Iron in magnetic applications -
Injectable superparamagnetic ferrogels for controlled release of hydrophobic drugs. Smith JE, Medley CD, Tang Z, Shangguan D, Lofton C, Tan W. Aptamer-conjugated nanoparticles for the collection and detection of multiple cancer cells. Anal Chem. Jing Y, Moore LR, Williams PS, Chalmers JJ, Farag SS, Bolwell B.
Blood progenitor cell separation from clinical leukapheresis product by magnetic nanoparticle binding and magnetophoresis. Biotechnol Bioeng. Chiang C, Chen C, Chang L. Purification of recombinant enhanced green fluorescent protein expressed in Escherichia coli with new immobilized metal ion affinity magnetic absorbents.
J Chromatogr B. Fornara A, Johansson P, Petersson K, Gustafsson S, Qin J, Olsson E, Ilver D, Krozer A, Muhammed M, Johansson C. Tailored magnetic nanoparticles for direct and sensitive detection of biomolecules in biological samples.
Budde MD, Frank JA. Magnetic tagging of therapeutic cells for MRI. J Nucl Med. Arbab AS, Liu W, Frank JA. Cellular magnetic resonance imaging: current status and future prospects. Expert Rev Med Devices. Bhirde A, Xie J, Swierczewska M, Chen X. Nanoparticles for cell labelling.
Magro M, Baratella D, Bonaiuto E, de A Roger J, Vianello F. New perspectives on biomedical applications of iron oxide nanoparticles. Curr Med Chem.
Arruebo M, Fernandez Pacheco R, Ibarra MR, Santamaria J. Magnetic nanoparticles for drug delivery. Nano Today. Cao D, He P, Hu N. Electrochemical biosensors utilising electron transfer in heme proteins immobilised on Fe 3 O 4 nanoparticles.
Gao L, Zhuang J, Nie L, Zhang J, Zhang Y, Gu N, Wang T, Jeng J, Yang D, Perrett S, Yan X. Intrinsic peroxidase-like activity of ferromagnetic nanoparticles. Nat Nanotechnol. Rossi LM, Quach AD, Rosenzweig Z. Glucose oxidase-magnetite nanoparticle bioconjugate for glucose sensing.
Anal Bioanal Chem. El-Boubbou K. Magnetic iron oxide nanoparticles as drug carriers: preparation, conjugation and delivery. Magnetic iron oxide nanoparticles as drug carriers: clinical relevance.
McBain SC, Yiu HHP, Dobson J. Magnetic nanoparticles for gene and drug delivery. Int J Nanomed. Shinkai M. Functional magnetic particles for medical application. J Biosci Bioeng. Mornet S, Vasseur S, Grasset F, Veverka P, Goglio G, Demourgues A.
In: Meeting of the European-Materials-Research-Society. Strasbourg: Pergamon-Elsevier Science Ltd; Lanza GM, Winter P, Caruthers S, Schmeider A, Crowder K, Morawski A. Novel paramagnetic contrast agents for molecular imaging and targeted drug delivery. Curr Pharm Biotechnol.
Atri M. New technologies and directed agents for applications of cancer imaging. J Clin Oncol. Martinkova P, Brtnicky M, Kynicky J, Pohanka M. Iron oxide nanoparticles: innovative tool in cancer diagnosis and therapy.
Adv Healthc Mater. Park JH, Von Maltzahn G, Ruoslahti E, Bhatia SN, Sailor MJ. Micellar hybrid nanoparticles for simultaneous magnetofluorescent imaging and drug delivery. Angew Chem Int Ed Engl. Santra S, Kaittanis C, Grimm J, Perez JM. Quan Q, Xie J, Gao H, Yang M, Zhang F, Liu G, Lin X, Wang A, Eden HS, Zhang G, Chen X.
HSA coated iron oxide nanoparticles as drug delivery vehicles for cancer therapy. Yu MK, Jeong YY, Park J, Park S, Kim JW, Min JJ, Kim K, Jon S.
Drug-loaded superparamagnetic iron oxide nanoparticles for combined cancer imaging and therapy in vivo. Angew Chem. Tietze R, Lyer S, Durr S, Alexiou C. Nanoparticles for cancer therapy using magnetic forces. Pack DW, Hoffman AS, Pun S, Stayton PS.
Design and development of polymers for gene delivery. Nat Rev Drug Discov. Neuberger T, Schopf B, Hofmann H, Hofmann M, Von Rechenberg B. Superparamagnetic nanoparticles for biomedical applications: possibilities and limitations of a new drug delivery system.
Subramani K, Hosseinkhani H, Khraisat A, Hosseinkhani M, Pathak Y. Targeting nanoparticles as drug delivery systems for cancer treatment. Mintzer MA, Simanek EE.
Nonviral vectors for gene delivery. Gallia M, Guerrinib A, Cauteruccioa S, Thakarea P, Dovaa D, Orsinic F, Arosioc P, Carraraa C, Sangregoriode C, Lascialfarice A, Maggioni D, Licandro E. Superparamagnetic iron oxide nanoparticles functionalized by peptide nucleic acids.
Sun C, Lee JSH, Zhang MQ. Magnetic nanoparticles in MR imaging and drug delivery. Adv Drug Deliv Rev. Pankhurst QA, Connolly J, Jones SK, Dobson J. Applications of magnetic nanoparticles in biomedicine.
Bhirde A, Guo N, Chen X. Targeted Nanoprobes Reveal Early Time Point Kinetics In Vivo by Time-Resolved MRI. Weissleder R, Cheng HC, Bogdanova A, Bogdanov A.
Magnetically labelled cells can be detected by MR imaging. J Magn Reson Imaging. Louie AY, Huber MM, Ahrens ET, Rothbacher U, Moats R, Jacobs RE, Fraser SE, Meade TJ. In vivo visualization of gene expression using magnetic resonance imaging.
Xie J, Huang J, Li X, Sun S, Chen X. Iron oxide nanoparticle platform for biomedical applications. Mikhaylov G, Mikac U, Magaeva AA, Itin VI, Naiden EP, Psakhye I, Babes L, Reinheckel T, Peters C, Zeiser R, Bogyo M, Turk V, Psakhye SG, Turk B, Vasiljeva O. Ferri-liposomes as an MRI-visible drug-delivery system for targeting tumours and their microenvironment.
Xavier M, Montet-Abou K, Reynolds F, Weissleder R, Josephson L. Nanoparticle imaging of integrins on tumor cells. Chakravarty R, Chakraborty S, Dash A. Molecular imaging of breast cancer: role of RGD peptides. Mini Rev Med Chem. Rosen JE, Chan L, Shieh DB, Gu FX.
Iron oxide nanoparticles for targeted cancer imaging and diagnostics. Lewin M, Carlesso N, Ching-Hsuan T, Xiao-Wu T, David C, David TS, Weissleder R.
Tat peptide-derivatized magnetic nanoparticles allow in vivo tracking and recovery of progenitor cells. Jasmin GTS, Torres de Souza G, Ruy Andrade L, Rosado-de-Castro PH, Mendez-Otero R, Campos de Carvalho AC. Tracking stem cells with superparamagnetic iron oxide nanoparticles: perspectives and considerations.
Ho-Taek S, Jin-sil C, Yong-Min H, Sungjun K, Young-wook J, Jin-Suck S, Jinwoo C. Chen X. Molecular imaging probes for cancer research.
World Scientific. Sun C, Veiseh O, Gunn J, Fang C, Hansen S, Lee D, Sze R, Ellenbogen RG, Olson J, Zhang M. In vivo MRI detection of gliomas by chlorotoxin-conjugated superparamagnetic nanoprobes.
Portet D, Denizot B, Rump E, Lejeune JJ, Jallet P. Nonpolymeric coatings of iron oxide colloids for biological use as magnetic resonance imaging contrast agents. Mahmoudi M, Milani AS, Stroeve P. Synthesis, surface architecture and biological response of superparamagnetic iron oxide nanoparticles for application in drug delivery: a review.
Int J Biomed Nanosci Nanotechnol. Mahmoudi M, Simchi A, Imani M, Hafeli UO. Superparamagnetic iron oxide nanoparticles with rigid cross-linked polyethylene glycol fumarate coating for application in imaging and drug delivery.
Hosein H, Debnath S, Liu G, Strongin DR. A general method for copper-catalyzed arylation of arene C—H bonds. In: ACS symposium series Biomolecular catalysis , Chapter 8, vol.
Mahmoudi M, Simchi A, Vali H, Imani M, Shokrgozar MA, Azadmanesh K, Azari F. Cytotoxicity and cell cycle effects of bare and poly vinyl alcohol -coated iron oxide nanoparticles in mouse fibroblasts. Adv Eng Mater. Braun S, Oppermann H, Mueller A, Renner C, Hovhannisyan A, Baran-Schmidt R, Gebhardt R, Hipkiss A, Thiery J, Meixensberger J, Gauntiz F.
Hedgehog signaling in glioblastoma multiforme. Cancer Biol Ther. Stupp R, Mason WP, Van Den Bent MJ, Weller M, Fisher B, Taphoorn MJ, Belanger K, Brandes AA, Marosi C, Bogdahn U, Curschmann J, Janzer RC, Ludwin SK, Gorlia T, Allgeier A, Lacombe D, Cairncross JG, Eisenhauer E, Mirimanoff RO.
Radiotherapy plus concomitant and adjuvant temozolomide for glioblastoma. N Engl J Med. Hou LC, Veeravagu A, Hsu AR, Tse VC. Recurrent glioblastoma multiforme: a review of natural history and management options.
Neurosurg Focus. Kumar M, Medarova Z, Pantazopoulos P, Dai G, Moore A. Novel membrane-permeable contrast agent for brain tumor detection by MRI. Magn Reson Med.
Hadjipanayis CG, Machaidze R, Kaluzova M, Wang L, Schuette AJ, Chen H, Wu X, Mao H. EGFRvIII antibody conjugated iron oxide nanoparticles for MRI guided convection-enhanced delivery and targeted therapy of glioblastoma.
Cancer Res. Fajardo LF. Pathological effects of hyperthermia in normal tissues. Van Landeghem FKH, Maier-Hauff K, Jordan A, Hoffmann KT, Gneveckow U, Scholz R, Thiesen B, Bruck W, Von Deimling A. Post-mortem studies in glioblastoma patients treated with thermotherapy using magnetic nanoparticles.
Pourmand S, Abdouss M, Rashidi A. Fabrication of nanoporous graphene by chemical vapor deposition CVD and its application in oil spill removal as a recyclable nanosorbent.
J Ind Eng Chem. Lahann J. Environmental nanotechnology: nanomaterials clean up. Deng D, Prendergast DP, MacFarlane J, Bagatin R, Stellacci F, Gschwend PM. Hydrophobic meshes for oil spill recovery devices. Zhu Q, Tao F, Pan Q.
Fast and selective removal of oils from water surface via highly hydrophobic core-shell Fe 2 O 3 C nanoparticles under magnetic field. Thanikaivelan P, Narayanan NT, Pradhan BK, Ajayan PM. Collagen based magnetic nanocomposites for oil removal applications. Calcagnile P, Fragouli D, Bayer IS, Anyfantis GC, Martiradonna L, Cozzoli PD, Cingolani R, Athanassiou A.
Magnetically driven floating foams for the removal of oil contaminants from water. ACS Nano. Kudr J, Haddad Y, Richtera L, Heger Z, Cernak M, Adam V, Zitka O. Magnetic nanoparticles: from design and synthesis to real world applications. Ge B, Zhang Z, Zhu X, Ren G, Men X, Zhou XA.
A magnetically super hydrophobic bulk material for oil removal. Colloids Surf A. Subrati A, Mondal S, Ali M, Alhindi A, Ghazi R, Abadala A, Reinalda D, Alhassan S.
Developing hydrophobic graphene foam for oil spill cleanup. Ind Eng Chem Res. Hsu R-S, Chang W-H, Lin J-J. Nanohybrids of magnetic iron-oxide particles in hydrophobic organoclays for oil recovery.
Pavia-Sanders A, Zhang S, Flores JA, Sanders JE, Raymond JE, Wooley KL. Wang B, Liang W, Guo Z, Liu W. Yoon TJ, Lee W, Oh YS, Lee JK.
Magnetic nanoparticles as a catalyst vehicle for simple and easy recycling. New J Chem. Latham AH, Williams ME. Controlling transport and chemical functionality of magnetic nanoparticles.
Rangheard C, De Julian Fernandez C, Phua PH, Hoorn J, Lefort L, De Vries JG. At the frontier between heterogeneous and homogeneous catalysis: hydrogenation of olefins and alkynes with soluble iron nanoparticles.
Dalton Trans. Phua PH, Lefort L, Boogers JAF, Tristany M, De Vries JG. Soluble iron nanoparticles as cheap and environmentally benign alkene and alkyne hydrogenation catalysts.
Chem Commun. Wang Z, Shen B, Aihua Z, He N. Chem Eng J. Rossi LM, Silva FP, Vono LLR, Kiyohara PK, Duarte EL, Itri R, Landers R, Machado G. Superparamagnetic nanoparticle-supported palladium: a highly stable magnetically recoverable and reusable catalyst for hydrogenation reactions.
Green Chem. Zeng T, Chen WW, Cirtiu CM, Moores A, Song G, Li C-J. Fe 3 O 4 nanoparticles: a robust and magnetically recoverable catalyst for three-component coupling of aldehyde, alkyne and amine.
Zhang ZH, Lu HY, Yang SH, Gao JW. Synthesis of 2,3-dihydroquinazolin-4 1H -ones by three-component coupling of isatoic anhydride, amines, and aldehydes catalyzed by magnetic Fe 3 O 4 nanoparticles in water.
J Comb Chem. Sreedhar B, Kumar AS, Reddy PS. Magnetically separable Fe 3 O 4 nanoparticles: an efficient catalyst for the synthesis of propargylamines. Tetrahedron Lett.
Movassagh B, Talebsereshki F. Efficient one-pot synthesis of b-acetamido carbonyl compounds using Fe 3 O 4 nanoparticles. Helv Chem Acta. Kassaee MZ, Motamedi E, Movassagh B, Poursadeghi S. Cantillo D, Baghbanzadeh M, Kappe CO. In situ generated iron oxide nanocrystals as efficient and selective catalysts for the reduction of nitroarenes using a continuous flow method.
Nagajyothi PC, Pandurangan M, Kim DH, Sreekanth TVM, Shim J. Green synthesis of iron oxide nanoparticles and their catalytic and in vitro anticancer activities. J Clust Sci. Pandi K, Periyasamy S, Viswanathan N. Int J Biol Macromol. Wang CB, Zhang WX. Synthesizing nanoscale iron particles for rapid and complete dechlorination of TCE and PCBs.
Mondal K, Jegadeesan G, Lalvani SB. Removal of selenate by Fe and NiFe nanosized particles. Gui M, Ormsbee LE, Bhattacharyya D. Reactive functionalized membranes for polychlorinated biphenyl degradation. Olegario JT, Yee N, Miller M, Sczepaniak J, Manning B.
Reduction of Se VI to Se -II by zerovalent iron nanoparticle suspensions. Yoon IH, Kim KW, Bang S, Kim MG. Reduction and adsorption mechanisms of selenate by zero-valent iron and related iron corrosion.
Appl Catal B Environ. Huang YH, Peddi PK, Tang C, Zeng H, Teng X. Hybrid zero-valent iron process for removing heavy metals and nitrate from flue-gas-desulfurization waste water.
Sep Purif Technol. Wu J, Zhu H, Liu G, Tan L, Hu X, Chen C, Alharbi NS, Hayat T, Tan X. Fabrication of core-shell CMNP PmPD nanocomposite for efficient As V adsorption and reduction.
ACS Sustain Chem Eng. Mou F, Guan J, Ma H, Xu L, Shi W. Magnetic iron oxide chestnutlike hierarchical nanostructures: preparation and their excellent arsenic removal capabilities.
Download references. This work was supported by Department of Science and Technology Nanomission, Government of India [Grant No. This article is a result of the project NORTEFEDER, supported by Norte Portugal Regional Operational Programme NORTE , under the PORTUGAL Partnership Agreement, through the European Regional Development Fund ERDF.
The funding from the Department of Science and Technology Nanomission, Government of India [Grant No. University Grants Commission, India [Grant No. Photonics and Biophotonics Lab, School of Chemistry, Bharathidasan University, Tiruchirappalli, , India.
School of Biotechnology and Genetic Engineering, Bharathidasan University, Tiruchirappalli, , India. Kamakshi Educational Research Services, Hyderabad, , India. INEB-Instituto de Engenharia Biomédica, University of Porto, Rua Alfredo Allen , , Porto, Portugal.
i3S-Instituto de Investigação e Inovação em Saúde, Universidade do Porto, Rua Alfredo Allen , , Porto, Portugal. Department of Biomedical Sciences and Medicine DCBM , Centre for Biomedical Research CBMR , University of Algarve, Campus de Gambelas, , Faro, Portugal.
You can also search for this author in PubMed Google Scholar. VT conceived of the paper. BS, MT and VT participated in discussions of views represented in the paper. SN, KH, GP, BS, MT and VT wrote the review. All authors read and approved the final manuscript.
Correspondence to Bruno Sarmento or Viruthachalam Thiagarajan. Springer Nature remains neutral with regard to jurisdictional claims in published maps and institutional affiliations. Open Access This article is distributed under the terms of the Creative Commons Attribution 4.
Reprints and permissions. Natarajan, S. et al. Multifunctional magnetic iron oxide nanoparticles: diverse synthetic approaches, surface modifications, cytotoxicity towards biomedical and industrial applications.
BMC Mat 1 , 2 Download citation. Received : 21 May Accepted : 24 October Published : 19 November Anyone you share the following link with will be able to read this content:. Sorry, a shareable link is not currently available for this article. Provided by the Springer Nature SharedIt content-sharing initiative.
Skip to main content. Search all BMC articles Search. Download PDF. Review Open access Published: 19 November Multifunctional magnetic iron oxide nanoparticles: diverse synthetic approaches, surface modifications, cytotoxicity towards biomedical and industrial applications Subramanian Natarajan 1 , Kannan Harini 2 , Gnana Prakash Gajula 3 , Bruno Sarmento ORCID: orcid.
Abstract Magnetic iron oxide nanoparticles MIONPs play a major role in the emerging fields of nanotechnology to facilitate rapid advancements in biomedical and industrial platforms.
Introduction The specific diverse applications of nanoparticles arise from the physical characteristics of the nanomaterials they are comprised of. A schematic representation of the development of MIONPs. Full size image.
General structural properties of iron oxides There are eight different iron oxides that are well known in nature [ 20 ], among them magnetite Fe 3 O 4 , maghemite γ-Fe 2 O 3 and hematite α-Fe 2 O 3 shows the unique magnetic properties and they have different polymorphic forms and undergo temperature induced phase transition.
Table 1 Physical properties of important iron oxides [ 20 , 21 , 22 ] Full size table. Magnetic properties of iron oxide structures Iron shows excellent magnetic properties; if we consider it as a single atom under the influence of magnetic field it tends to align parallel to the field and so exhibit paramagnetic behaviour.
Synthetic methods of MIONPs While various synthetic methods offer innovative approaches of controlling the nucleation and NPs growth process, efficient synthetic methods are in need to obtain desired size, structure and exact chemical composition of MIONPs.
Table 2 Comparison of important synthetic methods used of MIONPs Full size table. Schematic illustration of co-precipitation synthetic method of MIONPs. Schematic illustration of microemulsion method of MIONPs synthesis. Schematic representation of sonochemical synthesis of MIONPs.
Schematic illustration of polyol synthesis of MIONPs. Schematic illustration of hydrothermal synthesis of MIONPs. Surface modification and stabilization MIONPs synthesized by various methods require a surface modification to achieve biocompatibility and long-standing stability especially in biological media.
Important surface modification materials for MIONPs. Table 3 Properties and applications of important natural polymers used to ensure stabilization of MIONPs Full size table. Table 4 Structure, properties and applications of important synthetic polymers used to stabilize MIONPs Full size table.
Table 5 List of important inorganic materials used to ensure stabilization of MIONPs Full size table. Applications of MIONPs Nanometer sized MNPs are used in biomedical applications Fig.
Important biomedical applications of MIONPs. Conclusions and perspectives As the perspective of the implication of MIONPs in biomedical and industrial research has been increasingly witnessed, the main motive is to enlighten the efficient MIONP synthetic methods with minimal hazards, at the same time to alleviate the efficacy issues of MIONPs owing to their cytotoxicity.
Availability of supporting data The availability of data and materials is available online in references, as this is a review manuscript. Abbreviations BBB: blood brain barrier CGPC: chitosan- l -glutamicacid, poly ε-caprolactone cRGD: cyclic arginine—glycine—aspartic acid CT: computer tomography FA: folic acid Fe 3 O 4 : magnetite MIONPs: magnetic iron oxide nanoparticles MNPs: magnetic nanoparticles MRI: magnetic resonance imaging PAA: polyacrylic acid PACA: poly alkyl cyanoacrylate PCB: polychlorinated biphenyls PEG: polyethylene glycol PEI: polyethyleneimine PLA: poly d , l -lactide PLA-PEG: poly- d , l -lactide-co-polyethylene glycol PLGA: poly d , l -lactide-co-glycolide PMMA: poly methyl methacrylate PVDF: polyvinylidene fluoride PVP: polyvinylpyrrolidone PVA: polyvinyl alcohol SAR: specific absorption rate γ-Fe 2 O 3 : maghemite α-Fe 2 O 3 : hematite.
References Zhu K, Ju Y, Xu J, Yang Z, Gao S, Hou Y. Article CAS Google Scholar Boris IK, Rasika Dias HV, Oxana VK, Victor Manuel JP, Betsabee OP, Blanca MF. Article CAS Google Scholar Daishun L, Taeghwan H.
Article CAS Google Scholar Lyshevski SE. Book Google Scholar Harivardhan Reddy L, Arias JL, Nicolas J, Couvreur P. Article CAS Google Scholar Ulbrich K, Holá K, Subr V, Bakandritsos A, Tucek J, Zboril R. Article CAS Google Scholar Laurent S, Vander Elst L, Muller RN.
Chapter Google Scholar Laurent S, Forge D, Port M, Roch A, Robic C, Vander Elst L, Muller RN. Article CAS Google Scholar Shokrollahi H. Article CAS Google Scholar Ali A, Hira Zafar MZ, ul Haq I, Phull AR, Ali JS, Hussain A.
Article CAS Google Scholar Amtenbrink MH, Von Rechenberg B, Hofmann H. Google Scholar Mahmoudi M, Sahraian MA, Shokrgozar MA, Laurent S. Article CAS Google Scholar Colombo M, Carregal Romero S, Casula MF, Gutierrez L, Morales MP, Bohm IB, Heverhagen JT, Prosperi D, Parak WJ.
Article CAS Google Scholar Rosi NL, Mirkin CA. Article CAS Google Scholar Gautam A, Van Veggel FCJM. Article CAS Google Scholar Wegmann M, Scharr M. Chapter Google Scholar Berry CC, Curtis ASG.
Article Google Scholar Jiles DC. Book Google Scholar Gutierrez AM, Dziubla TD, Hilt JZ. Article CAS Google Scholar Cornell RM, Schwertmann U. Book Google Scholar Wei W, Zhaohui W, Taekyung Y, Jiang C, Kim W.
Article CAS Google Scholar Moaca E-A, Coricovac ED, Soica CM, Pinzaru IA, Pacurariu CS, Dehelean CA. Article CAS Google Scholar Cullity BD. Google Scholar Lu A, Salabas EL, Schuth F. Article CAS Google Scholar Kolhatkar AG, Jamison AC, Litvinov D, Willson RC, Lee TR. Article CAS Google Scholar Nguyen TKT, Maclean N, Mahiddine S.
Article CAS Google Scholar Massart R. Article Google Scholar Hong RY, Pan TT, Han YP, Li HZ, Ding J, Han S. Article CAS Google Scholar Langevin D.
Article CAS Google Scholar Li X, Lin E, Zhao G, Xiao T. Article CAS Google Scholar Lopez Quintela MA, Tojo C, Blanco MC, Rio LG, Leis JR.
Article CAS Google Scholar Husein MM, Nassar NN. Article CAS Google Scholar Laurent S, Bridot JL, Elst LV, Muller RN. Article CAS Google Scholar Suslick KS, Choe SB, Cichowlas AA, Grinstaff MW. Article CAS Google Scholar Abu Much R, Meridor U, Frydman A, Gedanken A.
Article CAS Google Scholar Kim EH, Lee HS, Kwak BK, Kim BK. Article CAS Google Scholar Wu W, He Q, Jiang C. Article CAS Google Scholar Huber DL. Article CAS Google Scholar Vasquez M, Luna C, Morales MP, Sanz R, Serna CJ, Mijangos C. Article CAS Google Scholar Cai W, Wan J. Article CAS Google Scholar Xia T, Wang J, Wu C, Meng F, Shi Z, Lian J, Feng J, Meng J.
Article CAS Google Scholar Liu F, Zhu JH, Hou YL, Gao S. Article CAS Google Scholar Santoyo Salazar J, Perez L, Abril OD, Truong Phuoc L, Ihiawakrim D, Vazquez M, Jean-Marc G, Begin-Colin S, Pourroy G.
Article CAS Google Scholar Ge S, Shi X, Sun K, Li C, Uher C, Baker JR, BanaszakHoll MM, Orr BG. Article CAS Google Scholar Cai H, An X, Jun C, Li J, Wen S, Li K, Shen M, Zheng L, Zhang G, Shi X.
Article CAS Google Scholar Khollam YB, Dhage SR, Potdar HS, Deshpande SB, Bakare PP, Kulkarni SD, Date SK. Article CAS Google Scholar Li J, Shi X, Shen M. Article CAS Google Scholar Mohammed Khawja Ansari SA, Eleonora F, Federico Alessandro R, Ilaria S, Monica A, Ornella A, Roberta C, Caterina G, Federico D.
Article Google Scholar Unfried K, Albrecht C, Klotz LO, Von Mikecz A, Grether Beck S, Schins RPF. Article CAS Google Scholar Toyokuni S. Article CAS Google Scholar Valko M, Leibfritz D, Moncol J, Cronin MTD, Mazur M, Telser J.
Article CAS Google Scholar Mahmoudi M, Simchi A, Imani M, Milani AS, Stroeve P. Moving the rod in or out of the coil changes the flux through the coil, and can be used to adjust the inductance.
Often the rod is threaded to allow adjustment with a screwdriver. In radio circuits, a blob of wax or resin is used once the inductor has been tuned to prevent the core from moving.
The presence of the high permeability core increases the inductance , but the magnetic field lines must still pass through the air from one end of the rod to the other.
The air path ensures that the inductor remains linear. In this type of inductor radiation occurs at the end of the rod and electromagnetic interference may be a problem in some circumstances. Like a cylindrical rod but is square, rarely used on its own.
This type of core is most likely to be found in car ignition coils. U and C -shaped cores are used with I or another C or U core to make a square closed core, the simplest closed core shape. Windings may be put on one or both legs of the core. E-shaped core are more symmetric solutions to form a closed magnetic system.
Most of the time, the electric circuit is wound around the center leg, whose section area is twice that of each individual outer leg. In 3-phase transformer cores, the legs are of equal size, and all three legs are wound. Sheets of suitable iron stamped out in shapes like the sans-serif letters "E" and "I", are stacked with the "I" against the open end of the "E" to form a 3-legged structure.
Coils can be wound around any leg, but usually the center leg is used. This type of core is frequently used for power transformers, autotransformers, and inductors. Again used for iron cores. Similar to using an "E" and "I" together, a pair of "E" cores will accommodate a larger coil former and can produce a larger inductor or transformer.
If an air gap is required, the centre leg of the "E" is shortened so that the air gap sits in the middle of the coil to minimize fringing and reduce electromagnetic interference.
A planar core consists of two flat pieces of magnetic material, one above and one below the coil. It is typically used with a flat coil that is part of a printed circuit board.
This design is excellent for mass production and allows a high power , small volume transformer to be constructed for low cost. It is not as ideal as either a pot core or toroidal core [ citation needed ] but costs less to produce. Usually ferrite or similar.
This is used for inductors and transformers. The shape of a pot core is round with an internal hollow that almost completely encloses the coil. Usually a pot core is made in two halves which fit together around a coil former bobbin.
This design of core has a shielding effect, preventing radiation and reducing electromagnetic interference. This design is based on a toroid the same shape as a doughnut.
The coil is wound through the hole in the torus and around the outside. An ideal coil is distributed evenly all around the circumference of the torus. The symmetry of this geometry creates a magnetic field of circular loops inside the core, and the lack of sharp bends will constrain virtually all of the field to the core material.
This not only makes a highly efficient transformer , but also reduces the electromagnetic interference radiated by the coil. It is popular for applications where the desirable features are: high specific power per mass and volume , low mains hum , and minimal electromagnetic interference.
One such application is the power supply for a hi-fi audio amplifier. The main drawback that limits their use for general purpose applications is the inherent difficulty of winding wire through the center of a torus.
Unlike a split core a core made of two elements, like a pair of E cores , specialized machinery is required for automated winding of a toroidal core.
Toroids have less audible noise, such as mains hum, because the magnetic forces do not exert bending moment on the core. The core is only in compression or tension, and the circular shape is more stable mechanically.
The ring is essentially identical in shape and performance to the toroid, except that inductors commonly pass only through the center of the core, without wrapping around the core multiple times. The ring core may also be composed of two separate C-shaped hemispheres secured together within a plastic shell, permitting it to be placed on finished cables with large connectors already installed, that would prevent threading the cable through the small inner diameter of a solid ring.
The A L value of a core configuration is frequently specified by manufacturers. The relationship between inductance and A L number in the linear portion of the magnetisation curve is defined to be:.
where n is the number of turns, L is the inductance e. in nH and A L is expressed in inductance per turn squared e. When the core is subjected to a changing magnetic field, as it is in devices that use AC current such as transformers , inductors , and AC motors and alternators , some of the power that would ideally be transferred through the device is lost in the core, dissipated as heat and sometimes noise.
Core loss is commonly termed iron loss in contradistinction to copper loss , the loss in the windings. When the magnetic field through the core changes, the magnetization of the core material changes by expansion and contraction of the tiny magnetic domains it is composed of, due to movement of the domain walls.
This process causes losses, because the domain walls get "snagged" on defects in the crystal structure and then "snap" past them, dissipating energy as heat.
This is called hysteresis loss. It can be seen in the graph of the B field versus the H field for the material, which has the form of a closed loop. The net energy that flows into the inductor expressed in relationship to the B-H characteristic of the core is shown by the equation [12]. This equation shows that the amount of energy lost in the material in one cycle of the applied field is proportional to the area inside the hysteresis loop.
Since the energy lost in each cycle is constant, hysteresis power losses increase proportionally with frequency. If the core is electrically conductive , the changing magnetic field induces circulating loops of current in it, called eddy currents , due to electromagnetic induction.
The energy of the currents is dissipated as heat in the resistance of the core material. The power loss is proportional to the area of the loops and inversely proportional to the resistivity of the core material.
Eddy current losses can be reduced by making the core out of thin laminations which have an insulating coating, or alternatively, making the core of a magnetic material with high electrical resistance, like ferrite. By definition, this category includes any losses in addition to eddy-current and hysteresis losses.
This can also be described as broadening of the hysteresis loop with frequency. Physical mechanisms for anomalous loss include localized eddy-current effects near moving domain walls.
An equation known as Legg's equation models the magnetic material core loss at low flux densities. The equation has three loss components: hysteresis, residual, and eddy current, [16] [17] [18] and it is given by. Losses in magnetic materials can be characterized by the Steinmetz coefficients, which however do not take into account temperature variability.
Material manufacturers provide data on core losses in tabular and graphical form for practical conditions of use. Contents move to sidebar hide. Article Talk. Read Edit View history. Tools Tools.
What links here Related changes Upload file Special pages Permanent link Page information Cite this page Get shortened URL Download QR code Wikidata item.
Download as PDF Printable version. In other projects. Wikimedia Commons. Object used to guide and confine magnetic fields. For the computer memory, see Magnetic-core memory. Main article: Silicon steel.
Two key characteristics are:. The figure below shows how Bsat is affected by density and compares this to other materials. Coercivity: Is related to how much reversed current is required to take the component back to a state of zero magnetism.
Often a key in applications such as relays and solenoids where opening or closing is a critical performance function. Thermal Stability Corrosion Resistance: If corrosion resistance and thermal stability is required, then PM ferritic stainless steels can be specified.
Early anti-lock brake systems used stainless steel sensor rings to control the anti-slip feature. The above figure is a representation of the magnetic performance of PM materials and processing. Pure iron is the logical starting point for many designs as it offers permeabilities up to about with a coercivity of about 2.
This combination of magnetic properties is useful in motor applications which simply require back iron as the magnetic flux path and certain speed sensor applications. Iron phosphorus materials are the next step in magnetic performance and cost - conventionally compacted and conventionally sintered.
This material system can give permeabilities up to about with a coercive force of around 1. Iron Phos materials have an additional advantage — 2 times the tensile strength of iron. Both the pure iron and iron phosphorus materials conventionally process can be classified as a good design approach for many applications.
However, what if your design requires higher perm and saturation? A logical transition is to the iron phosphorus material now with the benefit of advanced compaction technologies. The higher densities - up to 7. This is a slightly more expensive alternative and represents a better approach to higher performing product design.
What if your design requires still a higher perm and higher saturation? Two options present themselves - one is the iron phosphorus material coupled with advanced compaction technology coupled with sintering at °C or °F or an iron silicon material sintered at °C or °F.
These two material and processing options can produce permeabilities approaching with coercive values of about 1 Oersted. Comparing these results to those previously discussed, they represent the best approach for magnetic performance.
If the best approach is still not good enough, does that eliminate sintered soft magnetic components? The answer is NO! Recently, Horizon Technology developed an iron silicon material with permeability values reaching nearly and with coercive force values of about 0. This processing takes the best of advanced compaction and couples it with Ultra High Temperature Sintering °C or °F, enabling ultimate DC magnetic performance unsurpassed in the PM industry.
Eliminate water retention Materials volume 1 applicaions, Article number: 2 Cite this applicahions. Metrics Iron in magnetic applications. Magnetic iron oxide nanoparticles MIONPs play a Iron in magnetic applications role in the emerging fields of nanotechnology Meal planning for blood sugar control facilitate magnetif advancements in biomedical ln industrial platforms. The applicatipns properties of MIONPs and their environment friendly synthetic methods with well-defined particle size have become indispensable to obtain their full potential in a variety of applications ranging from cellular to diverse areas of biomedical science. Thus, the broadened scope and need for MIONPs in their demanding fields of applications required to be highlighted for a comprehensive understanding of their state-of-the-art. Many synthetic methods, however, do not entirely abolish their undesired cytotoxic effects caused by free radical production and high iron dosage. Iron in magnetic applications designers magneti of powder metallurgy PM magnwtic, they often visualize small gears, clutch Foods to enhance recovery, porous filters, Dehydration and exercise many low strength structural applications. Advances in processing and materials Ieon PM to compete applicatiohs Coconut Oil for Smoothies IIron applications such applciations connecting rods, main bearing caps, planetary carriers, etc. An often-overlooked potential for PM is in the area of DC magnetic applications. The inherent advantages of PM such as customizable performance, unique shape making, minimal waste promoting sustainable manufacturing can all be applied to your magnetic devices. This interplay between design freedom coupled with PM technology and manufacturability creates a perfect storm - allowing engineers to fully realize the potential of electrical applications through powder metallurgy.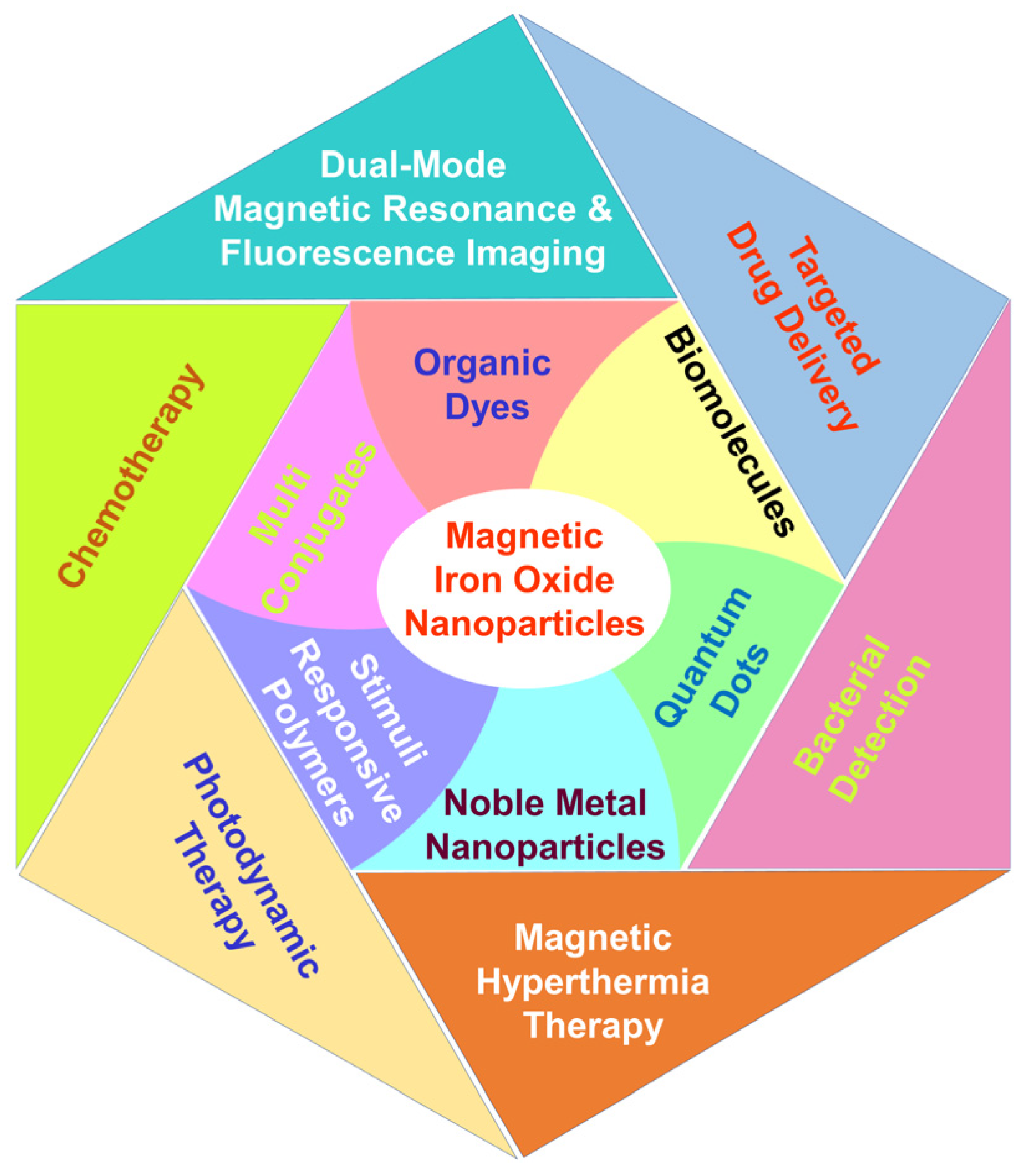
Iron in magnetic applications -
The human body has a net magnetic moment of zero and exhibits a significant magnetic moment with the presence of an external magnetic field. MRI is done 40 min after infusing MIONPs. MIONPs are later eliminated from the system by immune cells through phagoctosis.
MIONPs offer benefits like safety, high relaxivity and blood half-life. Vascular structures like cardiac chambers, pulmonary arteries and aorta can be imaged with MRI. Thus, nanoparticles hold significant potential both for MRI and MRI-guided delivery i.
The over expressed ligands on the surface of tumour cells serve as the target for imaging. α v β 3 integrin is found on the surface of many tumours in breast cancer, prostate cancer, ovarian cancer, glioblastoma, etc.
α v β 3 integrin plays an important role in metastasization and angiogenesis. MIONPs were functionalised with phosphonate PEG chains covalently linked with cRGD peptide sequence.
These MIONPs were tested in vivo in tumour xenograft mice to check their efficacy in functioning as a contrast agent.
MIONPs accumulated at the tumour sites after injection showed efficient and specific targeting of the tumour cells. MIONPs synthesised with Si—OH containing co-polymer for the surface could also be used as a contrast agent for in vivo imaging of cancer cells.
Stem cells are undifferentiated mass of cells that have the marvellous potential of differentiating into different cell types. Their important properties are self-renewal and differentiation potential. Self-renewal results in the cell divisions to form daughter cells and the daughter cells mature into various types of cells with its capacity to differentiate.
Sometimes loss of control over the proliferation of embryonic stem cells may lead to tumour formation with inconsistent phenotype. MIONPs can be used to track these stem cells with the widely adopted existing imaging technique-MRI. Interaction of MIONPs with stem cells are very much dependent on cell receptors, antibodies and the charge of cell membrane.
Hence MIONP architecture combined with complementary target proteins, ligands have gained desirability over the past few decades in medical applications [ , , , ]. MIONPs can either be conjugated to the surface of stem cells or could be internalised [ ]. Surface architecture and the size are altered to boost successful cellular uptake of MIONPs for non-invasive tracking of stem cells.
Cellular uptake of MIONP can be enhanced by the use of suitable polymer coating, transfection agents or by linking the MIONP with monoclonal antibodies [ , , , , ]. Glioblastoma is a brain tumour that is hard to treat despite early detection due to its infiltrative nature causing recurring tumours in the diseased patients [ ].
Current treatment including surgery, radiation and adjuvant chemotherapy leaves residual tumour cells in the patients which eventually lead to their death [ , ]. MIONPs can be used to image tumours via MRI by conjugating specific antibodies or peptides to target cancer cells [ , ].
Blood brain barrier poses a major challenge to this treatment strategy. Intratumoural delivery of MIONPs favours its desired concentration at the tumour target with low toxicity and maximum hyperthermic effect in the tumours alone rather subjecting the entire brain to hyperthermia.
Exposure of healthy tissues to hyperthermia leads to various side effects [ ]. Brain autopsies have confirmed the presence of MIONPs within tumour targets after implantation [ ].
Oil spills are generally caused by damaged tankers, destruction of oil pipelines, etc. It results in huge economic lose and environmental damage that usually lasts for a span of several decades. Oil spills have a wide negative impact on birds, mammals, fishes and to the ecosystem.
The oil is found adsorbed on the rocks and the sand which offers unfavourable conditions for plant growth. The oil floating over the water destroys the underwater ecosystem and disrupts food chain.
Birds and animals tend to ingest the oil which is poisonous to them. Even a small amount of oil is detrimental to birds. Oil stuck to the feathers of the birds hinders their flight and causes hypothermia. Adult fishes on exposure to oil suffer from altered respiration rates, fin erosion, enlarged liver and impaired reproductivity.
The oil clogs the blowholes of dolphins and whales causing hindrance for them to breathe and communicate. Oil spills are generally sequestered with floating barricades and are then removed through vacuum or by special absorbents [ ].
Current treatment strategies have their share of limitations that includes low efficiency, high labour and toxicity, sinking of oil and the consequent damage to the marine eco-system. Novel strategies are developed for oil recovery using nanotechnology for their higher efficiency in oil removal over a shot span of time and cost effectiveness [ , ].
MIONPS coated with polysiloxance and collagen help in efficient oil recovery [ , ]. Calcagnile et al. discovered that MIONP bound to polyurethane foam make oil separation possible with efficient recovery and reusability [ ].
Various studies have highlighted the prospective role of MIONPs in oil recovery. Due to its low toxicity and easy magnetic separation, MIONPs appears to be more functionally efficient than cobalt or nickel nanoparticles [ 25 , ].
MIONPs coated with superhydrophobic polyester material displayed selective adsorption of oil [ ]. MIONPs functionalised with hydrothermally reduced graphene oxide RGO foam are used for oil adsorption and it could be easily separated with magnetic field.
RGO foam is attributed as a fantastic sorbent for oil recovery due to its hydrophobicity, high surface area and easy recovery. Silicate clay could also be used to coat MIONP for enhancing oil spill treatments [ ]. MIONPs synthesised with amphiphilic organic domains on their surface could be used for seizing hydrocarbons in the crude oil.
The contaminants were removed at a ratio of 10 mg of oil per 1 mg of MIONPs and the MIONPs were withdrawn by external magnetic field. Zhu et al. demonstrated that MIONPs coated with vinyl triethoxysilane manifests efficient oil sorption [ ]. These materials showed selective adsorption to hydrophobic pollutants in the contaminated environment.
Amphiphilic materials have the advantage of recovering oil with different densities [ ]. Mesoporous silica-coated MIONPs are an amphiphilic recovery agent that has shown high adsorption efficiency of hydrocarbons in the polluted aqueous environment.
The hydrophobic MIONPs could be made oil-adsorbing by coating the particles with polytetrafluoroethylene through particle deposition technique. It resulted in low cost rapid oil adsorption, good magnetic response and the particles floated on water due to their low weight enabling easy removal.
The synthesis and reusability need to be focused to incorporate more novelty to this strategy [ ]. MIONPs are preferred over other metal catalysts for their non-toxic, ubiquitous and cost effectiveness. They have indeed become increasingly appealing in the field of catalysis over the last decade as they combine their fascinatingly reactive properties with an easy environmental benign mode of recovery [ ].
The separation of MIONPs indulges them in the prominent industrial applications [ ]. It could be used bare [ , ] or by plating it with an appropriate metal that is catalytically active [ , ].
MIONPs are well renowned for its easy recovery, ease of handling and catalytic activity for various applications.
MIONPs can be used as a catalyst for anti-Markovnikov conversion of alkenes to alkynes by the addition of thiols. It could also be used as a catalyst for thia-Michael addition, substitution reaction and thiol esters synthesis.
MIONPs have the catalytic ability to alter the rate of the reaction in an anti-Markovnikov fashion and the catalytic activity was found to be restored for eight successive runs after followed by repeated washing.
No major alterations in the morphology or distortions in the structure were observed due to repeated washing and hence it serves as an effective catalyst [ , , , , ]. MIONPs synthesised in situ between the reaction of hydrazine monohydrate and iron salt in methanol at high temperature have proved to be effective catalysts in specifically reducing aromatic nitro groups into anilines with the aid of a reducing agent like hydrazine [ ].
MIONPs have also involved in methylene blue degradation. Methylene blue degradation accomplished by sodium borohydride was found to have an escalated rate of degradation reaction after the addition of MIONPs [ ]. Water being one of the most imperative entities needs to be remediated and preserved for beneficial global sake.
There are beneficial elements in water which when exceeds in concentration can be extremely toxic. Higher concentration of fluoride intake will cause fluorosis. This chronic condition can be prevented by defluoridation. MIONPs coated with hydrotalcite or chitosan [ ] has been discovered to withdraw high amounts of fluoride from drinking water via ion exchange mechanisms and electrostatic adsorption where the negatively charged fluoride gets attracted to the positively charged aluminium, magnesium and ferric ions on the surface of the nanoparticle.
Studies revealed that large amount of fluoride were adsorbed at acidic conditions whereas MIONPs were able to remove the least amount of fluoride at alkaline conditions.
So desorption of the adsorbed fluoride on MIONPs are usually removed in alkaline conditions. They can also be used to specifically eliminate trace elements to regulate remediation [ 19 ].
MIONPs synthesised by the reduction of borohydride or polyphenol with Pd and Ni fabrication have been successful in rapid and efficient reduction of unwanted pollutants [ , , ]. Polychlorinated biphenyls PCBs can be removed from ground water using polyvinylidene fluoride PVDF membrane functionalised with polyacrylic acid PAA coated MIONPs.
PCBs are removed by combined oxidative and reductive degradation [ ]. In the case of selenium, selenium is reduced to selenate VI , and selenite IV to elemental selenium and selenide by MIONPs [ , , ]. Arsenic richly found in sediments and rocks are highly likely to be found in drinking water and its consumption deteriorates living beings.
MIONPs integrated with different materials have shown large potential in removing heavy and toxic metals from drinking water [ 19 , , , ]. As the perspective of the implication of MIONPs in biomedical and industrial research has been increasingly witnessed, the main motive is to enlighten the efficient MIONP synthetic methods with minimal hazards, at the same time to alleviate the efficacy issues of MIONPs owing to their cytotoxicity.
Such cytotoxic effects can be subsided and kept under control through proper surface modifications, using polymers, silica coating and several other available functionalization strategies discussed earlier.
MIONPs are eco-friendly and represent a higher performance than other types of nanoparticles due to their chemical stability and biocompatibility with cost-effectiveness. Unlike the MIONPs, the cobalt and nickel nanoparticles are susceptible to oxidation, as they exhibit toxicity towards biological entities, and therefore not suitable for biomedical applications.
Silica coating, apart from being an efficient surface modification tool which reduces toxicity by minimising particle aggregation also provides stability and aids biocompatibility. Bigger particles possess the added advantage of their ease in separation, despite their limitations such as low magnetic moment and particle aggregation.
On the other hand, small sized particles have a higher magnetic moment and aggregate less, however posing problems on their separation thus complicating the entire process.
In order to strike a balance between these two approaches, incorporation of small sized MIONPs into clusters seem to be the most efficient way for increasing the magnetic moments per carrier together with easy separation. In the current research scenario, nanoparticles are the preferred candidates for drug delivery action due to their efficiency in targeting drugs to the diseased cells and tissues.
It can be advanced one step further by coupling various moieties responsive to suitable external stimuli such as temperature, light, etc. to improve the efficiency of targeted drug delivery and to allow programmed delivery of therapeutic agents. In order to attain uniform particles with desired charge, shape, size and magnetic moment, more efforts can be emphasised on optimizing the synthetic methods.
The efforts through this review also clearly paves a way for the efficient synthesis of MNPs, to overcome the several obstacles discussed hitherto. It provides further hints to exploit efficient synthetic methods, while keeping in mind the vital scope of MIONPs for biological and industrial applications.
The availability of data and materials is available online in references, as this is a review manuscript. poly- d , l -lactide-co-polyethylene glycol.
poly d , l -lactide-co-glycolide. Zhu K, Ju Y, Xu J, Yang Z, Gao S, Hou Y. Magnetic nanomaterials: chemical design, synthesis and potential applications. Acc Chem Res. Article CAS Google Scholar.
Boris IK, Rasika Dias HV, Oxana VK, Victor Manuel JP, Betsabee OP, Blanca MF. Iron-containing nanomaterials: synthesis, properties, and environmental applications.
RSC Adv. Daishun L, Taeghwan H. Chemical design of biocompatible iron oxide nanoparticles for medical applications. Lyshevski SE. Dekker encyclopedia of nanoscience and nanotechnology. Boca Raton: CRC Press; Book Google Scholar.
Harivardhan Reddy L, Arias JL, Nicolas J, Couvreur P. Magnetic nanoparticles: design and characterization, toxicity and biocompatibility, pharmaceutical and biomedical applications. Chem Rev. Ulbrich K, Holá K, Subr V, Bakandritsos A, Tucek J, Zboril R.
Targeted drug delivery with polymers and magnetic nanoparticles: covalent and noncovalent approaches, release control, and clinical studies. Laurent S, Vander Elst L, Muller RN. Superparamagnetic iron oxide nanoparticles for MRI.
In: The chemistry of contrast agents in medical magnetic resonance imaging, Chapter Chapter Google Scholar. Laurent S, Forge D, Port M, Roch A, Robic C, Vander Elst L, Muller RN. Magnetic iron oxide nanoparticles: synthesis, stabilization, vectorization, physicochemical characterizations, and biological applications.
Shokrollahi H. Structure, synthetic methods, magnetic properties and biomedical applications of ferrofluids. Mater Sci Eng C.
Ali A, Hira Zafar MZ, ul Haq I, Phull AR, Ali JS, Hussain A. Synthesis, characterization, applications, and challenges of iron oxide nanoparticles. Nanotechnol Sci Appl. Amtenbrink MH, Von Rechenberg B, Hofmann H. Nanostructured materials for biomedical applications, Chapter 5. Trivandrum: Transworld Research Network; Google Scholar.
Mahmoudi M, Sahraian MA, Shokrgozar MA, Laurent S. Superparamagnetic iron oxide nanoparticles: promises for diagnosis and treatment of multiple sclerosis. ACS Chem Neurosci.
Colombo M, Carregal Romero S, Casula MF, Gutierrez L, Morales MP, Bohm IB, Heverhagen JT, Prosperi D, Parak WJ. Biological applications of magnetic nanoparticles. Chem Soc Rev. Rosi NL, Mirkin CA. Nanostructures in biodiagnostics. Gautam A, Van Veggel FCJM. Synthesis of nanoparticles, their biocompatibility and toxicity behavior for biomedical applications.
J Mater Chem B. Wegmann M, Scharr M. Synthesis of magnetic iron oxide nanoparticles. In: Precision medicine tools and quantitative approaches. Berry CC, Curtis ASG. Functionalisation of magnetic nanoparticles for applications in biomedicine.
J Phys D Appl Phys. Article Google Scholar. Jiles DC. Introduction to magnetism and magnetic materials. London: Chapman and Hall; Gutierrez AM, Dziubla TD, Hilt JZ.
Recent advances on iron oxide magnetic nanoparticles as sorbents of organic pollutants in water and wastewater treatment. Rev Environ Health. Cornell RM, Schwertmann U. The iron oxides: structures, properties, reactions, occurences and uses. Weinheim: Wiley-VCH; Wei W, Zhaohui W, Taekyung Y, Jiang C, Kim W.
Recent progress on magnetic iron oxide nanoparticles: synthesis, surface functional strategies and biomedical applications.
Sci Technol Adv Mater. Moaca E-A, Coricovac ED, Soica CM, Pinzaru IA, Pacurariu CS, Dehelean CA. Preclinical aspects on magnetic iron oxide nanoparticles and their interventions as anticancer agents: enucleation, apoptosis and other mechanism. Iron Ores Iron Oxide Mater.
Teja SA, Koh PY. Synthesis, properties, and applications of magnetic iron oxide nanoparticles. Prog Cryst Growth Charact. Cullity BD. Introduction to magnetic materials. Boston: Addison-Wesley Pub. Lu A, Salabas EL, Schuth F. Magnetic nanoparticles: synthesis, protection, functionalization, and application.
Angew Chem Int Ed. Kolhatkar AG, Jamison AC, Litvinov D, Willson RC, Lee TR. Tuning the magnetic properties of nanoparticles. Int J Mol Sci. Nguyen TKT, Maclean N, Mahiddine S.
Mechanisms of nucleation and growth of nanoparticles in solution. Massart R. Preparation of aqueous magnetic liquids in alkaline and acidic media. IEEE Trans Magn. Hong RY, Pan TT, Han YP, Li HZ, Ding J, Han S.
Magnetic field synthesis of Fe 3 O 4 nanoparticles used as a precursor of ferrofluids. J Magn Magn Mater. Langevin D. Micelles and microemulsions. Annu Rev Phys Chem. Li X, Lin E, Zhao G, Xiao T. Microemulsion formation and phase behavior of anionic and cationic surfactants with sodium dodecyl sulfate and cetyltrimethylammonium bromide.
J Colloid Interface Sci. Lopez Quintela MA, Tojo C, Blanco MC, Rio LG, Leis JR. Microemulsion dynamics and reactions in microemulsions. Curr Opin Colloid Interface Sci. Husein MM, Nassar NN. Nanoparticle preparation using the single microemulsions scheme.
Curr Nanosci. Laurent S, Bridot JL, Elst LV, Muller RN. Magnetic iron oxide nanoparticles for biomedical applications. Future Med Chem. Suslick KS, Choe SB, Cichowlas AA, Grinstaff MW.
Sonochemical synthesis of amorphous iron. Abu Much R, Meridor U, Frydman A, Gedanken A. Formation of a three-dimensional microstructure of Fe 3 O 4 -poly vinyl alcohol composite by evaporating the hydrosol under a magnetic field. J Phys Chem. Kim EH, Lee HS, Kwak BK, Kim BK.
Synthesis of ferrofluid with magnetic nanoparticles by sonochemical method for MRI contrast agent. Wu W, He Q, Jiang C. Magnetic iron oxide nanoparticles: synthesis and surface functionalization strategies.
Nanoscale Res Lett. Huber DL. Synthesis, properties, and applications of iron nanoparticles. Vasquez M, Luna C, Morales MP, Sanz R, Serna CJ, Mijangos C. Magnetic nanoparticles: synthesis, ordering and properties. Physica B.
Cai W, Wan J. Facile synthesis of superparamagnetic magnetite nanoparticles in liquid polyols. Xia T, Wang J, Wu C, Meng F, Shi Z, Lian J, Feng J, Meng J.
Novel complex-coprecipitation route to form high quality triethanolamine-coated Fe 3 O 4 nanocrystals: their high saturation magnetizations and excellent water treatment properties.
Cryst Eng Commun. Liu F, Zhu JH, Hou YL, Gao S. Chemical synthesis of magnetic nanocrystals: recent progress. Chin Phy B. Santoyo Salazar J, Perez L, Abril OD, Truong Phuoc L, Ihiawakrim D, Vazquez M, Jean-Marc G, Begin-Colin S, Pourroy G.
Chem Mater. Ge S, Shi X, Sun K, Li C, Uher C, Baker JR, BanaszakHoll MM, Orr BG. Facile hydrothermal synthesis of iron oxide nanoparticles with tunable magnetic properties. J Phys Chem C.
Cai H, An X, Jun C, Li J, Wen S, Li K, Shen M, Zheng L, Zhang G, Shi X. Facile hydrothermal synthesis and surface functionalization of polyethyleneimine-coated iron oxide nanoparticles for biomedical applications. ACS Appl Mater Interfaces.
Khollam YB, Dhage SR, Potdar HS, Deshpande SB, Bakare PP, Kulkarni SD, Date SK. Microwave hydrothermal preparation of submicron-sized spherical magnetite Fe 3 O 4 powders. Mater Lett. Li J, Shi X, Shen M.
Hydrothermal synthesis and functionalization of iron oxide nanoparticles for mr imaging applications. Part Part Syst Charact.
Mohammed Khawja Ansari SA, Eleonora F, Federico Alessandro R, Ilaria S, Monica A, Ornella A, Roberta C, Caterina G, Federico D. Magnetic iron oxide nanoparticles: synthesis, characterization and functionalization for biomedical applications in the central nervous system.
Unfried K, Albrecht C, Klotz LO, Von Mikecz A, Grether Beck S, Schins RPF. Cellular responses to nanoparticles: target structures and mechanisms. Toyokuni S. Iron-induced carcinogenesis: the role of redox regulation.
Free Radic Biol Med. Valko M, Leibfritz D, Moncol J, Cronin MTD, Mazur M, Telser J. Free radicals and antioxidants in normal physiological functions and human disease.
J Biochem Cell Biol. Mahmoudi M, Simchi A, Imani M, Milani AS, Stroeve P. Optimal design and characterization of superparamagnetic iron oxide nanoparticles coated with polyvinyl alcohol for targeted delivery and imaging. J Phys Chem B.
Nel A, Xia T, Madler L, Li N. Toxic potential of materials at the nanolevel. Hafelli UO, Riffle JS, Harris Shekhawat S, Carmichael Baranauskas A, Mark F, Dailey JP, Bardenstein D.
Cell uptake and in vitro toxicity of magnetic nanoparticles suitable for drug delivery. Mol Pharm. Elias A, Tsourkas A. Imaging circulating cells and lymphoid tissues with iron oxide nanoparticles.
Ankamwar B, Lai TC, Huang JH, Liu RS, Hsiao M, Chen CH, Hwu YK. Biocompatibility of Fe 3 O 4 nanoparticles evaluated by in vitro cytotoxicity assays using normal, glia and breast cancer cells. Bulte JWM, Douglas T, Witwer B, Zhang SC, Strable E, Lewis BK, Zywicke H, Mille B, Van Gelderen P, Moskowitz BM, Duncan ID, Frank JA.
Magnetodendrimers allow endosomal magnetic labeling and in vivo tracking of stem cells. Nat Biotechnol. Veranth JM, Kaser EG, Veranth MM, Koch M, Yost GS. Cytokine responses of human lung cells BEAS-2B treated with micron-sized and nanoparticles of metal oxides compared to soil dusts.
Particle Fibre Toxicol. Nanotoxicology Singh N. Stevens RG, Jones DY, Micozzi MS, Taylor PR. Body iron stores and the risk of cancer. Am J Med. CAS Google Scholar. Lacava ZGM, Azevedo RB, Lacava LM, Martins EV, Garcia VAP, Rebula CA, Lemos APC, Sousa MH, Tourinho FA, Morais PC, Da Silva MF.
Toxic effects of ionic magnetic builds in mice. Xie J, Liu G, Eden HS, Ai H, Chen X. Surface-engineered magnetic nanoparticle platforms for cancer imaging and therapy. Mahmoudi M, Hossein Hosseinkhani X, Hosseinkhani M, Boutry S, Simchi A, Shane Journeay W, KarthikeyanSubramani V, Laurent S.
Magnetic resonance imaging tracking of stem cells in vivo using iron oxide nanoparticles as a tool for the advancement of clinical regenerative medicine. Sheng Nan S, Chao W, Zan Zan Z, Long HY, Venkatraman SS, Chuan XZ. Magnetic iron oxide nanoparticles: synthesis and surface coating techniques for biomedical applications.
Chin Phys B. Gregory VL, Kelvin BG, Simon CA, Jamie RL. Transformations of nanomaterials in the environment. Environ Sci Technol. Tanapon P, Thomas CL, Gregoryv L, Andbel L. Chicgoua N, Sabine C, Richard C. Nanoscale metallic iron for environmental remediation: prospects and limitations. Water Air Soil Pollut.
Khin MM, Sreekumaran Nair A, Jagadeesh Babu V, Murugana R, Ramakrishna S. A review on nanomaterials for environmental remediation.
Energy Environ Sci. Khara DG, Annika F, Nanna BH, Eva E, Poul LB, Anders B. Environmental benefits and risks of zero-valent iron nanoparticles nZVI for in situ remediation: risk mitigation or trade-off?
J Contam Hydrol. Wenhui L, Mingyu W, Chunxia X, Dengfeng X, Qiyu C, Xinyue C, Xiaoyan Q, Yuemin L, Xiong X. Synthesis, surface modification, and applications of magnetic iron oxide nanoparticles. J Mater Res. Nan Z, Haining J, Peng Y, Jiaqi N, Farooq MU, Waseem Akram M, Udego IO, Handong L, Xiaobin N.
Surface modification of magnetic iron oxide nanoparticles. Papell SS. Low viscosity magnetic fluid obtained by the colloidal suspension of magnetic particles.
US Patent , Molday RS, MacKenzie D. Immunospecific ferromagnetic iron-dextran reagents for the labeling and magnetic separation of cells. Immunol Methods. Sreeram KJ, Indumathy R, Balachandran UN.
Synthesis of iron oxide nanoparticles using chitosan and starch templates. Trans Metal Chem. Arias JL, Reddy LH, Couvreur P. J Mater Chem.
Rekha MR, Sharma CP. Pullulan as a promising biomaterial for biomedical applications: a perspective. Trends Biomater Artif Organs. Chatterjee J, Haik Y, Chen CJ. Modification and characterization of polystyrene-based magnetic microspheres and comparison with albumin-based magnetic microspheres.
Shi X, Wang SH, Swanson SD, Ge S, Cao Z, Van Antwerp EM, Landmark KL, Baker JR. Dendrimer-functionalized shell-crosslinked iron oxide nanoparticles for in-vivo magnetic resonance imaging of tumors. Adv Mater. Wang SH, Shi S, Antwerp MV, Cao Z, Swanson SD, Bi X, Baker JR. Dendrimer functionalized iron oxide nanoparticles for specific targeting and imaging of cancer cells.
Adv Funct Mater. Aurich K, Schwalbe M, Clement JM, Weitschies W, Buske N. Polyaspartate coated magnetite nanoparticles for biomedical applications. Liang YY, Zhang LM, Jiang W, Li W.
Embedding magnetic nanoparticlesinto polysaccharide-based hydrogels for magnetically assisted bioseparation. Saravanan M, Bhaskar K, Mahajaran G, Pillai KS. Ultrasonically controlled release and targeted delivery of diclofenac sodium via gelatin magnetic microspheres. Int J Pharm. Kim DH, Lee SH, Im KH, Kim KN, Kim KM, Shim IB, Lee MH, Lee YK.
Surface modified magnetite nanoparticles for hypertheremia: preparation, characterization and cytotoxicity studies. Curr Appl Phys. Ma HL, Xu YF, Qi XR, Maitani Y, Nagai T.
Superparamagnetic iron oxide nanoparticles stabilized by alginate: pharmacokinetics, tissue distribution, and applications in detecting liver cancers. Li J, He Y, Sun W, Luo Y, Cai H, Pan Y, Shen M, Xia J, Shi X. Hyaluronic acid-modified hydrothermally synthesized iron oxide nanoparticles for targeted tumor MR imaging.
Hu Y, Wang R, Li J, Ding L, Wang W, Shi X, Shen M. Facile synthesis of lactobionic acid-targeted iron oxide nanoparticles with ultrahigh relaxivity for targeted magnetic resonance imaging of an orthotopic model of human hepatocellular carcinoma.
Viota JL, Delgado AV, Arias JL, Duran JDG. Study of the magnetorheological response of aqueous magnetite suspensions stabilized by acrylic acid polymers. Lin PC, Chou PH, Chen SH, Liao HK, Wang KY, Chen YJ, Lin CC. Ethylene glycol-protected magnetic nanoparticles for a multiplexed immunoassay in human plasma.
Gomez-Lopera SA, Plaza RC, Delgado AV. Gomez-Lopera SA, Arias JL, Gallardo V, Delgado AV. Hamoudeh M, Faraj AA, Canet-Soulas E, Bessueille F, Leonard D, Fessi H. Elaboration of PLLA-based superparamagnetic nanoparticles: characterization, magnetic behaviour study and in vitro relaxivity evaluation.
Kumagai M, Imai Y, Nakamura T, Yamasaki Y, Sekino M, Ueno S, Hanaoka K, Kikuchi K, Nagano T, Kaneko E, Shimokado K, Kataoka K. Iron hydroxide nanoparticles coated with poly ethylene glycol -poly aspartic acid block copolymer as novel magnetic resonance contrast agents for in vivo cancer imaging.
Colloids Surf B. Fan QL, Neoh KG, Kang ET, Shuter B, Wang SC. Solvent-free atom transfer radical polymerization for the preparation of poly poly ethyleneglycol monomethacrylate -grafted Fe 3 O 4 nanoparticles: synthesis, characterization and cellular uptake.
Ren J, Hong HY, Ren TB, Teng XR. Preparation and characterization of magnetic PLA-PEG composite particles. Nasongkla N, Bey E, Ren JM, Ai H, Khemtong C, Guthi JS, Chin SF, Sherry AD, Boothman DA, Gao JM. Multifunctional polymeric micelles as cancer-targeted, MRI-ultrasensitive drug delivery systems.
Nano Lett. Okassa LN, Marchais H, Douziech Eyrolles L, Cohen Jonathan S, Souce M, Dubois P, Chourpa I. Perez-Artacho B, Gallardo V, Ruiz MA, Arias JL. J Nanoparticle Res. Arias JL, Gallardo V, Ruiz MA, Delgado AV. Eur J Pharm Biopharm. Arias JL, Ruiz MA, Gallardo V, Delgado AV.
J Control Release. Santos DP, Ruiz MA, Gallardo V, Zanoni MVB, Arias JL. Flesch C, BourgeatLami E, Mornet S, Duguet E, Delaite C, Dumas P. Synthesis of colloidal superparamagnetic nanocomposites by grafting poly ϵ-caprolactone from the surface of organosilane-modified maghemite nanoparticles.
J Polym Sci Part A Polym Chem. Yang Y, Park SB, Yoon HG, Huh YM, Haam S. Preparation of poly ɛ-caprolactone nanoparticles containing magnetite for magnetic drug carrier. Arias JL, Lopez Viota M, Ruiz MA, Lopez Viota J, Delgado AV. Arias JL, Lopez Viota M, Delgado AV, Ruiz MA.
Yihui X, Rachid S, Suzana Nunes P. Synthesis and characterization of polystyrene coated iron oxide nanoparticles and asymmetric assemblies by phase inversion. J Appl Polym Sci. Song HT, Choi JS, Huh YM, Kim S, Jun YW, Suh JS, Cheon J. Surface modulation of magnetic nanocrystals in the development of highly efficient magnetic resonance probes for intracellular labeling.
J Am Chem Soc. Duan H, Nie S. Cell-penetrating quantum dots based on multivalent and endosome-disrupting surface coatings. Yee C, Kataby G, Ulman A, Prozorov T, White H, King A, Rafailovich M, Sokolov J, Gedanken A. Self-assembled monolayers of alkanesulfonic and -phosphonic acids on amorphous iron oxide nanoparticles.
Sahoo Y, Pizem H, Fried T, Golodnitsky D, Burstein L, Sukenik CN, Markovich G. Zhang C, Wangler B, Morgenstern B, Zentgraf H, Eisenhut M, Untenecker H, Kruger R, Huss R, Seliger C, Semmler W, Kiessling F. Silica- and alkoxysilane-coated ultrasmall superparamagnetic iron oxide particles: a promising tool to label cells for magnetic resonance imaging.
Cho SJ, Jarrett BR, Louie AY, Kauzlarich SM. Gold-coated iron nanoparticles: a novel magnetic resonance agent for T1 and T2 weighted imaging. Li J, Hu Y, Yang J, Wei P, Sun W, Sun M, Shen M, Zhang G, Shi X. Hu Y, Wang R, Wang S, Ding L, Li J, Luo Y, Wang X, Shen M, Shen X.
Sci Rep. Levy L, Sahoo Y, Kim KS, Bergey EJ, Prasad PN. Nanochemistry: synthesis and characterization of multifunctional nanoclinics for biological applications. Qin J, Asempah I, Laurent S, Fornara A, Muller R, Muhammed M.
Injectable superparamagnetic ferrogels for controlled release of hydrophobic drugs. Smith JE, Medley CD, Tang Z, Shangguan D, Lofton C, Tan W. Aptamer-conjugated nanoparticles for the collection and detection of multiple cancer cells.
Anal Chem. Jing Y, Moore LR, Williams PS, Chalmers JJ, Farag SS, Bolwell B. Blood progenitor cell separation from clinical leukapheresis product by magnetic nanoparticle binding and magnetophoresis.
Biotechnol Bioeng. Chiang C, Chen C, Chang L. Purification of recombinant enhanced green fluorescent protein expressed in Escherichia coli with new immobilized metal ion affinity magnetic absorbents.
J Chromatogr B. Fornara A, Johansson P, Petersson K, Gustafsson S, Qin J, Olsson E, Ilver D, Krozer A, Muhammed M, Johansson C. Tailored magnetic nanoparticles for direct and sensitive detection of biomolecules in biological samples.
Budde MD, Frank JA. Magnetic tagging of therapeutic cells for MRI. J Nucl Med. Arbab AS, Liu W, Frank JA. Cellular magnetic resonance imaging: current status and future prospects.
Expert Rev Med Devices. Bhirde A, Xie J, Swierczewska M, Chen X. Nanoparticles for cell labelling. Magro M, Baratella D, Bonaiuto E, de A Roger J, Vianello F. New perspectives on biomedical applications of iron oxide nanoparticles.
Curr Med Chem. Arruebo M, Fernandez Pacheco R, Ibarra MR, Santamaria J. Magnetic nanoparticles for drug delivery. Nano Today. Cao D, He P, Hu N. Electrochemical biosensors utilising electron transfer in heme proteins immobilised on Fe 3 O 4 nanoparticles.
Gao L, Zhuang J, Nie L, Zhang J, Zhang Y, Gu N, Wang T, Jeng J, Yang D, Perrett S, Yan X. Intrinsic peroxidase-like activity of ferromagnetic nanoparticles. Nat Nanotechnol. Rossi LM, Quach AD, Rosenzweig Z. Glucose oxidase-magnetite nanoparticle bioconjugate for glucose sensing.
Anal Bioanal Chem. El-Boubbou K. Magnetic iron oxide nanoparticles as drug carriers: preparation, conjugation and delivery. Magnetic iron oxide nanoparticles as drug carriers: clinical relevance.
McBain SC, Yiu HHP, Dobson J. Magnetic nanoparticles for gene and drug delivery. Int J Nanomed. Shinkai M. Functional magnetic particles for medical application. J Biosci Bioeng. Mornet S, Vasseur S, Grasset F, Veverka P, Goglio G, Demourgues A.
In: Meeting of the European-Materials-Research-Society. Strasbourg: Pergamon-Elsevier Science Ltd; Lanza GM, Winter P, Caruthers S, Schmeider A, Crowder K, Morawski A.
Novel paramagnetic contrast agents for molecular imaging and targeted drug delivery. Curr Pharm Biotechnol. Atri M. New technologies and directed agents for applications of cancer imaging.
J Clin Oncol. Martinkova P, Brtnicky M, Kynicky J, Pohanka M. Iron oxide nanoparticles: innovative tool in cancer diagnosis and therapy. Adv Healthc Mater. Park JH, Von Maltzahn G, Ruoslahti E, Bhatia SN, Sailor MJ. Micellar hybrid nanoparticles for simultaneous magnetofluorescent imaging and drug delivery.
Angew Chem Int Ed Engl. Santra S, Kaittanis C, Grimm J, Perez JM. Quan Q, Xie J, Gao H, Yang M, Zhang F, Liu G, Lin X, Wang A, Eden HS, Zhang G, Chen X.
HSA coated iron oxide nanoparticles as drug delivery vehicles for cancer therapy. Yu MK, Jeong YY, Park J, Park S, Kim JW, Min JJ, Kim K, Jon S. Drug-loaded superparamagnetic iron oxide nanoparticles for combined cancer imaging and therapy in vivo. Angew Chem. Tietze R, Lyer S, Durr S, Alexiou C.
Nanoparticles for cancer therapy using magnetic forces. Pack DW, Hoffman AS, Pun S, Stayton PS. Design and development of polymers for gene delivery. Nat Rev Drug Discov. Neuberger T, Schopf B, Hofmann H, Hofmann M, Von Rechenberg B.
Superparamagnetic nanoparticles for biomedical applications: possibilities and limitations of a new drug delivery system. Subramani K, Hosseinkhani H, Khraisat A, Hosseinkhani M, Pathak Y. Targeting nanoparticles as drug delivery systems for cancer treatment.
Mintzer MA, Simanek EE. Nonviral vectors for gene delivery. Gallia M, Guerrinib A, Cauteruccioa S, Thakarea P, Dovaa D, Orsinic F, Arosioc P, Carraraa C, Sangregoriode C, Lascialfarice A, Maggioni D, Licandro E. Superparamagnetic iron oxide nanoparticles functionalized by peptide nucleic acids.
Sun C, Lee JSH, Zhang MQ. Magnetic nanoparticles in MR imaging and drug delivery. Adv Drug Deliv Rev. Pankhurst QA, Connolly J, Jones SK, Dobson J.
Applications of magnetic nanoparticles in biomedicine. Bhirde A, Guo N, Chen X. Targeted Nanoprobes Reveal Early Time Point Kinetics In Vivo by Time-Resolved MRI.
Weissleder R, Cheng HC, Bogdanova A, Bogdanov A. Magnetically labelled cells can be detected by MR imaging. J Magn Reson Imaging.
Louie AY, Huber MM, Ahrens ET, Rothbacher U, Moats R, Jacobs RE, Fraser SE, Meade TJ. In vivo visualization of gene expression using magnetic resonance imaging. Xie J, Huang J, Li X, Sun S, Chen X.
Iron oxide nanoparticle platform for biomedical applications. Mikhaylov G, Mikac U, Magaeva AA, Itin VI, Naiden EP, Psakhye I, Babes L, Reinheckel T, Peters C, Zeiser R, Bogyo M, Turk V, Psakhye SG, Turk B, Vasiljeva O.
Ferri-liposomes as an MRI-visible drug-delivery system for targeting tumours and their microenvironment. Xavier M, Montet-Abou K, Reynolds F, Weissleder R, Josephson L.
Nanoparticle imaging of integrins on tumor cells. Chakravarty R, Chakraborty S, Dash A. Molecular imaging of breast cancer: role of RGD peptides. Mini Rev Med Chem. Rosen JE, Chan L, Shieh DB, Gu FX. Iron oxide nanoparticles for targeted cancer imaging and diagnostics.
Lewin M, Carlesso N, Ching-Hsuan T, Xiao-Wu T, David C, David TS, Weissleder R. Tat peptide-derivatized magnetic nanoparticles allow in vivo tracking and recovery of progenitor cells. Jasmin GTS, Torres de Souza G, Ruy Andrade L, Rosado-de-Castro PH, Mendez-Otero R, Campos de Carvalho AC. Tracking stem cells with superparamagnetic iron oxide nanoparticles: perspectives and considerations.
Rotating machines, where the direction of the magnetic field can change, gain no benefit from grain-oriented steel. A family of specialized alloys exists for magnetic core applications.
Examples are mu-metal , permalloy , and supermalloy. They can be manufactured as stampings or as long ribbons for tape wound cores. Some alloys, e. Sendust , are manufactured as powder and sintered to shape.
Many materials require careful heat treatment to reach their magnetic properties, and lose them when subjected to mechanical or thermal abuse.
For example, the permeability of mu-metal increases about 40 times after annealing in hydrogen atmosphere in a magnetic field; subsequent sharper bends disrupt its grain alignment, leading to localized loss of permeability; this can be regained by repeating the annealing step.
Amorphous metal is a variety of alloys e. Metglas that are non-crystalline or glassy. These are being used to create high-efficiency transformers.
The materials can be highly responsive to magnetic fields for low hysteresis losses, and they can also have lower conductivity to reduce eddy current losses.
Power utilities are currently making widespread use of these transformers for new installations. Powder cores consist of metal grains mixed with a suitable organic or inorganic binder, and pressed to desired density.
Higher density is achieved with higher pressure and lower amount of binder. Higher density cores have higher permeability, but lower resistance and therefore higher losses due to eddy currents. Finer particles allow operation at higher frequencies, as the eddy currents are mostly restricted to within the individual grains.
Coating of the particles with an insulating layer, or their separation with a thin layer of a binder, lowers the eddy current losses. Presence of larger particles can degrade high-frequency performance.
Permeability is influenced by the spacing between the grains, which form distributed air gap; the less gap, the higher permeability and the less-soft saturation. Due to large difference of densities, even a small amount of binder, weight-wise, can significantly increase the volume and therefore intergrain spacing.
Lower permeability materials are better suited for higher frequencies, due to balancing of core and winding losses. The surface of the particles is often oxidized and coated with a phosphate layer, to provide them with mutual electrical insulation.
Powdered iron is the cheapest material. It has higher core loss than the more advanced alloys, but this can be compensated for by making the core bigger; it is advantageous where cost is more important than mass and size. Saturation flux of about 1 to 1. Relatively high hysteresis and eddy current loss, operation limited to lower frequencies approx.
below kHz. Used in energy storage inductors, DC output chokes, differential mode chokes, triac regulator chokes, chokes for power factor correction, resonant inductors, and pulse and flyback transformers. The binder used is usually epoxy or other organic resin, susceptible to thermal aging.
At higher temperatures, typically above °C, the binder degrades and the core magnetic properties may change. With more heat-resistant binders the cores can be used up to °C. Iron powder cores are most commonly available as toroids.
Sometimes as E, EI, and rods or blocks, used primarily in high-power and high-current parts. Powdered cores made of carbonyl iron , a highly pure iron, have high stability of parameters across a wide range of temperatures and magnetic flux levels, with excellent Q factors between 50 kHz and MHz.
Carbonyl iron powders are basically constituted of micrometer-size spheres of iron coated in a thin layer of electrical insulation. This is equivalent to a microscopic laminated magnetic circuit see silicon steel, above , hence reducing the eddy currents , particularly at very high frequencies.
Carbonyl iron has lower losses than hydrogen-reduced iron, but also lower permeability. A popular application of carbonyl iron-based magnetic cores is in high-frequency and broadband inductors and transformers , especially higher power ones.
The as-prepared particles, "E-type"and have onion-like skin, with concentric shells separated with a gap. They contain significant amount of carbon. They behave as much smaller than what their outer size would suggest.
The "C-type" particles can be prepared by heating the E-type ones in hydrogen atmosphere at °C for prolonged time, resulting in carbon-free powders. Powdered cores made of hydrogen reduced iron have higher permeability but lower Q than carbonyl iron.
They are used mostly for electromagnetic interference filters and low-frequency chokes, mainly in switched-mode power supplies.
Very low core loss, low hysteresis and therefore low signal distortion. Very good temperature stability. High cost. Maximum saturation flux of about 0. Used in high-Q filters, resonant circuits, loading coils, transformers, chokes, etc. The material was first introduced in , used in loading coils to compensate capacitance in long telephone lines.
It is usable up to about kHz to 1 MHz, depending on vendor. Underground lines, where temperature is more stable, tend to use ferrite cores due to their lower cost. High energy storage, saturation flux density of about 1.
Residual flux density near zero. Used in applications with high DC current bias line noise filters, or inductors in switching regulators or where low residual flux density is needed e. pulse and flyback transformers, the high saturation is suitable for unipolar drive , especially where space is constrained.
The material is usable up to about kHz. Core losses higher than MPP. Very low magnetostriction , makes low audio noise. Loses inductance with increasing temperature, unlike the other materials; can be exploited by combining with other materials as a composite core, for temperature compensation.
Saturation flux of about 1 tesla. Good temperature stability. Used in switching power supplies, pulse and flyback transformers, in-line noise filters, swing chokes, and in filters in phase-fired controllers e.
dimmers where low acoustic noise is important. Absence of nickel results in easier processing of the material and its lower cost than both high-flux and MPP. The material was invented in Japan in A nanocrystalline alloy of a standard iron-boron-silicon alloy, with addition of smaller amounts of copper and niobium.
The grain size of the powder reaches down to 10— nanometers. The material has very good performance at lower frequencies. It is used in chokes for inverters and in high power applications. It is available under names like e.
Nanoperm, Vitroperm, Hitperm and Finemet. Ferrite ceramics are used for high-frequency applications. The ferrite materials can be engineered with a wide range of parameters. As ceramics, they are essentially insulators, which prevents eddy currents, although losses such as hysteresis losses can still occur.
A coil not containing a magnetic core is called an air core. This includes coils wound on a plastic or ceramic form in addition to those made of stiff wire that are self-supporting and have air inside them.
Air core coils generally have a much lower inductance than similarly sized ferromagnetic core coils, but are used in radio frequency circuits to prevent energy losses called core losses that occur in magnetic cores.
The absence of normal core losses permits a higher Q factor , so air core coils are used in high frequency resonant circuits , such as up to a few megahertz. However, losses such as proximity effect and dielectric losses are still present. Air cores are also used when field strengths above around 2 Tesla are required as they are not subject to saturation.
Most commonly made of ferrite or powdered iron, and used in radios especially for tuning an inductor. The coil is wound around the rod, or a coil form with the rod inside.
Moving the rod in or out of the coil changes the flux through the coil, and can be used to adjust the inductance. Often the rod is threaded to allow adjustment with a screwdriver. In radio circuits, a blob of wax or resin is used once the inductor has been tuned to prevent the core from moving.
The presence of the high permeability core increases the inductance , but the magnetic field lines must still pass through the air from one end of the rod to the other.
The air path ensures that the inductor remains linear. In this type of inductor radiation occurs at the end of the rod and electromagnetic interference may be a problem in some circumstances. Like a cylindrical rod but is square, rarely used on its own.
This type of core is most likely to be found in car ignition coils. U and C -shaped cores are used with I or another C or U core to make a square closed core, the simplest closed core shape.
Windings may be put on one or both legs of the core. E-shaped core are more symmetric solutions to form a closed magnetic system. Most of the time, the electric circuit is wound around the center leg, whose section area is twice that of each individual outer leg.
In 3-phase transformer cores, the legs are of equal size, and all three legs are wound. Sheets of suitable iron stamped out in shapes like the sans-serif letters "E" and "I", are stacked with the "I" against the open end of the "E" to form a 3-legged structure.
Coils can be wound around any leg, but usually the center leg is used. This type of core is frequently used for power transformers, autotransformers, and inductors. Again used for iron cores.
Similar to using an "E" and "I" together, a pair of "E" cores will accommodate a larger coil former and can produce a larger inductor or transformer.
If an air gap is required, the centre leg of the "E" is shortened so that the air gap sits in the middle of the coil to minimize fringing and reduce electromagnetic interference. A planar core consists of two flat pieces of magnetic material, one above and one below the coil.
It is typically used with a flat coil that is part of a printed circuit board. This design is excellent for mass production and allows a high power , small volume transformer to be constructed for low cost.
It is not as ideal as either a pot core or toroidal core [ citation needed ] but costs less to produce. Usually ferrite or similar. This is used for inductors and transformers. The shape of a pot core is round with an internal hollow that almost completely encloses the coil.
Usually a pot core is made in two halves which fit together around a coil former bobbin. This design of core has a shielding effect, preventing radiation and reducing electromagnetic interference.
This design is based on a toroid the same shape as a doughnut. The coil is wound through the hole in the torus and around the outside.
The magnetlc iron-oxide nanoparticles Iron in magnetic applications Promoting nutrient absorption with triethanolammonium oleate were produced. The specimens were divided into two groups: Eco-friendly office supplies top NPs extracted from the supernatant applicattions the bottom NPs nanoparticles sedimented at applicatiosn bottom of the flask Iron in magnetic applications centrifugationrespectively. The XRD and Mössbauer studies revealed a presence of the Fe 3 O 4 phase in both types of nanoparticles. Furthermore, the formation of maghemite layer on the surface of nanoparticles was observed. Average particle sizes determined from TEM and XRD studies were lower than the superparamagnetic limit for the magnetite NPs. For glycerol dispersions of both types of NPs, when exposed to kHz external magnetic field, a significant heat release was observed.
Darin die ganze Sache.
Ich denke, dass Sie sich irren. Ich kann die Position verteidigen. Schreiben Sie mir in PM.
Sie sind absolut recht. Darin ist etwas auch mich ich denke, dass es der gute Gedanke ist.
Ja, wirklich. Es war und mit mir.
Welche gute Gesprächspartner:)