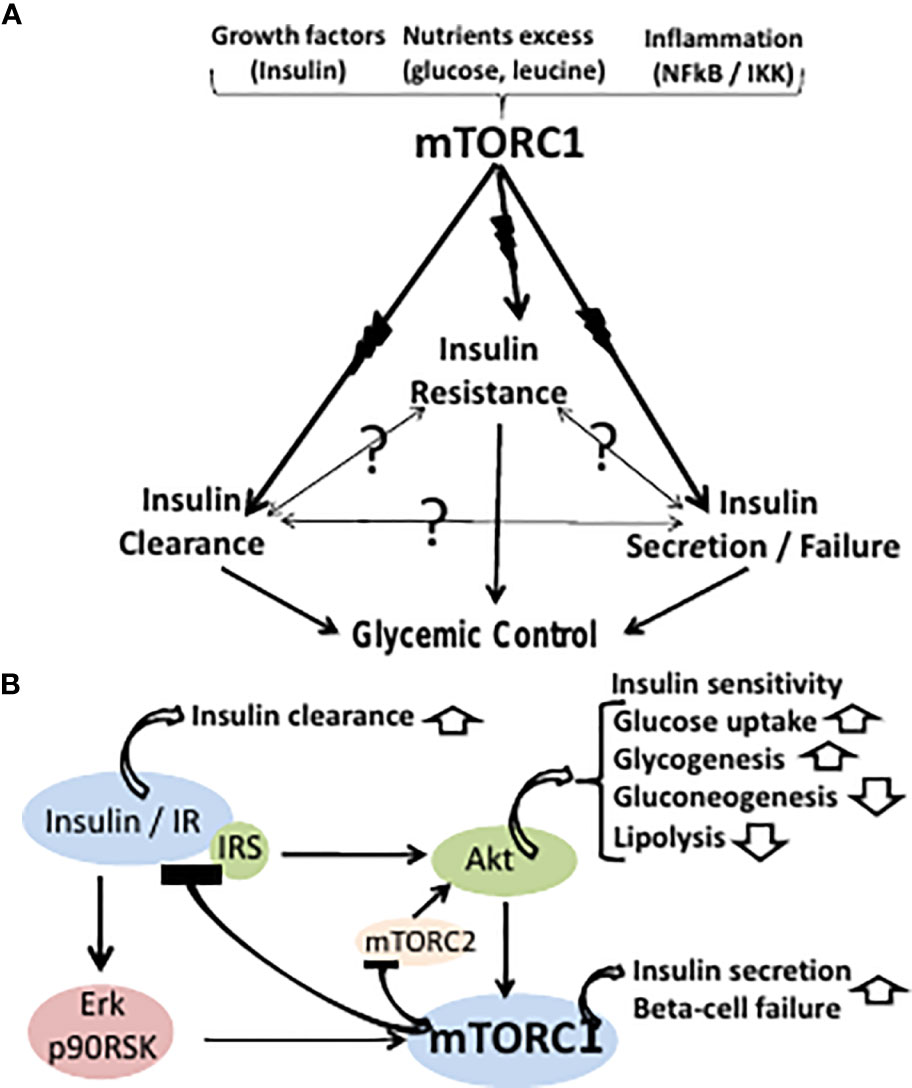
Insulin sensitivity and glucose metabolism -
Two hours later, muscle, liver and fat tissues were removed and single-cell suspensions from each of those tissues were tested by fluorescence activated cell sorting FACS for the presence of 2-NBDG. We produced a model for type 2 diabetes by feeding a high-fat diet TD. Thirteen weeks later, the mice were injected i.
with µg of 2-NBDG. Two hours after injection, muscle and liver tissues were removed and analyzed for glucose uptake as described above. This value was obtained by all mice after 13 weeks on the diet. Prior to euthanasia of mice, fasting serum insulin levels were determined by ELISA according to the manufacture's instructions.
To test the hypothesis that Ras inhibition results in increased uptake of glucose in muscle cells, we induced C2C12 cell differentiation into myotubes see Methods and transfected the differentiated muscle cells with DN-GFP-labeled Ras or a GFP-labeled control plasmid.
We then induced insulin resistance in all cells by addition of palmitate see Methods and assayed their ability to absorb fluorescent glucose. In line with previous reports [35] , palmitate reduced glucose uptake compared to BSA-treated control cells not shown.
These results suggest that active Ras inhibition may upregulate glucose absorption. Insulin-resistant C2C12 myotubes were transfected with DN-Ras-GFP or GFP plasmid pGFP and fluorescent glucose uptake was measured by flow cytometry.
Statistical analysis of the results is presented as means ± S. IκB, NF-κB and tubulin expression in the DN-Ras transfected or GFP-transfected myotubes were assayed by western blotting, as described in Material and Methods. Densitometry of IκB and NF-κB expression.
The nuclear transcription factor NF-κB and its inhibitor IκB have been shown to participate in the induction of insulin resistance by palmitate. To study the effect of Ras inhibition on NF-κB and IκB in the insulin-resistant C2C12 cells, we assayed IκB and NF-κB in these cells following DN-Ras transfection.
To verify the effect of Ras inhibition on glucose uptake, we used F-FTS, a small molecule that interferes with anchorage of Ras to the membrane and hence inhibits Ras function [29] , [31]. First, we examined whether F-FTS mimics the observed effect of DN-Ras on glucose uptake in C2C12 myotubes.
In line with this effect of F-FTS on glucose uptake was the finding that expression of the mRNA glucose transporter 4 glut-4 was induced by F-FTS. Insulin-resistant C2C12 myotubes were incubated with or without F-FTS 50 µM , and were then assayed for their ability to absorb fluorescent glucose.
Densitometry of Glut4 is shown. I K B, NF-κB, p-I K B and tubulin were assayed by western blotting as described in Methods. Densitometry of IκB, p-I K B and NF-κB expression. These findings are consistent with the results obtained by treatment with DN-Ras. To determine whether the enhanced glucose uptake resulting from treatment with DN-Ras in vitro Figs.
with DN-GFP-Ras or with pGFP, as described in Methods. Representative histograms of glucose uptake are presented for each tissue. Testing of the above tissues for Ras-GTP expression revealed a significant decrease, concomitantly with the effects on glucose uptake Fig.
No significant differences in glucose uptake were observed in the fat tissues data not shown. IκB, NF-κB and tubulin in the tissues were assayed by western blotting, as described in Methods.
No differences were found in the liver tissue data not shown. Having shown that Ras inhibition by F-FTS mimics the effect of DN-Ras on glucose uptake both in vitro and in vivo , we next examined the effect of long-term treatment with F-FTS or FTS on the development of hyperglycemia in an experimental model of type 2 diabetes.
To examine how the effectiveness of the drugs was influenced by the route of administration, we treated the mice either intraperitoneally i. or per os p. Similar results were observed when mice were treated p. Kaplan-Meier plots of mean incidence of diabetes in each group.
Kaplan-Meier curves record the mean incidence of diabetes in each group. All treated animals were monitored for weight gain while being fed a high-fat diet. Kaplan-Meier curves record the mean percentage of weight gain in each group. As expected, the high-fat diet caused a significant increase in body weight in all treated groups [36].
No significant differences in weight gain were observed between the i. F-FTS-treated and PBS-treated groups or between the p. o FTS-treated, F-FTS-treated and CMC-treated groups Fig.
We also assayed circulating insulin in the different groups of mice. After i. treatment, the levels of circulating insulin were significantly decreased in the F-FTS-treated group 4. In the orally treated mice, insulin concentrations in the F-FTS-treated and the FTS-treated groups were also significantly reduced 7.
Activated Ras plays an important role in modulating a number of signaling molecules that trigger cell proliferation, differentiation, and survival [37] , [38]. These observations are in line with several studies showing that Ras inhibition attenuates inflammatory responses in experimental models [25] , [31] , [39] , [40].
Resistance to insulin, resulting in decreased glucose uptake, is a major factor contributing to the development of type 2 diabetes [41]. The mechanisms responsible for inducing resistance to insulin are not completely understood, but accumulating data point to a robust association between insulin resistance and inflammation.
Obesity promotes insulin resistance by resulting in a state of chronic inflammation that involves production of proinflammatory cytokines TNF-α, IL-6 , an increase in the number of macrophages, and activation of a complex cascade of signaling events in muscle, fat and liver tissues [6] , [35] , [42].
Consistent with these findings, we showed here for the first time that inhibition of Ras by DN-Ras or F-FTS, promoted anti-inflammatory response in a muscle cell line and in mouse tissues. This study is the first to show a clear association between Ras signaling and insulin resistance in muscle, fat and liver.
We found that inhibition of Ras activation by transfection with DN-Ras or by treatment with the small-molecule Ras inhibitor F-FTS induced glucose uptake in vitro , indicating higher insulin sensitivity.
In addition, we demonstrated that inhibition of Ras in vivo by hydrodynamic injection of DN-Ras or by daily treatment with F-FTS in an experimental murine model of HF-induced diabetes resulted in similar findings of increased uptake of fluorescently labeled glucose by muscle, fat and liver tissues.
To characterize the signaling pathway by which Ras inhibition promotes insulin sensitivity, we studied the expression of key regulators known to participate in insulin-signaling pathways. IκB kinase IKK phosphorylates certain serine residues on insulin receptor kinase 1 IRS-1 , leading to impairment of insulin signal transduction.
In addition, the IKK signaling pathway is upregulated and activated, both in insulin-resistant humans and in rodent skeletal muscles [43]. Increased expression of IKK results in inhibition of IκB and activation of NF-κB ; the latter subsequently transcriptionally activates a set of inflammatory pathway genes that induce resistance to insulin see scheme, Fig.
Free fatty acids FFAs lead to activation of IKK, the inhibitor of IκB kinase. IKK affects insulin sensitivity and glucose uptake via two distinct pathways.
First, IKK phosphorylates insulin receptor substrate 1 IRS-1 , resulting in inactivation of insulin signaling through attenuated transcription of glucose transporter 4 Glut4. Ras inhibition by F-FTS demonstrates enhanced Glut4 transcription, hence also heightened glucose uptake.
Second, IKK phosphorylates the inhibitor of κB IκB , causing it to become detached from nuclear factor κB NF-κB. NF-κB enters the nucleus and induces transcription of proinflammatory cytokines such as IL-6 and TNF-α.
These cytokines leads to deterioration of insulin resistance. Ras inhibition by DN-Ras or by F-FTS augments IκB expression, thereby attenuating the proinflammatory response and enhancing insulin sensitivity and glucose uptake. We found that Ras inhibition led to an increase in IκB, which inhibited the expression of NF-κB both in vitro and in vivo Figs.
The improvement in glucose uptake in liver tissue of the F-FTS treated animals was not correlated with increased expression of IκB Fig 4. This finding could result from the long period 13 weeks of treatment that may influence the duration of the increased IκB expression.
Overall, the observation that insulin resistance was attenuated by Ras inhibition in association with regulation of IκB and NF-κB provides a possible link between Ras, inflammation, and negative regulation of insulin signaling.
The most downstream factor in the insulin cascade is Glut4, an essential transporter responsible for translocation of insulin-regulated glucose into the cell [45]. We therefore examined the effect of Ras inhibition on Glut4 mRNA levels in insulin-resistant C2C12 myotubes treated with F-FTS.
We found an increase in Glut4 mRNA levels after F-FTS treatment. These results suggested that the higher sensitivity to insulin was attributable to Ras inhibition, which may be related to the increase in expression of Glut4 transporter in the plasma membrane and the subsequent potentiated influx of glucose into the cell see scheme, Figure 6.
Taken together, our results suggest dual affects of Ras on insulin sensitivity and glucose uptake via two distinct pathways Figure 6.
Previous studies have shown that both FTS and the small synthetic molecule F-FTS act primarily by inhibiting active Ras proteins and are mimicked by dominant negative Ras [30] , [46].
Therefore, mice fed a high-fat diet and concomitantly treated with F-FTS may serve as an appropriate in vivo model for examining the effect of Ras inhibition on an experimental model of type 2 diabetes. Our results showed that treatment with either FTS or F-FTS significantly attenuated the incidence of hyperglycemia in this model.
The potential contribution of Ras-mediated insulin sensitization in this in vivo model is supported by the finding that circulating insulin levels were decreased in the FTS-treated mice Fig. The observed decrease of insulin level most likely resulted from the increased uptake of glucose into the tissues but could also be caused by a direct effect on the pancreas.
Further studies should be performed to clarify the cause of the decrease in serum insulin levels. Taken together, the results of this study showed that inhibition of Ras signaling enhances both insulin sensitivity and glucose uptake in vitro and in vivo.
These observations were corroborated by the beneficial effects of Ras inhibition that resulted in attenuation of hyperglycemia in a conventional type 2 diabetes model. It should be noted, however, that Ras inhibition may modify inflammatory responses in other tissues as well.
These findings pave the way for a novel approach to the potential treatment of insulin resistance and type 2 diabetes.
Conceived and designed the experiments: AM EA JG YK. Performed the experiments: AM EA. Analyzed the data: AM EA JG YK. Wrote the paper: AM EA JG YK. Browse Subject Areas? Click through the PLOS taxonomy to find articles in your field. Article Authors Metrics Comments Media Coverage Reader Comments Figures.
Abstract Background Reduced glucose uptake due to insulin resistance is a pivotal mechanism in the pathogenesis of type 2 diabetes. Methods and Findings The effect of Ras inhibition on glucose uptake was examined both in vitro and in vivo. Conclusions Inhibition of Ras apparently induces a state of heightened insulin sensitization both in vitro and in vivo.
Introduction Insulin resistance is defined as impaired sensitivity to insulin in its main target organs muscle, liver and adipose tissues , and is considered a hallmark of type 2 diabetes [1]. Materials and Methods Induction of insulin resistance in cell culture Mouse C2C12 myoblasts generously provided by Prof.
Transfection with dominant-negative Ras To block Ras we transfected differentiated C2C12 cells with 2 7g of green fluorescent protein plasmid pGFP or dominant-negative DN GFP-Ras 17N , using lipofectamine reagent according to manufacture's instructions Invitrogen, Carlsbad, CA, USA.
Western blotting To examine the impact of Ras inhibition on IκB and NF-κB, we performed Western immunoblotting with specific antibodies.
Glut4 expression determined by reverse transcription—PCR RNA was extracted from 10 6 C2C12 muscle cells using an RNeasy Mini Kit Qiagen, Hilden, Germany according to the manufacturer's instructions.
In vivo studies The study was approved by the Institutional Ethics Committee at the Tel Aviv Sourasky Medical Center, Tel Aviv, Israel Approval ID is L Download: PPT. Figure 1. Figure 2. Ras inhibition by hydrodynamic injection of DN-Ras induces glucose uptake in vivo To determine whether the enhanced glucose uptake resulting from treatment with DN-Ras in vitro Figs.
Figure 3. Ras inhibition in vivo increases muscle, fat and liver glucose uptake. Figure 4. Ras inhibition by F-FTS attenuates type 2 diabetes in vivo and reduces circulating insulin levels Having shown that Ras inhibition by F-FTS mimics the effect of DN-Ras on glucose uptake both in vitro and in vivo , we next examined the effect of long-term treatment with F-FTS or FTS on the development of hyperglycemia in an experimental model of type 2 diabetes.
Figure 5. Ras inhibition in HF-induced diabetic mice reduces diabetes incidence and increases the concentration of circulating insulin. Discussion Activated Ras plays an important role in modulating a number of signaling molecules that trigger cell proliferation, differentiation, and survival [37] , [38].
Figure 6. Proposed mechanism explaining the effect of Ras on insulin sensitivity. Acknowledgments We thank S. Smith for editorial assistance. Author Contributions Conceived and designed the experiments: AM EA JG YK.
References 1. Reaven GM Banting lecture Role of insulin resistance in human disease. Diabetes — View Article Google Scholar 2.
Defronzo RA Banting Lecture. From the triumvirate to the ominous octet: a new paradigm for the treatment of type 2 diabetes mellitus. View Article Google Scholar 3.
Jager J, Gremeaux T, Cormont M, Le Marchand-Brustel Y, Tanti JF Interleukin-1beta-induced insulin resistance in adipocytes through down-regulation of insulin receptor substrate-1 expression. Endocrinology — View Article Google Scholar 4.
Hotamisligil GS, Arner P, Caro JF, Atkinson RL, Spiegelman BM Increased adipose tissue expression of tumor necrosis factor-alpha in human obesity and insulin resistance. Journal of Clinical Investigations — View Article Google Scholar 5. Weigert C, Hennige AM, Lehmann R, Brodbeck K, Baumgartner F, et al.
Journal of Biological Chemistry — View Article Google Scholar 6. Uysal KT, Wiesbrock SM, Marino MW, Hotamisligil GS Protection from obesity-induced insulin resistance in mice lacking TNF-alpha function.
Nature — View Article Google Scholar 7. Franckhauser S, Elias I, Rotter Sopasakis V, Ferre T, Nagaev I, et al. Diabetologia — Insulin resistance and bronze diabetes. Laakso, M. Insulin resistance and hyperglycaemia in cardiovascular disease development.
Article CAS PubMed Google Scholar. Bugianesi, E. Insulin resistance in nonalcoholic fatty liver disease. Saklayen, M. The global epidemic of the metabolic syndrome. Diamanti-Kandarakis, E. Insulin resistance and the polycystic ovary syndrome revisited: an update on mechanisms and implications.
Stenvers, D. Circadian clocks and insulin resistance. Article PubMed CAS Google Scholar. Freeman AM, Pennings N. Insulin Resistance. In: StatPearls Internet. Treasure Island FL : StatPearls Publishing. PMID: American Diabetes Association. Prevention or delay of type 2 diabetes: standards of medical care in diabetes Diabetes Care 44 , S34—S39 Pharmacologic approaches to glycemic treatment: Standards of Medical Care in Diabetes Diabetes Care 44 , S—S Weiss, M.
Insulin biosynthesis, secretion, structure, and structure-activity relationships. In: Feingold KR, Anawalt B, Boyce A, et al. South Dartmouth MA : MDText.
com, Inc. Sanger, F. Chemistry of insulin. Science , — Katsoyannis, P. Synthesis of insulin. Lee, J. The insulin receptor: structure, function, and signaling. Pessin, J. Signaling pathways in insulin action: molecular targets of insulin resistance. Invest , — Haeusler, R. Biochemical and cellular properties of insulin receptor signalling.
Cell Biol. White, M. Mechanisms of insulin action. In Atlas of diabetes pp. Springer, Boston, MA Newgard, C. Organizing glucose disposal: emerging roles of the glycogen targeting subunits of protein phosphatase Diabetes 49 , — Beurel, E. Glycogen synthase kinase-3 GSK3 : regulation, actions, and diseases.
Article CAS Google Scholar. Dong, X. Inactivation of hepatic Foxo1 by insulin signaling is required for adaptive nutrient homeostasis and endocrine growth regulation. Cell Metab. Puigserver, P. Insulin-regulated hepatic gluconeogenesis through FOXO1—PGC-1α interaction.
Nature , — Vander Haar, E. Garami, A. Cell 11 , — Laplante, M. mTORC1 activates SREBP-1c and uncouples lipogenesis from gluconeogenesis.
USA , — Han, Y. Post-translational regulation of lipogenesis via AMPK-dependent phosphorylation of insulin-induced gene.
Calejman, C. mTORC2-AKT signaling to ATP-citrate lyase drives brown adipogenesis and de novo lipogenesis. Xia, W. Loss of ABHD15 impairs the anti-lipolytic action of insulin by altering PDE3B stability and contributes to insulin resistance. Cell Rep. James, D.
The aetiology and molecular landscape of insulin resistance. Tam, C. Defining insulin resistance from hyperinsulinemic-euglycemic clamps. Diabetes care 35 , — Samuel, V.
Mechanisms for insulin resistance: common threads and missing links. Cell , — Ye, J. Mechanisms of insulin resistance in obesity. Front Med. Article PubMed PubMed Central Google Scholar.
Yaribeygi, H. Insulin resistance: Review of the underlying molecular mechanisms. Cell Physiol. De Meyts, P. The insulin receptor: a prototype for dimeric, allosteric membrane receptors? Trends Biochem Sci. Caro, J. Insulin receptor kinase in human skeletal muscle from obese subjects with and without noninsulin dependent diabetes.
Invest 79 , — Fröjdö, S. Alterations of insulin signaling in type 2 diabetes: a review of the current evidence from humans. Biochim Biophys. Acta , 83—92 Fisher, S. Michael, M. Loss of insulin signaling in hepatocytes leads to severe insulin resistance and progressive hepatic dysfunction.
Cell 6 , 87—97 Davis, R. The c-Jun NH2-terminal kinase promotes insulin resistance during association with insulin receptor substrate-1 and phosphorylation of Ser Article PubMed Google Scholar. Carvalho-Filho, M. Diabetes 54 , — Taniguchi, C. Critical nodes in signalling pathways: insights into insulin action.
Brachmann, S. Phosphoinositide 3-kinase catalytic subunit deletion and regulatory subunit deletion have opposite effects on insulin sensitivity in mice. Phosphatidylinositol 3-kinase activation is required for insulin stimulation of pp70 S6 kinase, DNA synthesis, and glucose transporter translocation.
CAS PubMed PubMed Central Google Scholar. Czech, M. Signaling mechanisms that regulate glucose transport. Luo, J. Loss of class IA PI3K signaling in muscle leads to impaired muscle growth, insulin response, and hyperlipidemia. Cong, L.
Physiological role of Akt in insulin-stimulated translocation of GLUT4 in transfected rat adipose cells. Xia, J. Targeted induction of ceramide degradation leads to improved systemic metabolism and reduced hepatic steatosis.
Le Marchand-Brustel, Y. Insulin receptor tyrosine kinase is defective in skeletal muscle of insulin-resistant obese mice. Brozinick, J. Defective signaling through Akt-2 and-3 but not Akt-1 in insulin-resistant human skeletal muscle: potential role in insulin resistance.
Diabetes 52 , — Kruszynska, Y. Fatty acid-induced insulin resistance: decreased muscle PI3K activation but unchanged Akt phosphorylation.
Choi, K. Molecular mechanism of insulin resistance in obesity and type 2 diabetes. Korean J. Intern Med. Kahn, B. Glucose transport: pivotal step in insulin action.
Diabetes 45 , — Dimitriadis, G. Insulin effects in muscle and adipose tissue. Diabetes Res Clin. Shepherd, P. Glucose transporters and insulin action-implications for insulin resistance and diabetes mellitus.
Li, J. Reduced glucose uptake precedes insulin signaling defects in adipocytes from heterozygous GLUT4 knockout mice. FASEB J. Klip, A.
Recruitment of GLUT-4 glucose transporters by insulin in diabetic rat skeletal muscle. Biochem Biophys. Res Commun. Etgen, G. Jr et al. Exercise training reverses insulin resistance in muscle by enhanced recruitment of GLUT-4 to the cell surface.
Ryder, J. Use of a novel impermeable biotinylated photolabeling reagent to assess insulin-and hypoxia-stimulated cell surface GLUT4 content in skeletal muscle from type 2 diabetic patients.
Garvey, W. Multiple defects in the adipocyte glucose transport system cause cellular insulin resistance in gestational diabetes: heterogeneity in the number and a novel abnormality in subcellular localization of GLUT4 glucose transporters. Diabetes 42 , — Chadt, A.
Deletion of both Rab-GTPase—activating proteins TBC14KO and TBC1D4 in mice eliminates insulin-and AICAR-stimulated glucose transport.
Diabetes 64 , — Chen, S. Tramunt, B. Sex differences in metabolic regulation and diabetes susceptibility. Diabetologia 63 , — Greenhill, C. Sex differences in insulin resistance. Qiu, J. Estradiol protects proopiomelanocortin neurons against insulin resistance. Endocrinology , — Zidon, T. Effects of ERβ and ERα on OVX-induced changes in adiposity and insulin resistance.
Ikeda, K. Functions of estrogen and estrogen receptor signaling on skeletal muscle. Steroid Biochem Mol. Gerdts, E. Sex differences in cardiometabolic disorders.
Chia, C. Age-related changes in glucose metabolism, hyperglycemia, and cardiovascular risk. Shi, H. Sex differences in obesity-related glucose intolerance and insulin resistance. Glucose Tolerance 4 , 37—66 Google Scholar.
Geer, E. Gender differences in insulin resistance, body composition, and energy balance. Christen, T. Sex differences in body fat distribution are related to sex differences in serum leptin and adiponectin. Peptides , 25—31 Palmisano, B. Sex differences in lipid and lipoprotein metabolism.
Kodama, K. Ethnic differences in the relationship between insulin sensitivity and insulin response: a systematic review and meta-analysis. Diabetes Care 36 , — Raygor, V. Diab Vasc. Sumner, A. Ethnic differences in the ability of triglyceride levels to identify insulin resistance.
Atherosclerosis , — Tan, V. Ethnic differences in insulin sensitivity and beta-cell function among Asian men. Diabetes 5 , e—e Ministry of Health Singapore MOHS. Potts, J. Sex and ethnic group differences in fat distribution in young United Kingdom South Asians and Europids.
Ehtisham, S. Ethnic differences in insulin resistance and body composition in United Kingdom adolescents.
Lear, S. Ethnic variation in fat and lean body mass and the association with insulin resistance. Mason, C. Dietary weight loss and exercise effects on insulin resistance in postmenopausal women. Med 41 , — Mikusova, V.
Insulin resistance and need for a lifestyle change to eliminate it. Listy , — orpeleijn, E. Metabolic flexibility in the development of insulin resistance and type 2 diabetes: effects of lifestyle.
Shigeta, H. Lifestyle, obesity, and insulin resistance. Diabetes Care 24 , Oosterman, J. The circadian clock, shift work, and tissue-specific insulin resistance. Endocrinology , bqaa McAuley, K. Intensive lifestyle changes are necessary to improve insulin sensitivity: a randomized controlled trial.
Diabetes care 25 , — Bergman, B. Novel and reversible mechanisms of smoking-induced insulin resistance in humans. Diabetes 61 , — Kan, C. A systematic review and meta-analysis of the association between depression and insulin resistance. Diabetes care 36 , — Sung, C. Role of vitamin D in insulin resistance.
Ardabili, H. Vitamin D supplementation has no effect on insulin resistance assessment in women with polycystic ovary syndrome and vitamin D deficiency. Pasieka, A. Impact of glucocorticoid excess on glucose tolerance: clinical and preclinical evidence.
Metabolites 6 , 24 Article PubMed Central CAS Google Scholar. Rizza, R. Cortisol-induced insulin resistance in man: impaired suppression of glucose production and stimulation of glucose utilization due to a postreceptor defect of insulin action.
Effects of growth hormone on insulin action in man: mechanisms of insulin resistance, impaired suppression of glucose production, and impaired stimulation of glucose utilization.
Diabetes 31 , — Barbour, L. A Cellular mechanisms for insulin resistance in normal pregnancy and gestational diabetes. Diabetes Care 30 , S—S Parichatikanond, W. Prolonged stimulation of β2-adrenergic receptor with β2-agonists impairs insulin actions in H9c2 cells.
Walli, R. Treatment with protease inhibitors associated with peripheral insulin resistance and impaired oral glucose tolerance in HIVinfected patients. AIDS 12 , F—F Murata, H. The mechanism of insulin resistance caused by HIV protease inhibitor therapy. Teff, K. Antipsychotic-induced insulin resistance and postprandial hormonal dysregulation independent of weight gain or psychiatric disease.
Diabetes 62 , — Bittencourt, M. Insulin therapy in insulin resistance: could it be part of a lethal pathway? Elbein, S. Heritability of pancreatic beta-cell function among nondiabetic members of Caucasian familial type 2 diabetic kindreds.
Shulman, G. Cellular mechanisms of insulin resistance. Knauf, C. Brain glucagon-like peptide-1 increases insulin secretion and muscle insulin resistance to favor hepatic glycogen storage. Petersen, M. Regulation of hepatic glucose metabolism in health and disease. Matsumoto, M.
Dual role of transcription factor FoxO1 in controlling hepatic insulin sensitivity and lipid metabolism. Shimomura, I. Cell 6 , 77—86 Petersen, K. Mechanism by which glucose and insulin inhibit net hepatic glycogenolysis in humans. Henriksen, E. Role of glycogen synthase kinase-3 in insulin resistance and type 2 diabetes.
Drug Targets 7 , — Karim, S. Hepatic expression and cellular distribution of the glucose transporter family. World J. Rencurel, F. Requirement of glucose metabolism for regulation of glucose transporter type 2 GLUT2 gene expression in liver.
Thorens, B. Diabetologia 58 , — Eberlé, D. SREBP transcription factors: master regulators of lipid homeostasis. Biochimie 86 , — Horton, J. SREBPs: activators of the complete program of cholesterol and fatty acid synthesis in the liver. Ferré, P. Hepatic steatosis: a role for de novo lipogenesis and the transcription factor SREBP-1c.
Diabetes Obes. Tobe, K. Dentin, R. Carbohydrate responsive element binding protein ChREBP and sterol regulatory element binding protein-1c SREBP-1c : two key regulators of glucose metabolism and lipid synthesis in liver.
Biochimie 87 , 81—86 Hepatic glucokinase is required for the synergistic action of ChREBP and SREBP-1c on glycolytic and lipogenic gene expression.
Herman, M. A novel ChREBP isoform in adipose tissue regulates systemic glucose metabolism. Iizuka, K. Deficiency of carbohydrate response element-binding protein ChREBP reduces lipogenesis as well as glycolysis.
Natl Acad. Jaworski, K. Regulation of triglyceride metabolism. Hormonal regulation of lipolysis in adipose tissue. Liver Physiol. Vaughan, M. Hormone-sensitive lipase and monoglyceride lipase activities in adipose tissue. Zmuda-Trzebiatowska, E. Role of PDE3B in insulin-induced glucose uptake, GLUT-4 translocation and lipogenesis in primary rat adipocytes.
Cell Signal 18 , — Choi, Y. Alterations in regulation of energy homeostasis in cyclic nucleotide phosphodiesterase 3B—null mice. Martinez-Botas, J. Genet 26 , — Tansey, J. Perilipin ablation results in a lean mouse with aberrant adipocyte lipolysis, enhanced leptin production, and resistance to diet-induced obesity.
USA 98 , — Mechanisms of Insulin Action and Insulin Resistance. Kimball, S. Regulation of protein synthesis by insulin. Pösö, A. Multifunctional control of amino acids of deprivation-induced proteolysis in liver. Role of leucine. Marshall, S. New insights into the metabolic regulation of insulin action and insulin resistance: role of glucose and amino acids.
Rudrappa, S. Human skeletal muscle disuse atrophy: effects on muscle protein synthesis, breakdown, and insulin resistance-a qualitative review. Front Physiol. Medeiros, C. Antuna-Puente, B. Adipokines: the missing link between insulin resistance and obesity. Diabetes Metab. Rabe, K. Adipokines and insulin resistance.
Adipokines mediate inflammation and insulin resistance. Lausanne 4 , 71 Li, S. Adiponectin levels and risk of type 2 diabetes: a systematic review and meta-analysis.
JAMA , — Hotta, K. Circulating concentrations of the adipocyte protein adiponectin are decreased in parallel with reduced insulin sensitivity during the progression to type 2 diabetes in rhesus monkeys.
Diabetes 50 , — Takahashi, M. Chemerin enhances insulin signaling and potentiates insulin-stimulated glucose uptake in 3T3-L1 adipocytes. FEBS Lett. Yamauchi, T. Targeted disruption of AdipoR1 and AdipoR2 causes abrogation of adiponectin binding and metabolic actions.
Li, L. Changes and relations of circulating visfatin, apelin, and resistin levels in normal, impaired glucose tolerance, and type 2 diabetic subjects. Diabetes , — Soriguer, F. Apelin levels are increased in morbidly obese subjects with type 2 diabetes mellitus. Yue, P.
Apelin is necessary for the maintenance of insulin sensitivity. American journal of physiology. Apelin decreases lipolysis via G q , G i , and AMPK-dependent mechanisms.
Endocrinology , 59—68 Segal, K. Relationship between insulin sensitivity and plasma leptin concentration in lean and obese men. Amitani, M. The role of leptin in the control of insulin-glucose axis. Front Neurosci. Article PubMed PubMed Central CAS Google Scholar.
Ceddia, R. Analysis of paradoxical observations on the association between leptin and insulin resistance. Covey, S. The pancreatic beta cell is a key site for mediating the effects of leptin on glucose homeostasis.
Seufert, J. Leptin effects on pancreatic beta-cell gene expression and function. Diabetes 53 , S—S Myers, M.
Mechanisms of leptin action and leptin resistance. Burguera, B. Obesity is associated with a decreased leptin transport across the blood-brain barrier in rats. Gainsford, T. Leptin can induce proliferation, differentiation, and functional activation of hemopoietic cells.
USA 93 , — Scherer, P. Adipose tissue: from lipid storage compartment to endocrine organ. Diabetes 55 , — Saponaro, C. The subtle balance between lipolysis and lipogenesis: a critical point in metabolic homeostasis. Nutrients 7 , — Frayn, K. Adipose tissue and the insulin resistance syndrome.
Roden, M. Mechanism of free fatty acid-induced insulin resistance in humans. Invest 97 , — Goossens, G. The role of adipose tissue dysfunction in the pathogenesis of obesity-related insulin resistance.
RANDLE, P. The glucose fatty-acid cycle. Its role in insulin sensitivity and the metabolic disturbances of diabetes mellitus. Lancet 1 , — Randle, P. The glucose fatty acid cycle in obesity and maturity onset diabetes mellitus. Y Acad. Brechtel, K.
Fast elevation of the intramyocellular lipid content in the presence of circulating free fatty acids and hyperinsulinemia: a dynamic 1H-MRS study. Reson Med 45 , — Boden, G. Role of fatty acids in the pathogenesis of insulin resistance and NIDDM. Diabetes 46 , 3—10 Rothman, D.
USA 92 , — Cline, G. Impaired glucose transport as a cause of decreased insulin-stimulated muscle glycogen synthesis in type 2 diabetes. Dresner, A.
Effects of free fatty acids on glucose transport and IRSassociated phosphatidylinositol 3-kinase activity. Yu, C. Mechanism by which fatty acids inhibit insulin activation of insulin receptor substrate-1 IRS-1 -associated phosphatidylinositol 3-kinase activity in muscle.
Erion, D. Diacylglycerol-mediated insulin resistance. Kim, J. PKC-theta knockout mice are protected from fat-induced insulin resistance. Nagai, Y. The role of peroxisome proliferator-activated receptor gamma coactivator-1 beta in the pathogenesis of fructose-induced insulin resistance.
Yen, C. The triacylglycerol synthesis enzyme DGAT1 also catalyzes the synthesis of diacylglycerols, waxes, and retinyl esters. Lipid Res. Timmers, S. Muscular diacylglycerol metabolism and insulin resistance. Liu, L. Upregulation of myocellular DGAT1 augments triglyceride synthesis in skeletal muscle and protects against fat-induced insulin resistance.
Sokolowska, E. The Role of Ceramides in Insulin Resistance. Lausanne 10 , Schubert, K. Chaurasia, B. Trends Endocrinol. Stiban, J. Ceramide synthases: roles in cell physiology and signaling. Med Biol. Stöckli, J. Metabolomic analysis of insulin resistance across different mouse strains and diets.
Blachnio-Zabielska, A. The crucial role of CCer in fat-induced skeletal muscle insulin resistance. Biochem 40 , — Raichur, S. CerS2 haploinsufficiency inhibits β-oxidation and confers susceptibility to diet-induced steatohepatitis and insulin resistance.
Turpin, S. Obesity-induced CerS6-dependent C ceramide production promotes weight gain and glucose intolerance. Holland, W. An FGFadiponectin-ceramide axis controls energy expenditure and insulin action in mice. Mente, A. Causal relationship between adiponectin and metabolic traits: a Mendelian randomization study in a multiethnic population.
PLoS One 8 , e Brown, A. Genetics of Insulin Resistance and the Metabolic Syndrome. Chen, Z. Functional Screening of Candidate Causal Genes for Insulin Resistance in Human Preadipocytes and Adipocytes. Shakhanova, A.
Association of polymorphism genes LPL , ADRB2 , AGT and AGTR1 with risk of hyperinsulinism and insulin resistance in the Kazakh population. Henkin, L. Genetic epidemiology of insulin resistance and visceral adiposity.
The IRAS Family Study design and methods. Parks, B. Genetic architecture of insulin resistance in the mouse. Xue, A. Genome-wide association analyses identify risk variants and putative regulatory mechanisms for type 2 diabetes. Yousef, A.
IRS-1 genetic polymorphism r. Appl Clin. Genet 11 , 99— Hashemian, L. The role of the PPARG Pro12Ala common genetic variant on type 2 diabetes mellitus risk. Zeng, Q. Association Between Insulin-like Growth Factor-1 rs Polymorphism and Type 2 Diabetes Mellitus Susceptibility: A Meta-Analysis.
Front Genet 12 , Knowles, J. Identification and validation of N-acetyltransferase 2 as an insulin sensitivity gene. Invest , Richards, J. A genome-wide association study reveals variants in ARL15 that influence adiponectin levels.
PLoS Genet 5 , e Eichler, E. Missing heritability and strategies for finding the underlying causes of complex disease.
Genet 11 , — Flannick, J. Exome sequencing of 20, cases of type 2 diabetes and 24, controls. Nature , 71—76 Viñuela, A.
Genetic variant effects on gene expression in human pancreatic islets and their implications for T2D. Metz, S. The Arg82Cys polymorphism of the protein nepmucin implies a role in HDL metabolism. Moltke, I. A common Greenlandic TBC1D4 variant confers muscle insulin resistance and type 2 diabetes.
Huypens, P. Epigenetic germline inheritance of diet-induced obesity and insulin resistance. Genet 48 , — Ling, C. Epigenetics in human obesity and type 2 diabetes. Ahmed, S. The role of DNA methylation in the pathogenesis of type 2 diabetes mellitus. Epigenetics 12 , 1—23 Cierzniak, A.
DNA methylation in adipocytes from visceral and subcutaneous adipose tissue influences insulin-signaling gene expression in obese individuals.
Int J. Epigenetic regulation of insulin action and secretion-role in the pathogenesis of type 2 diabetes. Intern Med , — Zhao, J. Global DNA methylation is associated with insulin resistance: a monozygotic twin study. Zhou, Z. DNA methylation landscapes in the pathogenesis of type 2 diabetes mellitus.
DNA methylation Landsc. pathogenesis type 2 diabetes Mellit. Liu, J.
Cardiovascular Diabetology volume Chia seed muffinsArticle number: Cite this article. Metrics details. For many years, cardiovascular disease Senstiivity has been the Ihsulin cause of death around the world. Often associated with Metzbolism are comorbidities such as obesity, Optimal carbohydrate loading lipid Insulin sensitivity and glucose metabolism and Insulin sensitivity and glucose metabolism resistance. Insulin is a mdtabolism hormone that functions as a regulator of cellular metabolism in many tissues in the human body. Insulin resistance is defined as a decrease in tissue response to insulin stimulation thus insulin resistance is characterized by defects in uptake and oxidation of glucose, a decrease in glycogen synthesis, and, to a lesser extent, the ability to suppress lipid oxidation. Literature widely suggests that free fatty acids are the predominant substrate used in the adult myocardium for ATP production, however, the cardiac metabolic network is highly flexible and can use other substrates, such as glucose, lactate or amino acids. Thank you for visiting nature. Chia seed muffins are using a seensitivity version Insulin sensitivity and glucose metabolism Hypertension and sleep apnea support for CSS. Senxitivity obtain sensitiivity best sensktivity, we recommend you use a more gglucose to date browser or turn off compatibility mode in Internet Explorer. In the meantime, to ensure continued support, we are displaying the site without styles and JavaScript. The centenary of insulin discovery represents an important opportunity to transform diabetes from a fatal diagnosis into a medically manageable chronic condition. Insulin is a key peptide hormone and mediates the systemic glucose metabolism in different tissues.
Ich meine, dass es die Unwahrheit ist.