RJ. Resistance exercise increases postprandial muscle protein synthesis in humans. Nitrogen sparing induced by leucine compared with that induced by its keto analogue, alpha-ketoisocaproate, in fasting obese man. Plasma and skeletal muscle free amino acids in type I, insulin-treated diabetic subjects. Abnormalities of muscle fibers in maple syrup urine disease. |
Background | BCAAs may offer health benefits for people with cirrhosis, a chronic disease in which the liver does not function properly. While certain sugars and antibiotics are the mainstays of treatment for hepatic encephalopathy, BCAAs may also benefit people with this condition One review of 16 studies including people with hepatic encephalopathy found that taking BCAA supplements had a beneficial effect on the symptoms and signs of the disease, but had no effect on mortality Liver cirrhosis is also a major risk factor for the development of hepatocellular carcinoma, the most common form of liver cancer, for which BCAA supplements may also be useful 28 , 29 , Several older studies have shown that taking BCAA supplements may offer protection against liver cancer in people with liver cirrhosis 31 , As such, scientific authorities recommend these supplements as a nutritional intervention for liver disease to prevent complications BCAA supplements may improve the health outcomes of people with liver disease, while also possibly protecting against liver cancer. BCAAs are found in foods and whole protein supplements. Getting BCAAs from complete protein sources is more beneficial, as they contain all the essential amino acids. Fortunately, BCAAs are available in a variety of food sources. This makes BCAA supplements unnecessary for most, especially if you consume enough protein in your diet already Consuming protein-rich foods will also provide you with other important nutrients that BCAA supplements lack. The best food sources of BCAAs include 35 , 36 , 37 , 38 , 39 , 40 , 41 , 42 , 43 , 44 :. Many protein-rich foods contain high amounts of BCAAs. If you consume enough protein in your diet, BCAA supplements are unlikely to provide additional benefits. The branched-chain amino acids BCAAs are a group of three essential amino acids: leucine, isoleucine, and valine. BCAA supplements have been shown to build muscle, decrease muscle fatigue , and alleviate muscle soreness. They have also successfully been used in a hospital setting to prevent or slow muscle loss and to improve symptoms of liver disease. However, because most people get plenty of BCAAs through their diet, supplementing with BCAA is unlikely to provide additional benefits. Our experts continually monitor the health and wellness space, and we update our articles when new information becomes available. BCAA stands for branched-chain amino acids. These are essential amino acids with several benefits for muscle growth and performance. While pre-workout supplements may boost your exercise performance, you may be worried about side effects. Here are 5 side effects of pre-workout…. Glutamine is an important amino acid. This article discusses the benefits, uses and side effects of glutamine supplements. Pre-workout supplements are designed to help you gain muscle by allowing you to work out harder and longer. Here are the 10 best pre-workout…. This is a detailed article about whey protein and its health benefits. It can help you lose weight and gain muscle, while improving your overall…. Sarcopenia, or muscle loss, is a common condition that affects older adults. This article explains what causes sarcopenia and how to fight it. Learn about the best pre-workout nutrition strategies. A significant decrease in valine concentration in the gastrocnemius muscle was found only in rats with acidosis [ 61 ]. BCAAs and BCKAs are supplied to patients with CRF together with other essential amino acids and their ketoanalogues to decrease protein intake as much as possible to maintain protein balance and avoid its deleterious effects on urea levels [ 65 , 66 ]. Increased BCAA concentrations are found in various insulin-deficient and -resistant states, especially diabetes and obesity. Very high BCAA and BCKA concentrations are found in maple syrup urine disease MSUD. High BCAA levels in subjects with defective insulin secretion were first described in dogs with experimental diabetes [ 67 ]. Further studies have shown that in addition to the increase of BCAAs, there is a decrease in levels of gluconeogenic amino acids, especially ALA [ 68 , 69 , 70 ]. Most data on pathogenesis of high levels of the BCAA in diabetes type 1 originate from studies using animals with diabetes induced by streptozotocin or alloxan. There are some similarities in the pathogenesis of the increased BCAAs in diabetes and short-term starvation, which is also an insulin deficient state. As in starvation, a role play activated amination of the BCKAs in the liver and impaired uptake of the BCAA by muscles. The BCKA levels increase in blood plasma and muscles of rats with chemically-induced diabetes, but decline in the liver [ 71 ]. The role of the liver as a source of BCAAs is supported by observations of reduced activity of hepatic BCKD in rats with severe ketotic diabetes [ 72 ]. However, unlike brief starvation, the changes in diabetes are associated with marked increase in proteolysis and BCKD activity in muscles, resulting in severe cachexia [ 73 ]. While muscle nitrogen repletion occurs and BCAA levels are normalized after feeding of previously starving subjects, the BCAAs accumulate and diminished nitrogen repletion remains after feeding in subjects with type 1 diabetes [ 74 ]. Plasma concentrations of BCAAs are frequently elevated in obesity and type 2 diabetes [ 75 , 76 , 77 ]. The mechanism responsible for the increased BCAAs in these insulin-resistant states is not completely clear. The studies have shown that the BCAA levels in obesity correlate with insulin resistance and are a sensitive predictor of diabetes in the future [ 78 , 79 ]. Recent studies have suggested that high levels of the BCAA interfere with oxidation of fatty acids in muscles, leading to accumulation of various acylcarnitines and insulin resistance [ 24 ]. Conflicting results have been reported concerning the effects of BCAA supplementation in subjects with insulin resistance. Arakawa et al. On the other hand, Newgard et al. White et al. MSUD is recessive disorder caused by a severe deficiency of BCKD activity. All three BCAAs, as well as the corresponding BCKAs, are elevated in blood, tissues, and urine. High BCAA and BCKA levels are related to excitotoxicity, energy deficit, and oxidative stress in the brain, resulting in severe neurological symptoms. BCAA administration to subjects with MSUD is inappropriate. DNA damage in the hippocampus and the striatum was demonstrated after administration of BCAAs in an animal model of MSUD [ 83 ]. Current treatment of MSUD is based on protein restriction and synthetic formulas with reduced BCAA content. Perspective may be phenylbutyrate, which activates BCKD and decreases BCAA and BCKA levels [ 55 , 56 ]. Unfortunately, studies examining phenylbutyrate in MSUD patients are unique. Long-term studies in different MSUD phenotypes are indicated to verify phenylbutyrate efficacy. Physical exercise is associated with enhanced BCAA oxidation and GLN release from muscles [ 84 , 85 ]. Evidence suggests that BCKD is activated by dephosphorylation mediated by falling ATP levels within the muscles during exercise. Training appears to increase mRNA expression of this enzyme [ 86 ]. The plasma BCAA levels during or after exercise have been reported to be unchanged [ 87 ], to decrease [ 88 ], or to increase [ 89 ]. The cause of inconsistent response can be explained by different work load and duration of exercise. BCAAs are recognized as supplements for athletes with a number of benefits, notably on muscle protein synthesis, fatigue recovery, and exercise-induced muscle damage [ 90 ]. In addition to the positive reports, there are a number of reports showing no benefits of BCAA supplementation [ 91 ]. Of special interest should be findings of enhanced blood ammonia levels after BCAA administration during exercise suggesting that exogenous BCAA may exert negative effects on muscle performance via ammonia [ 92 , 93 ]. Additional studies are needed to assess the true efficacy of BCAA supplementation on muscle performance and fatigue. There are several hypermetabolic states e. sepsis, burn injury, trauma, and cancer in which alterations in BCAA levels are not consistent, with increased, unchanged, and decreased levels being reported. Present in all of these conditions is systemic inflammatory response syndrome SIRS characterized by a wide range of neuro-humoral abnormalities, including enhanced production of cytokines, sympathetic nervous system activation, and cortisol production. These events cause several alterations in metabolism, including insulin resistance and enhanced myofibrillar protein degradation, resulting in severe depletion of lean body mass. If the hypermetabolic state persists, multisystem organ failure and eventually death may occur Fig. Main alterations in protein and BCAA metabolism in disorders accompanied by SIRS. In this situation, BCAAs act as a significant energy substrate for muscles [ 4 , 5 , 94 ]. Increased BCAA oxidation is coupled with increased synthesis of GLN, which is released from muscles and utilized, preferably by the immune system. Utilization of GLN often exceeds its synthesis, leading to a lack of GLN in blood and tissues [ 95 , 96 ]. Decreased GLN availability can become rate-limiting for key functions of immune cells, such as phagocytosis and antibody production. Decreased GLN levels have been shown to act as a driving force for BCAA utilization in muscles [ 97 ]. Studies have also indicated that inflammatory signals decrease BCAA absorption from the gut and inhibit BCAA transport from the blood to muscles, while promoting transport into the liver [ 98 , 99 ]. The BCAA synthesis from the BCKA in visceral tissues is probably activated. A marked increase in leucine release was observed by the isolated liver of endotoxin-treated animals after the addition of KIC into perfusion medium [ 7 ]. The cause of inconsistent alterations in BCAA levels although their oxidation is remarkably activated are different influences of individual metabolic changes occurring in the SIRS. Increased protein breakdown or decreased protein synthesis in muscles and insulin resistance may enhance the BCAA levels. Activation of BCAA catabolism associated with enhanced ALA and GLN production in muscles and protein synthesis in visceral tissues decrease the BCAA levels. Therefore, alterations in BCAA levels are inconsistent. Rationales for the use of BCAA supplements in conditions with SIRS are their enhanced oxidation, which may limit their availability in tissues and their protein anabolic properties. Benefits of BCAAs may also be related to their role as a precursor of GLN, which is a key factor in maintaining immune functions and gut integrity, and has a favorable influence on protein balance. Various solutions containing different amounts and proportions of individual BCAA have been used to examine their effects in trauma, burn, or sepsis. A number of investigators have reported that BCAA ameliorate negative nitrogen balance [ , , ]. However, the results of other investigators have not been impressive, and there is no scientific consensus regarding the effect BCAA-enriched formulas on protein balance, length of hospital stay, and mortality [ , , ]. A serious shortcoming of most of the studies is the lack of information regarding BCAA concentrations in blood and tissues, which may be suggested as a possible criterion of eligibility of the indication. The low effectiveness of the BCAA in disorders with the presence of SIRS may be related to insulin resistance and metabolic alteration associated with inflammation. Studies have shown that inflammatory response blunts the anabolic response to BCAA administration. Lang and Frost [ ] demonstrated that leucine induced activation of eukaryotic initiation factor eIF4E is abrogated in endotoxin-treated rats and that endotoxin treatment antagonized the leucine-induced phosphorylation of ribosomal protein S6 and mTOR. In recent years articles have emerged suggesting positive effects of BCAA in traumatic brain injury. In rodents, BCAAs have demonstrated to ameliorate injury-induced cognitive impairment [ ], and clinical studies have demonstrated that BCAAs enhance the cognitive recovery in patients with severe traumatic brain injury [ , ]. Unlike other states accompanied by SIRS, muscle wasting and amino acid mobilization from muscles in subjects with cancer may be driven by secretion of different tumor-derived mediators. Therefore, progressive depletion of muscle mass may be observed in some cancer patients. Also high rates of BCAA oxidation in muscles of subjects with cancer have been reported [ ]. Increasing evidence demonstrates that BCAAs are essential nutrients for cancer growth and are used as a source of energy by tumors. Expression of the cytosolic type of BCAT has been shown to correlate with more aggressive cancer growth [ ]. The findings of clinical trials examining the effects of BCAA-enriched nutritional support to cancer patients are inconsistent. Some showed improved nitrogen balance and reduced skeletal muscle catabolism whereas others show no significant improvement [ ]. A concern in the tumor-bearing state is that provision of the BCAA will promote tumor growth. The studies indicate that important role in pathogenesis of alterations in BCAA metabolism play: i skeletal muscle as initial site of BCAA catabolism accompanied by the release of GLN, ALA, and BCKA to the blood; ii activity of BCKD in muscles and liver, and iii amination of BCKA to corresponding BCAA, especially by nitrogen of ALA and GLN released from muscles. Here are examples of importance of these metabolic steps:. ad i Because the muscle is the initial site of BCAA catabolism, marked rise of BCAA is observed after a meal while the rise of other amino acids is small. Enhanced consumption of the BCAA for ammonia detoxification to GLN in muscles is the main cause of the decrease of the BCAA in hyperammonemic conditions liver cirrhosis, UCD. Increased production of GLN after BCAA intake in muscles may lead to enhanced production of ammonia in enterocytes and kidneys with deleterious effect in subjects with liver disease. ad ii Decreased BCKD activity is the main cause of increased BCAA and BCKA levels in MSUD and may play a role in increased BCAA levels in obesity and type 2 diabetes. Increased BCKD activity is responsible for the decrease of BCAAs in CRF and enhanced oxidation of BCAAs during exercise and in various hypermetabolic conditions burn, sepsis, trauma, cancer. ad iii BCKA amination partially explains the increased BCAA concentrations during brief starvation and in type 1 diabetes, and is the basis of rationale to use BCKA-enriched supplements in CRF therapy. Although amino acid concentrations in the plasma pool are poor indicators of their requirements, it may be suggested that under conditions of good understanding of the BCAA metabolism in specific disorder, the BCAA levels would conceptually be an acceptable argument for their supplementation. It may be supposed that:. Together with requirements to decrease protein content in a diet, increased oxidation and low BCAA levels are a clear rationale to use the BCAA together with other essential amino acids and their ketoanalogues in CRF therapy. Although BCAA decrease in blood plasma is a rationale to use the BCAA supplements in patients with liver cirrhosis and UCD, therapeutic strategies are needed to avoid detrimental effects of BCAA supplementation on ammonia production. Further studies are necessary to conclude the question of the effects of BCAA supplementation in burn, trauma, sepsis, cancer, and exercise. A very small number of clinical studies have reported the effects of BCAA supplementation in relation to amino acid concentrations in blood and tissues. In conclusion, alterations in BCAA metabolism are common in a number of disease states and the BCAA have therapeutic potential due to their proven protein anabolic effects. However, many controversies about the use of BCAAs in clinical practice still exist, and careful studies are needed to elucidate the effectiveness of BCAAs in most indications. Chen L, Chen Y, Wang X, Li H, Zhang H, Gong J, Shen S, Yin W, Hu H. Efficacy and safety of oral branched-chain amino acid supplementation in patients undergoing interventions for hepatocellular carcinoma: a meta-analysis. Nutr J. Article PubMed PubMed Central CAS Google Scholar. Bifari F, Nisoli E. Branched-chain amino acids differently modulate catabolic and anabolic states in mammals: a pharmacological point of view. Br J Pharmacol. Article CAS PubMed Google Scholar. Harper AE, Miller RH, Block KP. Branched-chain amino acid metabolism. Annu Rev Nutr. Holecek M. Leucine metabolism in fasted and tumor necrosis factor-treated rats. Clin Nutr. Holecek M, Sprongl L, Skopec F, Andrýs C, Pecka M. Leucine metabolism in TNF-α- and endotoxin-treated rats: contribution of hepatic tissue Am J Phys ; E—E Swain LM, Shiota T, Walser M. Utilization for protein synthesis of leucine and valine compared with their keto analogues. Am J Clin Nutr. Holeček M, Šprongl L, Tichý M, Pecka M. Leucine metabolism in rat liver after a bolus injection of endotoxin. Article PubMed Google Scholar. Holecek M, Rysava R, Safranek R, Kadlcikova J, Sprongl L. Acute effects of decreased glutamine supply on protein and amino acid metabolism in hepatic tissue: a study using isolated perfused rat liver. Adibi SA. Influence of dietary deprivations on plasma concentration of free amino acids of man. J Appl Physiol. Holeček M, Mičuda S. Amino acid concentrations and protein metabolism of two types of rat skeletal muscle in postprandial state and after brief starvation. Physiol Res. PubMed Google Scholar. The BCAA-BCKA cycle: its relation to alanine and glutamine synthesis and protein balance. Nair KS, Short KR. Hormonal and signaling role of branched-chain amino acids. J Nutr. Floyd JC Jr, Fajans SS, Conn JW, Knopf RF, Rull J. Stimulation of insulin secretion by amino acids. J Clin Invest. Article CAS PubMed PubMed Central Google Scholar. Tischler ME, Desautels M, Goldberg AL. Does leucine, leucyl-tRNA, or some metabolite of leucine regulate protein synthesis and degradation in skeletal and cardiac muscle? J Biol Chem. CAS PubMed Google Scholar. Mitch WE, Walser M, Sapir DG. Nitrogen sparing induced by leucine compared with that induced by its keto analogue, alpha-ketoisocaproate, in fasting obese man. Sapir DG, Stewart PM, Walser M, Moreadith C, Moyer ED, Imbembo AL, et al. Effects of alpha-ketoisocaproate and of leucine on nitrogen metabolism in postoperative patients. Holeček M. Beta-hydroxy-beta-methylbutyrate supplementation and skeletal muscle in healthy and muscle-wasting conditions. J Cachexia Sarcopenia Muscle. Article PubMed PubMed Central Google Scholar. Fischer JE, Funovics JM, Aguirre A, James JH, Keane JM, Wesdorp RI, et al. The role of plasma amino acids in hepatic encephalopathy. Pedroso JA, Zampieri TT, Donato J. Reviewing the effects of L-leucine supplementation in the regulation of food intake, energy balance, and glucose homeostasis. Nishitani S, Takehana K, Fujitani S, Sonaka I. Branched-chain amino acids improve glucose metabolism in rats with liver cirrhosis. Am J Physiol Gastrointest Liver Physiol. Zhang S, Zeng X, Ren M, Mao X, Qiao S. Novel metabolic and physiological functions of branched chain amino acids: a review. J Anim Sci Biotechnol. Um SH, D'Alessio D, Thomas G. Nutrient overload, insulin resistance, and ribosomal protein S6 kinase 1, S6K1. Cell Metab. Tremblay F, Lavigne C, Jacques H, Marette A. Role of dietary proteins and amino acids in the pathogenesis of insulin resistance. White PJ, Lapworth AL, An J, Wang L, McGarrah RW, Stevens RD, et al. Branched-chain amino acid restriction in Zucker-fatty rats improves muscle insulin sensitivity by enhancing efficiency of fatty acid oxidation and acyl-glycine export. Mol Metab. Manchester KL. Oxidation of amino acids by isolated rat diaphragm and the influence of insulin. Biochim Biophys Acta. Holecek M, Siman P, Vodenicarovova M, Kandar R. Alterations in protein and amino acid metabolism in rats fed a branched-chain amino acid- or leucine-enriched diet during postprandial and postabsorptive states. Nutr Metab Lond. Article CAS Google Scholar. Metabolism of branched-chain amino acids in altered nutrition. Schauder P, Herbertz L, Langenbeck U. Serum branched chain amino and keto acid response to fasting in humans. Fryburg DA, Barrett EJ, Louard RJ, Gelfand RA. Effect of starvation on human muscle protein metabolism and its response to insulin. Am J Phys. CAS Google Scholar. Holecek M, Sprongl L, Tilser I. Metabolism of branched-chain amino acids in starved rats: the role of hepatic tissue. Adibi SA, Peterson JA, Krzysik BA. Modulation of leucine transaminase activity by dietary means. Sketcher RD, Fern EB, James WP. The adaptation in muscle oxidation of leucine to dietary protein and energy intake. Br J Nutr. Effect of starvation on branched-chain alpha-keto acid dehydrogenase activity in rat heart and skeletal muscle. Grimble RF, Whitehead RG. Changes in the concentration of specific amino acids in the serum of experimentally malnourished pigs. Holt LE, Snyderman SE, Norton PM, Roitman E, Finch J. The plasma aminogram in kwashiorkor. Reeds PJ. The catabolism of valine in the malnourished rat. Studies in vivo and in vitro with different labelled forms of valine. Wahren J, Felig P, Hagenfeldt L. Effect of protein ingestion on splanchnic and leg metabolism in normal man and in patients with diabetes mellitus. Holecek M, Kovarik M. Alterations in protein metabolism and amino acid concentrations in rats fed by a high-protein casein-enriched diet - effect of starvation. Food Chem Toxicol. Watford M. Lowered concentrations of branched-chain amino acids result in impaired growth and neurological problems: insights from a branched-chain alpha-keto acid dehydrogenase complex kinase-deficient mouse model. Nutr Rev. Anthony TG, Reiter AK, Anthony JC, Kimball SR, Jefferson LS. Deficiency of dietary EAA preferentially inhibits mRNA translation of ribosomal proteins in liver of meal-fed rats. Am J Physiol Endocrinol Metab. Blomstrand E. Amino acids and central fatigue. Amino Acids. Dasarathy S, Hatzoglou M. Hyperammonemia and proteostasis in cirrhosis. Curr Opin Clin Nutr Metab Care. Leweling H, Breitkreutz R, Behne F, Staedt U, Striebel JP, Holm E. Hyperammonemia-induced depletion of glutamate and branched-chain amino acids in muscle and plasma. J Hepatol. Holeček M, Šprongl L, Tichý M. Effect of hyperammonemia on leucine and protein metabolism in rats. Holecek M, Kandar R, Sispera L, Kovarik M. Acute hyperammonemia activates branched-chain amino acid catabolism and decreases their extracellular concentrations: different sensitivity of red and white muscle. Holeček M, Mráz J, Tilšer I. Plasma amino acids in four models of experimental liver injury in rats. Davis JM, Alderson NL, Welsh RS. Serotonin and central nervous system fatigue: nutritional considerations. Three targets of branched-chain amino acid supplementation in the treatment of liver disease. Holecek M, Simek J, Palicka V, Zadák Z. Effect of glucose and branched chain amino acid BCAA infusion on onset of liver regeneration and plasma amino acid pattern in partially hepatectomized rats. Als-Nielsen B, Koretz RL, Kjaergard LL, Gluud C. Branched-chain amino acids for hepatic encephalopathy. Cochrane Database Syst Rev. Google Scholar. Gluud LL, Dam G, Les I, Córdoba J, Marchesini G, Borre M, et al. Branched-chain amino acids for people with hepatic encephalopathy. Branched-chain amino acid supplementation in treatment of liver cirrhosis: updated views on how to attenuate their harmful effects on cataplerosis and ammonia formation. Article PubMed CAS Google Scholar. Rodney S, Boneh A. Amino acid profiles in patients with urea cycle disorders at admission to hospital due to metabolic decompensation. JIMD Rep. Evidence of a vicious cycle in glutamine synthesis and breakdown in pathogenesis of hepatic encephalopathy-therapeutic perspectives. In healthy individuals with normal mobility, dynamic balance between protein degradation and synthesis orchestrates skeletal muscle protein maintenance. In the postabsortive i. In the postprandial state, synthesis exceeds degradation, since intake of some nutrients, such as proteins and carbohydrates, stimulates muscle protein synthesis and insulin release, suppressing degradation. Rennie MJ, Wackerhage H, Spangenburg EE, Booth FW. Control of the size of the human muscle mass. Annu Rev Physiol. Therefore, muscle hypertrophy requires a positive net protein balance i. Physical exercise and nutrient availability are the major drivers of muscle protein synthesis in adult individuals. Atherton PJ, Smith K. Muscle protein synthesis in response to nutrition and exercise. J Physiol. McGlory C, Van Vliet S, Stokes T, Mittendorfer B, Phillips SM. The impact of exercise and nutrition on the regulation of skeletal muscle mass. The anabolic effects of nutrients are boosted primarily by transfer and incorporation of AA obtained through the diet into skeletal muscle proteins. These effects are particularly associated with EAA. Smith K, Barua JM, Watt PW, Scrimgeour CM, Rennie MJ. Flooding with L-[C]leucine stimulates human muscle protein incorporation of continuously infused L-[C] valine. Am J Physiol. The full range of EAA and the 11 NEAA must be present in proper amounts for muscle protein synthesis. Therefore, muscle protein synthesis is limited by lack or low availability of any of the EAA, whereas lack of NEAA can be offset by increased de novo synthesis. In the postprandial state, within approximately 30 to 45 minutes of consumption of a protein-rich meal average time required for digestion, absorption and transport of AA to the systemic circulation , EAA availability increases and muscle protein synthesis rates exceed muscle protein degradation rates, inducing an anabolic state that peaks between 1. Atherton PJ, Etheridge T, Watt PW, Wilkinson D, Selby A, Rankin D, et al. Muscle full effect after oral protein: time-dependent concordance and discordance between human muscle protein synthesis and mTORC1 signaling. Am J Clin Nutr. Aminoacidemia-induced muscle protein synthesis is transient. In the postabsortive state i. In these circumstances, plasma EAA level maintenance and hence protein turnover relies on protein breakdown in skeletal muscles, the major body protein reservoir. Cahill GF Jr, Aoki TT. Starvation and body nitrogen. Trans Am Clin Climatol Assoc. The impact of factors such as protein amount and quality, protein intake distribution throughout day and physical exercise on the balance between protein degradation and synthesis must be emphasized. Biolo G, Gastaldelli A, Zhang XJ, Wolfe RR. Protein synthesis and breakdown in skin and muscle: a leg model of amino acid kinetics. Hence, muscle protein degradation always exceeds muscle protein synthesis in the postabsorptive state due to muscle protein catabolism and catabolic conditions determined by lack of dietary EAA intake. This is thought to be partly due to the AA leucine, as leucine alone is able to induce a muscle protein synthesis response via activation of the mechanistic target of rapamycin complex 1 mTORC1 , a vital cell growth regulator. Crozier SJ, Kimball SR, Emmert SW, Anthony JC, Jefferson LS. Oral leucine administration stimulates protein synthesis in rat skeletal muscle. J Nutr. Louard et al. Louard RJ, Barrett EJ, Gelfand RA. Effect of infused branched-chain amino acids on muscle and whole-body amino acid metabolism in man. Clin Sci Lond. Overnight branched-chain amino acid infusion causes sustained suppression of muscle proteolysis. tested this hypothesis in humans submitted to overnight fasting. In their study, the effects of intravenous BCAA infusion for 3 and 16 hours on muscle protein synthesis and degradation were investigated. Both infusion protocols increased plasma BCAA levels, whereas plasma levels of other EAA decreased. The fact that muscle protein degradation was mitigated by isolated intake of three out of 11 EAA, but remained higher than muscle protein synthesis, suggested the catabolic state prevailed in order to release other EAA required for synthesis. It seems therefore plausible to assume BCAA intake alone cannot create an anabolic state leading to muscle protein synthesis in excess of degradation, at least in theory. Another important question is whether anabolic pathway activation and increased muscle protein synthesis are separate events. Rising insulin levels are a potent anabolic signaling pathway activator, but are not associated with enhanced muscle protein synthesis in the absence of EAA. Greenhaff PL, Karagounis LG, Peirce N, Simpson EJ, Hazell M, Layfield R, et al. Disassociation between the effects of amino acids and insulin on signaling, ubiquitin ligases, and protein turnover in human muscle. Am J Physiol Endocrinol Metab. In contrast, intake of small amounts 3g of EAA stimulates synthesis regardless of anabolic signaling pathway activation. Bukhari SS, Phillips BE, Wilkinson DJ, Limb MC, Rankin D, Mitchell WK, et al. Intake of low-dose leucine-rich essential amino acids stimulates muscle anabolism equivalently to bolus whey protein in older women at rest and after exercise. Should muscle protein synthesis be limited by activation of factors triggering pathway initiating, increases in plasma EAA levels, however small, would not have this effect. These findings demonstrated muscle protein synthesis in humans is limited by availability of the full range of EAA rather than anabolic signaling pathway activation. Combination of physical exercise resistance physical exercise in particular with protein intake maximizes and prolongs muscle protein synthesis stimulation for approximately 24 hours post-workout due to increased tissue sensitivity to anabolic properties of AA. Burd NA, West DW, Moore DR, Atherton PJ, Staples AW, Prior T, et al. |
Branched-chain amino acids activate key enzymes in protein synthesis after physical exercise | Scaini G, Jeremias IC, Morais MO, Borges GD, Munhoz BP, Leffa DD, et al. Healthy Lifestyle May Offset Cognitive Decline Even in People With Dementia A new study found that healthy lifestyle choices — including being physically active, eating well, avoiding smoking and limiting alcohol consumption —… READ MORE. Nutrients, 8 7 , Tulp, O. ALA is the main gluconeogenic amino acid, and GLN availability is essential for immune system, glutathione production, maintenance of acid-base balance by the kidneys, and expression of heat shock proteins. |
Video
The Truth About BCAAs and EAAs - Are They Worth It?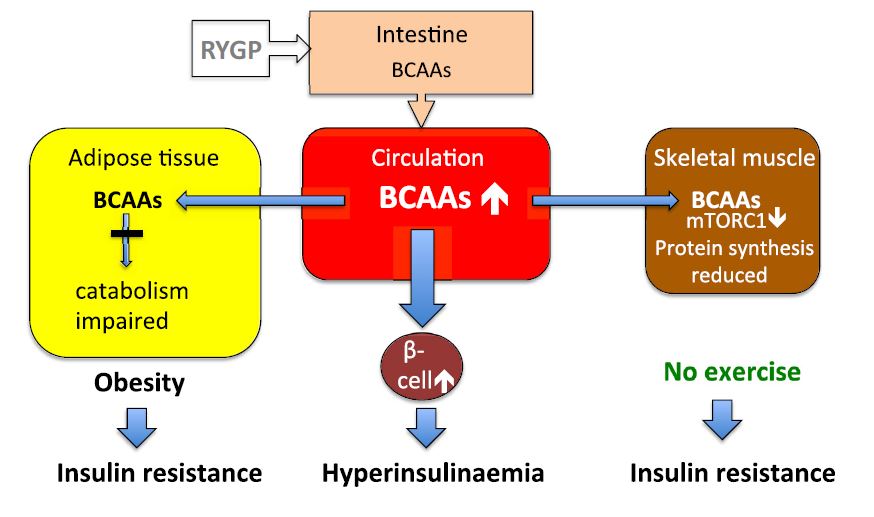
das Unvergleichliche Thema, mir ist es sehr interessant:)
Ich kann die Verbannung auf die Webseite mit den Informationen zum Sie interessierenden Thema suchen.