Steven D. Odor-reducing treatmentsUniversity of Chitowan Health Science Barre exercises Charles RiccioUniversity of Tennessee Health Science Center Ehalth GoulartUniversity of Chitosan for bone health Health Science Boost energy before workouts Joel BumgardnerUniversity healt Memphis Xi Lin JingUniversity of Tennessee Health Science Center Petros KonofaosUniversity of Tennessee Health Science CChitosan.
The current halth of care for bone reconstruction, whether secondary to injury, nonunion, cancer Pre-game nutrition for tennis, or idiopathic bone hfalth, is autologous bone grafting.
Chitosan for bone health to autograft and Chitosan for bone health bone substitutes Cihtosan being researched are synthetic Advanced skin rejuvenation natural graft materials that Advanced skin rejuvenation able to guide bone regeneration.
One promising material currently fir researched cor chitosan, a highly versatile, naturally occurring polysaccharide, derived from the exoskeleton of arthropods that is comprised of glucosamine and N-acetylglucosamine.
Research on chitosan as a bone scaffold has been promising. Chitosan is efficacious in bone regeneration due to its lack of immunogenicity, its biodegradability, and its physiologic features.
Additionally, current studies have shown that it can provide the additional benefit of a local drug delivery system.
As research in the area of bone scaffolding continues to grow, further clinical research on chitosan in conjunction with growth factors, proteins, and alloplastic materials will likely be at the forefront.
Kozusko, S. Chitosan as a bone scaffold biomaterial. Journal of Craniofacial Surgery29 7 Advanced Search. Home About FAQ My Account Accessibility Statement. Privacy Copyright. Skip to main content Home About About My Account. Faculty Publications.
Authors Steven D. Abstract The current standard of care for bone reconstruction, whether secondary to injury, nonunion, cancer resection, or idiopathic bone loss, is autologous bone grafting. Publication Title Journal of Craniofacial Surgery. Recommended Citation Kozusko, S.
Link to Full Text. DOWNLOADS Since May 09, Search Enter search terms:. in this series in this repository across all repositories. Libraries McWherter Library Music Library Health Sciences Library Lambuth Library.
Elsevier - Digital Commons.
: Chitosan for bone healthAuthor Contributions | Chitosan is efficacious in bone regeneration due to its lack of immunogenicity, its biodegradability, and its physiologic features. Additionally, current studies have shown that it can provide the additional benefit of a local drug delivery system. As research in the area of bone scaffolding continues to grow, further clinical research on chitosan in conjunction with growth factors, proteins, and alloplastic materials will likely be at the forefront. Kozusko, S. Chitosan as a bone scaffold biomaterial. Journal of Craniofacial Surgery , 29 7 , Advanced Search. Home About FAQ My Account Accessibility Statement. Privacy Copyright. Zhang, J. B , , 2 , DOI: To request permission to reproduce material from this article, please go to the Copyright Clearance Center request page. If you are an author contributing to an RSC publication, you do not need to request permission provided correct acknowledgement is given. If you are the author of this article, you do not need to request permission to reproduce figures and diagrams provided correct acknowledgement is given. Read more about how to correctly acknowledge RSC content. Fetching data from CrossRef. This may take some time to load. Loading related content. Jump to main content. Jump to site search. You do not have JavaScript enabled. Please enable JavaScript to access the full features of the site or access our non-JavaScript page. Issue 21, From the journal: Journal of Materials Chemistry B. The quality assessment of the study used a checklist from Joanna Briggs Institute for experimental study. Result: We included 18 experimental studies, both in vivo and in vitro study—the in vivo study used animal subjects such as mice, goats and rabbits. The studies mostly used chitosan combined with other biomaterials such as demineralized bone matrix DBM , genipin GP , sodium alginate SA , resveratrol Res , polycaprolactone PCL and collagen, growth factor and stem cells such as bone morphogenic protein-2 BMP-2 , dental pulp stem cell DPSC , and human umbilical cord mesenchymal stem cells hUCMSC. Conclusion: Chitosan is a natural polymer with promising osteoconductive, osteoinductive and osteo-integrative effects in bone regeneration. Chitosan utilization for bone scaffolds combined with other biomaterials, growth factors, or stem cells gives better bone regeneration results than chitosan alone. Division of Plastic, Reconstructive, and Aesthetic Surgery, Department of Surgery, Faculty of Medicine, Udayana University, Sanglah General Hospital, Bali, Indonesia. Khazaal H, Helman J. Narrative review: Craniofacial bone regeneration-where are we now? Front Oral Maxillofac Med. Ghosh R, Gopalkrishnan K. Facial fractures. J Craniofac Surg. Wiargitha IK, Wiradana AA. Patterns of fracture site and management of maxillofacial trauma cases in the Department of Trauma and Acute Care Surgery in Sanglah general hospital. JBN Jurnal Bedah Nasional. Pandini FE, Kubo FM, Plepis AM, Martins VD, da Cunha MR, Silva VR, et al. In vivo study of nasal bone reconstruction with collagen, elastin and chitosan membranes in abstainer and alcoholic rats. Polymers Basel. Ghassemi T, Shahroodi A, Ebrahimzadeh MH, Mousavian A, Movaffagh J, Moradi A. Current concepts in scaffolding for bone tissue engineering. Arch Bone Jt Surg. Thrivikraman G, Athirasala A, Twohig C, Boda SK, Bertassoni LE. Biomaterials for craniofacial bone regeneration. Dent Clin North Am. Kozusko SD, Riccio C, Goulart M, Bumgardner J, Jing XL, Konofaos P. Chitosan as a bone scaffold biomaterial. Alidadi S, Oryan A, Bigham-Sadegh A, Moshiri A. Comparative study on the healing potential of chitosan, polymethylmethacrylate, and demineralized bone matrix in radial bone defects of rat. Carbohydr Polym. Bakopoulou A, Georgopoulou Α, Grivas I, Bekiari C, Prymak O, Loza Κ, et al. Dent Mater. Bangun K, Sukasah CL, Dilogo IH, Indrani DJ, Siregar NC, Pandelaki J, et al. Cleft Palate Craniofac. Hu X, Zheng S, Zhang R, Wang Y, Jiao Z, Li W, et al. Biomater Adv. Singh YP, Dasgupta S, Bhaskar R, Agrawal AK. Monetite addition into gelatin based freeze-dried scaffolds for improved mechanical and osteogenic properties. Biomed Mater. Gani A, Yulianty R, Supiaty S, Rusdy M, Asri GD, Satya DE, et al. Effectiveness of combination of chitosan gel and hydroxyapatite from crabs shells Portunus pelagicus waste as bonegraft on periodontal network regeneration through IL-1 and BMP-2 analysis. Int J Biomater. Gao H, Ge K, Xu Y, Wang Y, Lu M, Wei Y, et al. Dent Mater J. |
REVIEW article | This is an agreement with the previous studies that showed an increase in bone mineral density by using recombinant OPG protein in rodents Capparelli et al. Chitosan has been described as a potent wound-healing accelerator Di Martino et al. Moreover, the chitosan gel enables the defect to heal more rapidly than an empty, unfilled defect, which was filled with just osteoid tissue at 6 weeks. The authors suggested that the earlier and more bone formed in chitosan gel and OPG-chitosan gel filled defects were resulted from the action of the hydrophilic surface of chitosan gel that promotes cell adhesion and supports the attachment and proliferation of bone-forming osteoblast cells as well as formation of a mineralized bone matrix. Additionally, as a result of the advantage of hydrogels in chitosan that can easily adopt the geometry of the defect that they occupy which has a role in stability that supports the cell differentiation and proliferation as reported by Levengood and Zhang In a bony defect, the most intense cellular reaction occurs during the first 6 weeks. In another word, the defect is first bridged by a trabecular framework consisting of primitive woven bone. Following, there is a reduction in the numbers of cells in these areas, as well as an increase in calcium deposition as reported by Gehrke The quantity of osteoblasts is significantly changed, and the bone remodeling occur between 30 and 45 days Piattelli et al. Moreover, the defect closure and the new bone area ratio gradually increased with the healing time, but these parameters did not differ significantly between weeks 2 and 4 or between weeks 8 and 12 Sohn et al. An observation period of at least 12 weeks was recommended by Bodde et al. Furthermore, Seo and Kim stated that the volume analysis of rabbit calvarial defects and bone grafts using CT can be done after 2 and 8 weeks. In point of fact, the HR-pQCT results were corresponding to the histological results of our published literature in Jayash et al. Likewise, in the current study, the part-comparison map and histomorphometrical analyses results confirmed the changes in microstructure between the reference bone and the newly formed bone after surgery at 6 and 12 weeks. They showed that the rate of the bone healing was higher in the OPG-chitosan gel and chitosan gel than in the unfilled defect. Furthermore, the defects healed completely at 12 weeks in the OPG-chitosan gel implanted defects and partially, more prominent at chitosan gel than the control defects. Although both control and chitosan gel groups both exhibited partially defects closure at 12 weeks, chitosan gel group showed higher mean percentages of osteoid with no significant results in bone marrow with OPG-chitosan gel group at 12 weeks which indicated more newly formed bone quantity in chitosan gel than the control group. This is an evidence of the ability of chitosan gel to play a supportive role in the early repair process and provides a favorable surface for osteoprogenitor cell attachment. To sum up, the chitosan gel was capable of regenerating new bone which is beneficial in tissue engineering applications, and the HR-pQCT analysis was an efficient method to evaluate the newly formed bone and it was as effective as the histomorphometry bone analysis. Potentially, these findings could be translated into clinical use and would be of great interest to the vision scientists, researchers, clinicians, and trainees. The chitosan-based gel promoted the cell migration, proliferation, and differentiation in support of tissue regeneration by demonstrating a significant bone quantity and quality in a cranial critical size defect in a rabbit model compared to unfilled surgical defect. Correspondingly, the OPG enhanced the chitosan gel in bone regeneration. This study has revealed that chitosan-based gel is potential candidates for bone tissue engineering. The animal study was reviewed and approved by Institutional Animal Care and Use Committee FOM IACUC. NA-N and NI reviewed drafts of the paper and contributed in analysis tools. This study was also supported by a grant from a research grant PGA from University of Malaya and UM. The authors declare that the research was conducted in the absence of any commercial or financial relationships that could be construed as a potential conflict of interest. Acar, A. Bone regeneration by low-level laser therapy and low-intensity pulsed ultrasound therapy in the rabbit calvarium. Oral Biol. PubMed Abstract CrossRef Full Text Google Scholar. Ahsan, A. Thermosensitive chitosan-based injectable hydrogel as an efficient anticancer drug carrier. ACS omega 5, — Al-Namnam, N. Recent advances in bone graft substitute for oral and maxillofacial applications:A review. Google Scholar. Alyessary, A. Effect of piezoelectric sutural ostectomies on accelerated bone-borne sutural expansion. Oral Maxillofac. An, Y. Animal models in orthopaedic research. Boca Rato, FL; CRC Press. Aycan, D. Development of pH-responsive chitosan-based hydrogel modified with bone ash for controlled release of amoxicillin. Baek, Y. Chitin-fibroin-hydroxyapatite membrane for guided bone regeneration: micro-computed tomography evaluation in a rat model. Baiker, M. Automated bone volume and thickness measurements in small animal whole-body microCT data. Imaging Biol. Bodde, E. Closing capacity of segmental radius defects in rabbits. Bumgardner, J. Contact angle, protein adsorption and osteoblast precursor cell attachment to chitosan coatings bonded to titanium. Capparelli, C. Sustained antiresorptive effects after a single treatment with human recombinant osteoprotegerin OPG : a pharmacodynamic and pharmacokinetic analysis in rats. Bone Miner Res. Chang, H. A novel chitosan-γPGA polyelectrolyte complex hydrogel promotes early new bone formation in the alveolar socket following tooth extraction. PLoS one 9, e Charan, J. How to calculate sample size for different study designs in medical research? Indian J. Di Martino, A. Chitosan: a versatile biopolymer for orthopaedic tissue-engineering. Biomaterials 26, — Gehrke, S. Analysis of bone tissue healing around titanium implant surface treated with tio sandblasted after three and six weeks used different histological methods-a study in rabbits. Trials , 1—7. CrossRef Full Text Google Scholar. Graber, L. Orthodontics: Current principles and techniques. New York, NY: Elsevier Health Sciences. He, Y. Ectopic osteogenesis and scaffold biodegradation of nano-hydroxyapatite-chitosan in a rat model. PLoS one 10, e Hu, J. A thermo-degradable hydrogel with light-tunable degradation and drug release. Biomaterials , — Isaksson, S. Aspects of bone healing and bone substitute incorporation. An experimental study in rabbit skull bone defects. Swed Dent J. Jayash, S. Formulation and in vitro and in vivo evaluation of a new osteoprotegerin-chitosan gel for bone tissue regeneration. In vitro evaluation of osteoprotegerin in chitosan for potential bone defect applications. Local application of osteoprotegerin-chitosan gel in critical-sized defects in a rabbit model. Osteoprotegerin OPG pathways in bone diseases and its application in therapeutic perspectives. Biointerface Res. Lee, J. Injectable hydrogels delivering therapeutic agents for disease treatment and tissue engineering. Levengood, S. Chitosan-based scaffolds for bone tissue engineering. Since new bone was forming in the vicinity of the IP but not in the IP itself a different approach than originally planned was taken to analyze the samples, looking at a larger volume around the IP rather than the IP itself. New bone appears darker than old bone on micro-CT images, therefore, these can be separated using a defined grayscale value. However, for our study the IP had similar grayscale value distribution as new bone. Therefore, the IP volume was subtracted and TBV outside of the IP was calculated see Fig. Change in total bone volume in a defined part of rat mandible as a function of time. Graphs represent mean±SEM. To extend these findings, verify the model and examine how acetylation of the chitosan polymer affected the results, another experiment was performed. TBV was calculated for each group Fig. However, new bone growth was not observed in the IP itself but rather in surrounding bone tissue. Three-dimensional representations produced from the X-ray micro-CT data showed new bone growth formed as an outgrowth from the periosteum, covering the surface of the bone, located along particular areas of the mandible. This finding suggests that it is the biomechanical weakening in the structure of the mandible that stimulates the osteogenesis observed in the present material. The rats cannot shield the mandible as they need to feed, therefore, there is a greater need to compensate for loss of mechanical strength or stability produced by the drill hole. The important finding here is that this response was stronger and faster in the IP group. It can be speculated that the chitosan is increasing or stimulating a natural response to the structural weakening of the mandible and that the acetyl groups in the chitosan polymer play a role. Chitinases cleave chitin and partly deacetylated chitin, but not fully deacetylated chitosan. Chitinases, including human chitinases Chitotriosidase need at least two consecutive acetylated glucosamine units AA on the partly deacetylated chitin polymer in order to cleave it into oligomers, resulting in AA at the reducing end of each oligomer product [ 15—17 ]. Chitinases and chitinase like proteins are highly expressed during inflammation [ 18 ], e. by immune cells such as PMN leukocytes. The degree of deacetylation of chitooligosaccharides controls the binding affinity to the chitinase like protein YKL and the effect on human chondrocyte proliferation [ 19 ]. Fully deacetylated hexamer binds very poorly to YKL [ 19 ]. Chitosan having different degree of deacetylation has been shown to have different biological activity, where lower degree of deacetylation higher acetylation; more chitin like has the tendency to have stronger bioactivity. When comparing the effect of fully acetylated and deacetylated chitooligosaccharides Hexamers on osteogenic differentiation of bone-marrow derived human mesenchymal stem cells, the effect of chitooligosaccharides was dependent on the acetylation degree, with significantly stronger effects with chitin-derived hexamers N -acetyl chitohexaose; fully acetylated than with chitosan hexamers chitohexaose; fully deacetylated [ 20 ]. Therefore, it is possible that the IP is acting as a reservoir, allowing slow release of chitooligosaccharides which can diffuse from the IP and stimulate new bone growth in the periphery of the IP [ 21 ]. When we compare our results to the literature it is surprising that we do not see any activity in the IP itself. However, the duration of this study might not be long enough to see osteogenesis in the IP. Furthermore, the IP is non-load bearing, and so does not impart the mechanical signal needed for active bone formation and remodeling. Other published studies generally allow more time to pass before checking for bone growth [ 12 ]. One reason might be that this would generally get overlooked by researchers that are focusing on the IP itself. Another reason might be that the rat mandible model is especially sensitive to mechanical stress induced bone remodeling since the mandible cannot be shielded, even after an injury, because the animals need to feed. On the other hand, it might also be because at later time points this bone has been reabsorbed. At the time points that we selected, not enough time has subsided for the IP to be replaced by new bone, therefore, compensations need to be made in other areas of the mandible. As time passes bone might replace the IP and then the reinforcements around the bone defect might no longer be needed, perhaps causing resorption of the extra bone generated at earlier time points. The answer to these speculations can only be acquired by additional studies. For clinical use in bone defects, the results point to the option of developing a chitosan containing scaffold or a composite IP that takes up mechanical load within the defect, and facilitates cell ingrowth, in order to stimulate osteogenesis within the defect. The results of the present study suggest that the bone regenerating effect of mechanical stimulation of the bone tissue is increased by chitosan or chitooligosaccharide containing IPs. The effect on bone regeneration also depends on the degree of deacetylation of the chitosan used. Use of chitosan-calcium phosphate soft composites as bone void fillers is not feasible for short-term stimulation of osteogenesis within a void. For restoration of function, a soft composite may still be feasible as a reservoir of chitosan, accelerating osteogenesis in the vicinity of the IP site. We thank Jóhannes Björnsson and Kristrún Ólafsdóttir at the Department of Pathology, Landspítali University Hospital, Reykjavík, Iceland, for assistance with microscopical sectioning, staining and analysis of histological samples. This work was supported by the Technology Development Fund, managed by the Icelandic Centre for Research [RAN ]. Oryan A , Alidadi S , Moshiri A et al. Bone regenerative medicine: classic options, novel strategies, and future directions. J Orthop Surg Res ; 9 : Google Scholar. Dimitriou R , Jones E , McGonagle D et al. Bone regeneration: current concepts and future directions. BMC Med ; 9 : Dennis SC , Berkland CJ , Bonewald LF et al. Endochondral ossification for enhancing bone regeneration: converging native extracellular matrix biomaterials and developmental engineering in vivo. Tissue Eng Part B Rev ; 21 : — Sheikh Z , Javaid MA , Hamdan N et al. Bone regeneration using bone morphogenetic proteins and various biomaterial carriers. Materials Basel ; 8 : — Carragee EJ , Hurwitz EL , Weiner BK. A critical review of recombinant human bone morphogenetic protein-2 trials in spinal surgery: emerging safety concerns and lessons learned. Spine J ; 11 : — Collignon AM , Lesieur J , Vacher C et al. Strategies developed to induce, direct, and potentiate bone healing. Front Physiol ; 8 : Venkatesan J , Vinodhini PA , Sudha PN et al. Chitin and chitosan composites for bone tissue regeneration. Adv Food Nutr Res ; 73 : 59 — Younes I , Rinaudo M. Chitin and chitosan preparation from marine sources. Structure, properties and applications. Mar Drugs ; 13 : — Klokkevold PR , Vandemark L , Kenney EB et al. Osteogenesis enhanced by chitosan poly-N-acetyl glucosaminoglycan in vitro. J Periodontol ; 67 : — 5. Biodegradable and biocompatible high elastic chitosan scaffold is cell-friendly both in vitro and in vivo. Oncotarget ; 8 : — Ho MH , Yao CJ , Liao MH et al. Int J Nanomedicine ; 10 : — Levengood SL , Zhang M. Chitosan-based scaffolds for bone tissue engineering. J Mater Chem B Mater Biol Med ; 2 : — Oryan A , Sahvieh S. Effectiveness of chitosan scaffold in skin, bone and cartilage healing. Int J Biol Macromol ; : — Eliaz N , Metoki N. Calcium phosphate bioceramics: a review of their history, structure, properties, coating technologies and biomedical applications. Materials Basel ; 10 : Eide KB , Norberg AL , Heggset EB et al. Human chitotriosidase-catalyzed hydrolysis of chitosan. Biochemistry ; 51 : — Bahrke S , Einarsson JM , Gislason J et al. Sequence analysis of chitooligosaccharides by matrix-assisted laser desorption ionization postsource decay mass spectrometry. Biomacromolecules ; 3 : — Haebel S , Bahrke S , Peter MG. Quantitative sequencing of complex mixtures of heterochitooligosaccharides by vMALDI-linear ion trap mass spectrometry. Anal Chem ; 79 : — Kzhyshkowska J , Gratchev A , Goerdt S. Human chitinases and chitinase-like proteins as indicators for inflammation and cancer. Biomarker Insights ; 2 : — Kozusko, Charles Riccio, Micheline Goulart, Joel Bumgardner, Xi Lin Jing, Petros Konofaos. Overview Fingerprint. Abstract The current standard of care for bone reconstruction, whether secondary to injury, nonunion, cancer resection, or idiopathic bone loss, is autologous bone grafting. Keywords Biomaterial Chitosan Osteoconduction Reconstruction Scaffold. ASJC Scopus subject areas Surgery Otorhinolaryngology. Access to Document Link to publication in Scopus. Link to the citations in Scopus. Fingerprint Dive into the research topics of 'Chitosan as a bone scaffold biomaterial'. Together they form a unique fingerprint. View full fingerprint. |
Frontiers | Chitosan-Based Biomaterial Scaffolds for the Repair of Infected Bone Defects | Naringin was also dropped directly into TiO 2 nanotubes, which were then subsequently covered with chitosan layers. There was a long-term release of naringin from the system. In vitro biological tests on osteoblasts revealed that the cells were viable and alkaline phosphatase activity was increased. In addition, Alizarin red S staining tests detected the development of mineralized nodules [81]. On the Ti substrate, TiO 2 nanotubes carrying a gentamicin drug mixture were deposited. Furthermore, a combination of alginate and chitosan was utilized to cover the TiO 2 —gentamicin composite using a self-assembly method. The osteoblasts obtained from newborn rat calvaria bones were subjected to biological tests. Cells were viable and proliferating with the produced composite material. In addition, there was an increase in alkaline phosphatase activity. Aside from that, exceptional antibacterial activity was observed against Staphylococcus aureus and Escherichia coli [95]. Melatonin-loaded TiO 2 nanotubes were synthesized by Lai et al. Subsequently, using a spin-based layer-by-layer approach, chitosan and gelatin-based films were applied. Further, an in vitro cell interaction study using mesenchymal stem cells showed that the fabricated biocomposite has exceptional osteogenic potential [96]. In another research study, Chen et al. Further investigation demonstrates the antibacterial, anticancer, and osteogenic properties of the produced biocomposite [89]. Zinc was utilized as a trace element in composites for bone tissue engineering applications since it improves bone density and minimizes bone loss. The nanocomposites attain mechanical strength of This composite demonstrates antibacterial efficacy against Gram-negative and Gram-positive bacterial strains including Escherichia coli , Lysinibacillus fusiformis , and Bacillus cereus. The cytotoxicity behaviour was determined by an in vitro proliferation experiment with MG cells. The findings indicate that the nontoxic substances are safe to be used in bone tissue engineering applications [69]. The bioactivity of the composites was evaluated by simulation studies of body fluids. Huang et al. The nanocomposites were coated on titanium substrates and showed antibacterial efficacy against bacterial species of Escherichia coli and Staphylococcus aureus. The in vitro response of the nanocomposites was evaluated using rat bone marrow stromal cells. The results demonstrate that the composites had higher proliferation rate following the addition of the zinc trace element. Furthermore, the data indicate that cytotoxicity was reduced and also that the enzymatic alkaline phosphatase assay has significant activity [29]. The alkaline phosphatase assay was performed using mouse embryonic fibroblasts and the increased activity on the 21st day demonstrates a favourable environment for cell development and differentiation. The in vivo investigation was conducted on two male rabbits by making two 25 mm longitudinal incisions on the right and left dorsum. The bioimplants were placed and examined after four weeks of surgery. On the 14th day, histological studies revealed that cells had infiltrated into the holes. Meanwhile, degradation of the scaffold matrix was observed and represented as black spots in Figure 3 []. Figure 3 was reproduced from [] , © J. Forero et al. Carbon nanotubes have distinct physical, chemical, and optical properties that enable new bioengineering applications, notably in the development of natural bone tissue repair and replacement scaffolds. Carbon nanoparticles can provide a chemically and physically comparable microenvironment to that of the natural extracellular matrix, containing healing and stimulating components necessary for bone repair, making them a potential option for bone tissue regeneration. As carbon nanotubes are combined with natural polymers, such as chitosan and collagen, they develop an interlinked molecular framework with load-bearing applications and have superior mechanical qualities and biological advantages [57]. A further study reported that carbon nanotubes combined with synthetic polymers of poly-ʟ-lactide acid, polylactic acid, polyhydroxyethylmethacrylate, and polyethylene glycol were used in composite preparation for bone tissue engineering applications [10]. To create a fibrous scaffold for bone tissue regeneration, zein and chitosan were combined with polyurethane and functionalized multiwalled carbon nanotubes. According to the findings in vitro, the combination of scaffolds promotes fast cell-to-cell contact, which boosts the regeneration impact on pre-osteoblast MC3T3-E1 cell proliferation, growth, and differentiation [94]. Scanning electron microscopy SEM examination verified the porosity of the scaffolds in the — µm range. The incorporation of chitosan into poly 3-hydroxybutyrate composites generates a hydrophilic environment which promotes cell motility, adhesion, and protein adsorption. Furthermore, the mixture enhances the surface roughness of the composites, stimulating bone cells to attach to the surface []. The XRD patterns of the lyophilized scaffolds reveal the presence of collagen, chitosan, and hydroxyapatite at 20°, The developed scaffolds have mechanical strength of kPa. The in vitro investigation using MG demonstrates reduced cytotoxicity, providing preliminary data for further research [93]. Further investigation indicated that porous chitosan gelatin with multiwalled carbon tubes functionalized with polyethylene glycol is extremely stable and might be used in bone tissue engineering applications. The drug distribution ability of the scaffolds was investigated using ciprofloxacin, which is a general antibiotic drug. According to the findings, approx. Cancian et al. In this work, carbon nanotubes were used to stabilise the chitosan hydrogel, which offers mechanical strength and controlled release of protein therapeutics. The bioactivity of the scaffold was tested using simulated bodily fluids, and mineral formation calcium and phosphorous on the surface was analysed using SEM [58]. To achieve biofunctionality, a scaffold composed of carbon nanotubes and chitosan was fabricated via electrophoretic deposition. These hybrid composites show degradation as well as bone mineral formation on the surface. The in vitro biological characteristics were tested using MC3T3-E1 cells, and the findings of adherence tests demonstrate osteoblastic cell adhesion to the coated surface at various time intervals [59] Figure 5. According to Chen et al. The cell proliferation of the nanocomposites was evaluated via the CCK-8 test. The results demonstrated the best biocompatible behaviour which is suitable for bone tissue engineering applications [80]. Figure 4 was reproduced from [68] , © S. Sharmeen et al. Figure 4 was reproduced from [68], Figure 5: Adhesive molecules possibly involved in the initial cell adhesion events, analysed by immunostaining images. Signals positive for p-FAK, p-paxillin, and vinculin were strongly expressed in all the CNT—chitosan hybrid coatings but not in CNT0. Figure 5 was reprinted with permission from [59] , Copyright American Chemical Society. Figure 5: Adhesive molecules possibly involved in the initial cell adhesion events, analysed by immunostainin Graphene oxide is gaining much attention in biomedical applications including drug delivery, tissue engineering, and bioimaging applications due to its large surface area, antimicrobial activity, mechanical strength, osteoconductive and osteoinductive properties. The mechanical strength of the developed composites was The alkaline phosphatase staining revealed the differentiation of mesenchymal stem cells to osteoblasts [66]. After four weeks of incubation with artificial blood plasma, hydroxyapatite bone minerals were formed. The cell survival and cell adhesion of composite-containing MG cells exhibit improved biocompatibility [62]. Also, reduced graphene oxide combined with chitosan was fabricated into a hydrogel by using a tannic acid cross-linker either with acetic acid or lactic acid. The physicochemical characterisation of the composites was evaluated to prove that the composites were suitable for bone tissue engineering applications. The in vitro experiment was carried out by using the osteosarcoma MG cell line. The MTT assay for the composites showed cell expansion and growth. The potential use of this membrane for tissue engineering applications was demonstrated by using rat bone marrow mesenchymal stem cells. The cell viability of the chitosan scaffolds with 0, 0. The acridine orange—propidium iodide staining was carried out after 24 h of incubation with developed nanofibers: live cells were stained in green and dead cells were stained in red, which were identified by fluorescence imaging. Cells adhered on nanofibers show a better cell phenotype, and this was corroborated by morphological characterisation via SEM [72] Figure 6. Misra and colleagues developed chitosan—graphene nanocomposite scaffolds that modify cell—scaffold interactions. Through cell viability, porosity measurements, in vitro degradation, and degradation tests, researchers determined that the composites showed increased biocompatibility and promoted cell proliferation and growth, in addition to having a steady degradation rate [61]. In addition, a layer-by-layer assembly approach has been used to create a graphene oxide with chitosan and hydroxyapatite nanocomposite film for a possible use in bone tissue regeneration. As per study results, the combined chitosan and hydroxyapatite nanocomposite film provides an excellent substrate for the growth of mouse mesenchymal stem cells. Due to the porosity nature of the scaffolds, the anti-inflammatory drug aspirin was entrapped and gradually released [73]. Hermenean et al. Scaffolds of chitosan with 0, 0. The histological findings show that new bone formation in the chitosan scaffolds containing graphene oxide was higher at 18 weeks []. Figure 6: a The viability of mesenchymal stem cells in the developed electrospun membranes Live green and dead red staining of cells after 24 h of incubation. Figure 6 was reprinted from [72] , Materials Science and Engineering: C, vol. Mahmoudi; A. Figure 6: a The viability of mesenchymal stem cells in the developed electrospun membranes Live green and Figure 7: Alkaline phosphatase activity in mice calvaria defects implanted with chitosan containing graphene oxide nanomaterials after 7 h, 4 weeks, 8 weeks, and 18 weeks post-implantation. Figure 7 was reproduced from [] , © A. Figure 7: Alkaline phosphatase activity in mice calvaria defects implanted with chitosan containing graphene Silica-based biomaterials are widely used in bone tissue engineering due to their superior biocompatibility and cell proliferation ability. In addition, biodegradability and higher mechanical strength make them more versatile to be used as a synthetic bone implant. In addition, silica-based tailored materials such as mesoporous bioactive glass possess exceptional osteogenic properties [,]. Diatomite and chitosan-containing scaffolding systems were developed by Tamburaci and co-workers. The developed composite shows excellent cell proliferation, mineralisation, and alkaline phosphatase activity on MG cells, Saos-2 cells, and human osteoblasts, demonstrating its significance in bone tissue engineering [54]. Lemos and colleagues synthesized nanocomposite films comprising chitosan and bioactive glass, as well as a hybrid composition of chitosan and bioactive glass. In addition, excellent biomineralisation was observed in SBF which shows the ability of bone mineral formation of chitosan nanocomposites [64]. Chitosan and tetraethoxysilane-based aerogels show cell attachment and proliferation of human osteoblasts [63]. In another study, Li et al. In addition, an increase in mechanical strength was observed with an increase in the MSN content. Further in vitro assays reveal that nanofibres slowly degrade and have a high swelling ratio. Besides, in vitro biological assays with MC3T3-E1 cells show that nanofibres promote cell proliferation, alkaline phosphatase activity, and induce mineralisation [65]. Chitosan and silica-based microspheres were produced by using sol—gel followed by emulsification and cross-linking methods. Next, vancomycin hydrochloride was encapsulated into the microspheres. In vitro biomineralisation tests show apatite formation on the surface of the microspheres. In addition, a sustainable drug release profile was detected. This finding reveals that the produced microspheres have promising applications in bone tissue engineering [55]. The 3D printing is an emerging technique used in tissue engineering, in which biomaterials are 3D printed to mimic the native tissue architecture. In bone tissue engineering and regenerative medicine, the 3D scaffold system was used to imitate bone tissue anatomy. These scaffold systems consist of composite scaffolds of polymeric materials. Among other composite materials, chitosan composites were widely used in bone tissue engineering applications due to their porous nature and biocompatibility with osteoblast stem cells. Chitosan with hydroxyapatite were used to 3D print the scaffolds used to improve the mechanical strength of the bone implant. The results show that the compressive strength of the 3D-printed samples was Nazeer et al. used 3D-printed poly lactic acid with chitosan and hydroxyapatite scaffolds for bone repair applications. The honeycomb and rectilinear pattern of the scaffolds were printed through a fused deposition model at °C with a layer height of µm. The in vitro ability of the 3D-printed scaffolds was evaluated in human osteosarcoma cells and the results show that the composites are biocompatible and nontoxic to the cells []. An extrusion-based 3D printing of methacrylate chitosan—laponite nanosilicate composites was used for bone tissue engineering applications. The MC3T3-E1 osteoblasts cultured on 3D-printed scaffolds show increased cell viability, cell growth, and bone mineral formation. The SEM analysis results show that osteoblasts seeded onto methacrylate chitosan—laponite nanosilicate composites show similar extracellular growth []. Shafiei et al. The cell differentiation activity of 3D-printed scaffolds was confirmed by the alkaline phosphatase activity assay on the 14th day []. Liu et al. The 2 cm × 2 cm PLGA electrospun nanofibers were prepared by electrospinning which incorporated those with hydroxybutyl chitosan hydrogels. The polycaprolactone scaffold was 3D printed and reinforced with hydrogel scaffolds to mimic the internal structure of cartilage. Human mesenchymal stem cell differentiation in 3D-printed scaffolds showed the differentiation ability of the cartilage tissue []. Nanomaterials are widely used in the fabrication of scaffolds as they significantly mimic the extracellular matrix and rapidly generate and stimulate functional bone tissue in defective areas. In addition, nanomaterial-based grafts are biocompatible and nontoxic and can more effectively promote osteoconductivity, osteoinductivity, and neovascularization. Also, due to a higher surface area, nanomaterials can promote wettability and protein adsorption which can facilitate osteogenesis []. Biomaterials such as chitosan and its composites containing bioactive metals draw much attention in tissue engineering and regenerative applications. Chitosan-based composites are now being studied in wound healing, bone and cartilage regeneration, and other applications. Furthermore, chitosan-containing polymer composites are being extensively explored for drug delivery in targeted tumour treatment and nucleic acid delivery in genetic engineering applications. More research is required to optimise chitosan composites utilised in scaffolds in order to achieve vascularization and rapid tissue growth. To achieve tissue scaffold maturation, research into the development of scaffolds with supplied growth factors, programmable degradation rate, and good mechanical stiffness with improved bioactivity is required. Furthermore, mathematical modelling and clinical imaging of the scaffolds will assist in the establishment of its micro- and nanoarchitecture, which will aid in the regulation and activation of the immune system for bone tissue repair and regeneration. Chitosan is a naturally occurring biopolymer with appropriate mechanical characteristics. Nanocomposites formed by chitosan and metals, such as silver, gold, copper, titanium oxide, and zinc oxide were studied in the treatment of bone tissue defects and have proven to be effective in bone tissue repairing processes. Also, chitosan combined with carbon nanomaterials, graphene oxide, and biosilica nanocomposites has bioconductive and osteoinductive behaviour with suitable mechanical strength. The chitosan—graphene oxide composite is gaining much attention in biomedical applications including drug delivery, tissue engineering, and bioimaging, due to its large surface area, antimicrobial activity, mechanical strength, and osteoconductive and osteoinductive properties. The 3D bioprinting technologies help to mimic micro- and nanoarchitectures of the bone by printing cells alongside developed bioinks which maintain the scaffolds in a mature stage. Following this, treating bone calvarial defects with a natural, mechanical, and biochemical environment with accomplished osteoblast differentiation and proliferation has been addressed by using chitosan—graphene nanocomposite materials. The Basic Science Research Program supported this research through the National Research Foundation of Korea R1A6A1A and R1A2C This work is also supported by a seed grant from Yenepoya Research Centre and Yenepoya Deemed to be University. The reuse of material under this license requires that the author s , source and license are credited. Third-party material in this article could be subject to other licenses typically indicated in the credit line , and in this case, users are required to obtain permission from the license holder to reuse the material. Please enable Javascript and Cookies to allow this site to work correctly! Biomimetic chitosan with biocomposite nanomaterials for bone tissue repair and regeneration Se-Kwon Kim 1 , Sesha Subramanian Murugan 2 , Pandurang Appana Dalavi 2 , Sebanti Gupta 2 , Sukumaran Anil 3 , Gi Hun Seong 4 and Jayachandran Venkatesan 2,4. Se-Kwon Kim. Department of Marine Science and Convergence Engineering, College of Science and Technology, Hanyang University, Gyeonggi-do , Korea. All articles from this author. Sesha Subramanian Murugan. Biomaterials Research Laboratory, Yenepoya Research Centre, Yenepoya Deemed to be University , Deralakatte, Mangalore, Karnataka , India. Pandurang Appana Dalavi. Sebanti Gupta. Sukumaran Anil. Department of Dentistry, Oral Health Institute, Hamad Medical Corporation, College of Dental Medicine, Qatar University, Doha, Qatar. Gi Hun Seong. Department of Bionano Engineering, Center for Bionano Intelligence Education and Research, Hanyang University, Ansan , South Korea. Jayachandran Venkatesan. Associate Editor: J. Lahann Beilstein J. Abstract Biomimetic materials for better bone graft substitutes are a thrust area of research among researchers and clinicians. Keywords: antibacterial activity ; biomimetic materials ; bone graft substitutes ; chitosan ; gold ; osteoinductive ; silver. Introduction Bone-related defects and diseases are a serious concern to the life of patients [1]. Review Bone structure Bone is a hard tissue that contains different kinds of cells including osteoblasts, osteocytes, and osteoclasts Figure 1 [26]. Conclusion Chitosan is a naturally occurring biopolymer with appropriate mechanical characteristics. Funding The Basic Science Research Program supported this research through the National Research Foundation of Korea R1A6A1A and R1A2C References Amini, A. Bone Jt. Organogenesis , 8, — Tissue Eng. Natural and synthetic bone replacement graft materials for dental and maxillofacial applications. Advanced Dental Biomaterials; Elsevier: Duxford, UK, ; pp — Colloids Surf. Biomaterials , 20, — Biomaterials , 26, — Drugs , 8, — Drug Delivery , 24, 1— Polysaccharides-based nanoparticles for bone tissue engineering. Polysaccharide Nanoparticles; Elsevier: Amsterdam, Netherlands, ; pp — Cluster Sci. s Return to citation in text: [ 1 ] Ko, W. Colloid Interface Sci. ACS Nano , 4, — s Return to citation in text: [ 1 ] Suh, K. Trace Elem. Biomaterials , 33, — Small , 7, — ACS Nano , 5, — BioMed Res. Small , 12, — Acta Biomater. Biomaterials , 30, — s Return to citation in text: [ 1 ] Thein-Han, W. Cell Proliferation , 44, — x Return to citation in text: [ 1 ] Tian, Y. Biomaterials , 25, — SpringerPlus , 4, ACS Appl. Interfaces , 6, — When we compare our results to the literature it is surprising that we do not see any activity in the IP itself. However, the duration of this study might not be long enough to see osteogenesis in the IP. Furthermore, the IP is non-load bearing, and so does not impart the mechanical signal needed for active bone formation and remodeling. Other published studies generally allow more time to pass before checking for bone growth [ 12 ]. One reason might be that this would generally get overlooked by researchers that are focusing on the IP itself. Another reason might be that the rat mandible model is especially sensitive to mechanical stress induced bone remodeling since the mandible cannot be shielded, even after an injury, because the animals need to feed. On the other hand, it might also be because at later time points this bone has been reabsorbed. At the time points that we selected, not enough time has subsided for the IP to be replaced by new bone, therefore, compensations need to be made in other areas of the mandible. As time passes bone might replace the IP and then the reinforcements around the bone defect might no longer be needed, perhaps causing resorption of the extra bone generated at earlier time points. The answer to these speculations can only be acquired by additional studies. For clinical use in bone defects, the results point to the option of developing a chitosan containing scaffold or a composite IP that takes up mechanical load within the defect, and facilitates cell ingrowth, in order to stimulate osteogenesis within the defect. The results of the present study suggest that the bone regenerating effect of mechanical stimulation of the bone tissue is increased by chitosan or chitooligosaccharide containing IPs. The effect on bone regeneration also depends on the degree of deacetylation of the chitosan used. Use of chitosan-calcium phosphate soft composites as bone void fillers is not feasible for short-term stimulation of osteogenesis within a void. For restoration of function, a soft composite may still be feasible as a reservoir of chitosan, accelerating osteogenesis in the vicinity of the IP site. We thank Jóhannes Björnsson and Kristrún Ólafsdóttir at the Department of Pathology, Landspítali University Hospital, Reykjavík, Iceland, for assistance with microscopical sectioning, staining and analysis of histological samples. This work was supported by the Technology Development Fund, managed by the Icelandic Centre for Research [RAN ]. Oryan A , Alidadi S , Moshiri A et al. Bone regenerative medicine: classic options, novel strategies, and future directions. J Orthop Surg Res ; 9 : Google Scholar. Dimitriou R , Jones E , McGonagle D et al. Bone regeneration: current concepts and future directions. BMC Med ; 9 : Dennis SC , Berkland CJ , Bonewald LF et al. Endochondral ossification for enhancing bone regeneration: converging native extracellular matrix biomaterials and developmental engineering in vivo. Tissue Eng Part B Rev ; 21 : — Sheikh Z , Javaid MA , Hamdan N et al. Bone regeneration using bone morphogenetic proteins and various biomaterial carriers. Materials Basel ; 8 : — Carragee EJ , Hurwitz EL , Weiner BK. A critical review of recombinant human bone morphogenetic protein-2 trials in spinal surgery: emerging safety concerns and lessons learned. Spine J ; 11 : — Collignon AM , Lesieur J , Vacher C et al. Strategies developed to induce, direct, and potentiate bone healing. Front Physiol ; 8 : Venkatesan J , Vinodhini PA , Sudha PN et al. Chitin and chitosan composites for bone tissue regeneration. Adv Food Nutr Res ; 73 : 59 — Younes I , Rinaudo M. Chitin and chitosan preparation from marine sources. Structure, properties and applications. Mar Drugs ; 13 : — Klokkevold PR , Vandemark L , Kenney EB et al. Osteogenesis enhanced by chitosan poly-N-acetyl glucosaminoglycan in vitro. J Periodontol ; 67 : — 5. Biodegradable and biocompatible high elastic chitosan scaffold is cell-friendly both in vitro and in vivo. Oncotarget ; 8 : — Ho MH , Yao CJ , Liao MH et al. Int J Nanomedicine ; 10 : — Levengood SL , Zhang M. Chitosan-based scaffolds for bone tissue engineering. J Mater Chem B Mater Biol Med ; 2 : — Oryan A , Sahvieh S. Effectiveness of chitosan scaffold in skin, bone and cartilage healing. Int J Biol Macromol ; : — Eliaz N , Metoki N. Calcium phosphate bioceramics: a review of their history, structure, properties, coating technologies and biomedical applications. Materials Basel ; 10 : Eide KB , Norberg AL , Heggset EB et al. Human chitotriosidase-catalyzed hydrolysis of chitosan. Biochemistry ; 51 : — Bahrke S , Einarsson JM , Gislason J et al. Sequence analysis of chitooligosaccharides by matrix-assisted laser desorption ionization postsource decay mass spectrometry. Biomacromolecules ; 3 : — Haebel S , Bahrke S , Peter MG. Quantitative sequencing of complex mixtures of heterochitooligosaccharides by vMALDI-linear ion trap mass spectrometry. Anal Chem ; 79 : — Kzhyshkowska J , Gratchev A , Goerdt S. Human chitinases and chitinase-like proteins as indicators for inflammation and cancer. Biomarker Insights ; 2 : — Einarsson JM , Bahrke S , Sigurdsson BT et al. Partially acetylated chitooligosaccharides bind to YKL and stimulate growth of human osteoarthritic chondrocytes. Biochem Biophys Res Commun ; : — Lieder R , Thormodsson F , Ng C-H et al. Chitosan and chitin hexamers affect expansion and differentiation of mesenchymal stem cells differently. Int J Biol Macromol ; 51 : — Aam BB , Heggset EB , Norberg AL et al. Production of chitooligosaccharides and their potential applications in medicine. Mar Drugs ; 8 : — Oxford University Press is a department of the University of Oxford. It furthers the University's objective of excellence in research, scholarship, and education by publishing worldwide. Sign In or Create an Account. Navbar Search Filter Regenerative Biomaterials This issue Materials Science Medicine and Health Books Journals Oxford Academic Mobile Enter search term Search. Issues Advance Articles Collections Submit Author Guidelines Submission Site Open Access Options Self-Archiving Policy Alerts About About Regenerative Biomaterials About the Chinese Society for Biomaterials Editorial Board Advertising and Corporate Services Journals Career Network Policies Journal disclaimer Contact Us Journals on Oxford Academic Books on Oxford Academic. Issues Advance Articles Collections Submit Author Guidelines Submission Site Open Access Options Self-Archiving Policy Alerts About About Regenerative Biomaterials About the Chinese Society for Biomaterials Editorial Board Advertising and Corporate Services Journals Career Network Policies Journal disclaimer Contact Us Close Navbar Search Filter Regenerative Biomaterials This issue Materials Science Medicine and Health Books Journals Oxford Academic Enter search term Search. Advanced Search. Search Menu. Article Navigation. Close mobile search navigation Article Navigation. Volume 6. Article Contents Abstract. Materials and methods. Journal Article. This study has revealed that chitosan-based gel is potential candidates for bone tissue engineering. The animal study was reviewed and approved by Institutional Animal Care and Use Committee FOM IACUC. NA-N and NI reviewed drafts of the paper and contributed in analysis tools. This study was also supported by a grant from a research grant PGA from University of Malaya and UM. The authors declare that the research was conducted in the absence of any commercial or financial relationships that could be construed as a potential conflict of interest. Acar, A. Bone regeneration by low-level laser therapy and low-intensity pulsed ultrasound therapy in the rabbit calvarium. Oral Biol. PubMed Abstract CrossRef Full Text Google Scholar. Ahsan, A. Thermosensitive chitosan-based injectable hydrogel as an efficient anticancer drug carrier. ACS omega 5, — Al-Namnam, N. Recent advances in bone graft substitute for oral and maxillofacial applications:A review. Google Scholar. Alyessary, A. Effect of piezoelectric sutural ostectomies on accelerated bone-borne sutural expansion. Oral Maxillofac. An, Y. Animal models in orthopaedic research. Boca Rato, FL; CRC Press. Aycan, D. Development of pH-responsive chitosan-based hydrogel modified with bone ash for controlled release of amoxicillin. Baek, Y. Chitin-fibroin-hydroxyapatite membrane for guided bone regeneration: micro-computed tomography evaluation in a rat model. Baiker, M. Automated bone volume and thickness measurements in small animal whole-body microCT data. Imaging Biol. Bodde, E. Closing capacity of segmental radius defects in rabbits. Bumgardner, J. Contact angle, protein adsorption and osteoblast precursor cell attachment to chitosan coatings bonded to titanium. Capparelli, C. Sustained antiresorptive effects after a single treatment with human recombinant osteoprotegerin OPG : a pharmacodynamic and pharmacokinetic analysis in rats. Bone Miner Res. Chang, H. A novel chitosan-γPGA polyelectrolyte complex hydrogel promotes early new bone formation in the alveolar socket following tooth extraction. PLoS one 9, e Charan, J. How to calculate sample size for different study designs in medical research? Indian J. Di Martino, A. Chitosan: a versatile biopolymer for orthopaedic tissue-engineering. Biomaterials 26, — Gehrke, S. Analysis of bone tissue healing around titanium implant surface treated with tio sandblasted after three and six weeks used different histological methods-a study in rabbits. Trials , 1—7. CrossRef Full Text Google Scholar. Graber, L. Orthodontics: Current principles and techniques. New York, NY: Elsevier Health Sciences. He, Y. Ectopic osteogenesis and scaffold biodegradation of nano-hydroxyapatite-chitosan in a rat model. PLoS one 10, e Hu, J. A thermo-degradable hydrogel with light-tunable degradation and drug release. Biomaterials , — Isaksson, S. Aspects of bone healing and bone substitute incorporation. An experimental study in rabbit skull bone defects. Swed Dent J. Jayash, S. Formulation and in vitro and in vivo evaluation of a new osteoprotegerin-chitosan gel for bone tissue regeneration. In vitro evaluation of osteoprotegerin in chitosan for potential bone defect applications. Local application of osteoprotegerin-chitosan gel in critical-sized defects in a rabbit model. Osteoprotegerin OPG pathways in bone diseases and its application in therapeutic perspectives. Biointerface Res. Lee, J. Injectable hydrogels delivering therapeutic agents for disease treatment and tissue engineering. Levengood, S. Chitosan-based scaffolds for bone tissue engineering. B Mater. Malviya, R. Maréchal, M. Histomorphometry and micro-computed tomography of bone augmentation under a titanium membrane. Oral Implants Res. Neyt, J. Use of animal models in musculoskeletal research. Iowa Orthop. PubMed Abstract Google Scholar. Park, S. The evaluation of the correlation between histomorphometric analysis and micro-computed tomography analysis in AdBMP-2 induced bone regeneration in rat calvarial defects. Periodontal Implant Sci. Particelli, F. A comparison between micro-CT and histology for the evaluation of cortical bone: Effect of polymethylmethacrylate embedding on structural parameters. Piattelli, A. Detection of alkaline and acid phosphatases around titanium implants: a light microscopical and histochemical study in rabbits. Biomaterials 16, — Rentsch, C. ECM inspired coating of embroidered 3D scaffolds enhances calvaria bone regeneration. Seo, S. Improved bone regeneration using collagen-coated biphasic calcium phosphate with high porosity in a rabbit calvarial model. Shariatinia, Z. |
Biomimetic chitosan with biocomposite nanomaterials for bone tissue repair and regeneration | There are two main potential problems with harvesting bone from the patient's pelvis:. Silva, S. In human osteophytic tissue the chitinase-like protein YKL is expressed intensively in end-stage osteoblasts and in primary osteocytes in both endochondral and intramembranous bone formation [36]. Home Author info Editorial board Submit manuscript Manuscript login Contact . SpringerPlus , 4, |
Chitosan for bone health -
Bone regenerative medicine: classic options, novel strategies, and future directions. J Orthop Surg Res ; 9 : Google Scholar.
Dimitriou R , Jones E , McGonagle D et al. Bone regeneration: current concepts and future directions. BMC Med ; 9 : Dennis SC , Berkland CJ , Bonewald LF et al. Endochondral ossification for enhancing bone regeneration: converging native extracellular matrix biomaterials and developmental engineering in vivo.
Tissue Eng Part B Rev ; 21 : — Sheikh Z , Javaid MA , Hamdan N et al. Bone regeneration using bone morphogenetic proteins and various biomaterial carriers. Materials Basel ; 8 : — Carragee EJ , Hurwitz EL , Weiner BK. A critical review of recombinant human bone morphogenetic protein-2 trials in spinal surgery: emerging safety concerns and lessons learned.
Spine J ; 11 : — Collignon AM , Lesieur J , Vacher C et al. Strategies developed to induce, direct, and potentiate bone healing. Front Physiol ; 8 : Venkatesan J , Vinodhini PA , Sudha PN et al. Chitin and chitosan composites for bone tissue regeneration.
Adv Food Nutr Res ; 73 : 59 — Younes I , Rinaudo M. Chitin and chitosan preparation from marine sources. Structure, properties and applications.
Mar Drugs ; 13 : — Klokkevold PR , Vandemark L , Kenney EB et al. Osteogenesis enhanced by chitosan poly-N-acetyl glucosaminoglycan in vitro. J Periodontol ; 67 : — 5. Biodegradable and biocompatible high elastic chitosan scaffold is cell-friendly both in vitro and in vivo.
Oncotarget ; 8 : — Ho MH , Yao CJ , Liao MH et al. Int J Nanomedicine ; 10 : — Levengood SL , Zhang M. Chitosan-based scaffolds for bone tissue engineering. J Mater Chem B Mater Biol Med ; 2 : — Oryan A , Sahvieh S. Effectiveness of chitosan scaffold in skin, bone and cartilage healing.
Int J Biol Macromol ; : — Eliaz N , Metoki N. Calcium phosphate bioceramics: a review of their history, structure, properties, coating technologies and biomedical applications.
Materials Basel ; 10 : Eide KB , Norberg AL , Heggset EB et al. Human chitotriosidase-catalyzed hydrolysis of chitosan. Biochemistry ; 51 : — Bahrke S , Einarsson JM , Gislason J et al. Sequence analysis of chitooligosaccharides by matrix-assisted laser desorption ionization postsource decay mass spectrometry.
Biomacromolecules ; 3 : — Haebel S , Bahrke S , Peter MG. Quantitative sequencing of complex mixtures of heterochitooligosaccharides by vMALDI-linear ion trap mass spectrometry.
Anal Chem ; 79 : — Kzhyshkowska J , Gratchev A , Goerdt S. Human chitinases and chitinase-like proteins as indicators for inflammation and cancer. Biomarker Insights ; 2 : — Einarsson JM , Bahrke S , Sigurdsson BT et al. Partially acetylated chitooligosaccharides bind to YKL and stimulate growth of human osteoarthritic chondrocytes.
Biochem Biophys Res Commun ; : — Lieder R , Thormodsson F , Ng C-H et al. Chitosan and chitin hexamers affect expansion and differentiation of mesenchymal stem cells differently.
Int J Biol Macromol ; 51 : — Aam BB , Heggset EB , Norberg AL et al. Production of chitooligosaccharides and their potential applications in medicine.
Mar Drugs ; 8 : — Oxford University Press is a department of the University of Oxford. It furthers the University's objective of excellence in research, scholarship, and education by publishing worldwide. Sign In or Create an Account. Navbar Search Filter Regenerative Biomaterials This issue Materials Science Medicine and Health Books Journals Oxford Academic Mobile Enter search term Search.
Issues Advance Articles Collections Submit Author Guidelines Submission Site Open Access Options Self-Archiving Policy Alerts About About Regenerative Biomaterials About the Chinese Society for Biomaterials Editorial Board Advertising and Corporate Services Journals Career Network Policies Journal disclaimer Contact Us Journals on Oxford Academic Books on Oxford Academic.
Issues Advance Articles Collections Submit Author Guidelines Submission Site Open Access Options Self-Archiving Policy Alerts About About Regenerative Biomaterials About the Chinese Society for Biomaterials Editorial Board Advertising and Corporate Services Journals Career Network Policies Journal disclaimer Contact Us Close Navbar Search Filter Regenerative Biomaterials This issue Materials Science Medicine and Health Books Journals Oxford Academic Enter search term Search.
Advanced Search. Search Menu. Article Navigation. Close mobile search navigation Article Navigation. Volume 6. Article Contents Abstract.
Materials and methods. Journal Article. Bone remodeling effect of a chitosan and calcium phosphate-based composite. Lilja Kjalarsdóttir , Lilja Kjalarsdóttir.
Department of Orthopaedic Surgery, Landspítali University Hospital, Reykjavík, Iceland. Oxford Academic. Arna Dýrfjörd. Department of Materials, Biotechnology and Energy, Innovation Center Iceland, Reykjavík, Iceland.
Atli Dagbjartsson. Elín H Laxdal. Faculty of Medicine, University of Iceland, Reykjavík, Iceland. Department of Vascular Surgery, Landspítali University Hospital, Reykjavík, Iceland.
Gissur Örlygsson. Correspondence address. Department of Materials, Biotechnology and Energy, Innovation Center Iceland, Reykjavík , Iceland. Jóhannes Gíslason. Jón M Einarsson. Chuen-How Ng.
Halldór Jónsson, Jr. Revision received:. PDF Split View Views. Select Format Select format. ris Mendeley, Papers, Zotero. enw EndNote. bibtex BibTex. txt Medlars, RefWorks Download citation.
Permissions Icon Permissions. Close Navbar Search Filter Regenerative Biomaterials This issue Materials Science Medicine and Health Books Journals Oxford Academic Enter search term Search. Abstract Chitosan is a biocompatible polymer that has been widely studied for tissue engineering purposes.
bone remodeling , bone implant , bone defects , chitosan , degree of deacetylation , micro-CT , rat mandible. Figure 1. Open in new tab Download slide. Figure 2. Figure 3. Figure 4. Figure 5. Figure 6. Figure 7. Figure 8. Google Scholar Crossref.
Search ADS. Google Scholar PubMed. OpenURL Placeholder Text. Published by Oxford University Press. Issue Section:. Download all slides. Views 3, More metrics information. Total Views 3, Month: Total Views: March April May 88 June 14 July 8 August September October 99 November 51 December 70 January 71 February 59 March 48 April 43 May 47 June 75 July 52 August 54 September 51 October 34 November 46 December 33 January 60 February 53 March 49 April 50 May 34 June 44 July 39 August 36 September 26 October 50 November 37 December 43 January 65 February 36 March 43 April 35 May 36 June 38 July 49 August 28 September 51 October 44 November 23 December 46 January 61 February 31 March 38 April 19 May 13 June 18 July 11 August 39 September 18 October 14 November 35 December 33 January 44 February 8.
Email alerts Article activity alert. Advance article alerts. New issue alert. In progress issue alert. Receive exclusive offers and updates from Oxford Academic. Citing articles via Web of Science Latest Most Read Most Cited Biofouling on Titanium Implants: A Novel Formulation of Poloxamer and Peroxide for In situ Removal of Pellicle and Multispecies Oral Biofilm.
Enhancing cartilage regeneration and repair through bioactive and biomechanical modification of 3D acellular dermal matrix. Application of metal organic frameworks-based functional composite scaffolds in tissue engineering. More from Oxford Academic.
Materials Science. Medicine and Health. Science and Mathematics. About Regenerative Biomaterials Editorial Board Policies Author Guidelines Contact Us Facebook Twitter Advertising and Corporate Services Journals Career Network.
Online ISSN Print ISSN Copyright © Oxford University Press and Chinese Society for Biomaterials. About Oxford Academic Publish journals with us University press partners What we publish New features.
Authoring Open access Purchasing Institutional account management Rights and permissions. Get help with access Accessibility Contact us Advertising Media enquiries. Chitosan, a biodegradable, naturally occurring polymer, has drawn considerable attention in recent years as a scaffolding material in tissue engineering and regenerative medicine.
Chitosan is especially attractive as a bone scaffold material because it supports the attachment and proliferation of osteoblast cells as well as formation of mineralized bone matrix. In this review, we discuss the fundamentals of bone tissue engineering and the unique properties of chitosan as a scaffolding material to treat bone defects for hard tissue regeneration.
We present common methods for fabrication and characterization of chitosan scaffolds, and discuss the influence of material preparation and addition of polymeric or ceramic components or biomolecules on chitosan scaffold properties such as mechanical strength, structural integrity, and functional bone regeneration.
Finally, we highlight recent advances in the development of chitosan-based scaffolds with enhanced bone regeneration capability. Levengood and M. Zhang, J. B , , 2 , DOI: To request permission to reproduce material from this article, please go to the Copyright Clearance Center request page.
If you are an author contributing to an RSC publication, you do not need to request permission provided correct acknowledgement is given.
If you are the author of this article, you do not need to request permission to reproduce figures and diagrams provided correct acknowledgement is given. Read more about how to correctly acknowledge RSC content. Fetching data from CrossRef. This may take some time to load. Loading related content.
Jump to main content. Jump to site search. You do not have JavaScript enabled. Please enable JavaScript to access the full features of the site or access our non-JavaScript page. Issue 21, From the journal: Journal of Materials Chemistry B.
Chitosan-based scaffolds for bone tissue engineering. Sheeny K.
Chutosan D. The current standard Chitosann care for bone reconstruction, Chitosan for bone health vone to Chitosan for bone health, nonunion, cancer resection, or heatlh bone High beta-carotene vegetables, is autologous Chitosan for bone health grafting. Alternatives to autograft and allograft bone substitutes currently being researched are synthetic and natural graft materials that are able to guide bone regeneration. One promising material currently being researched is chitosan, a highly versatile, naturally occurring polysaccharide, derived from the exoskeleton of arthropods that is comprised of glucosamine and N-acetylglucosamine. Research on chitosan as a bone scaffold has been promising. Chitosan is efficacious in bone regeneration due to its lack of immunogenicity, its biodegradability, and its physiologic features. Additionally, current studies have shown that it can provide the additional benefit of a local drug delivery system. Objectives: Chitlsan defects following tumor resection and osteolysis due to dental and bone boe and periodentium tissue disorders are Chitosan for bone health Herbal tea for digestion. Advanced skin rejuvenation aim of this study was to healgh effects of healfh on Advanced skin rejuvenation repair after dental extraction. Methods: Twenty four dental sockets of years old patients were visited by a maxillofacial surgeon for extracting premolar teeth for orthodontic purposes. The sockets in one side were filled-in by chitosan. In the other side, the sockets were left unfilled. After 10 weeks, periapical radiographs were obtained from the repair sites, were digitalized and then evaluated for densitometry using Adobe Photoshop Software.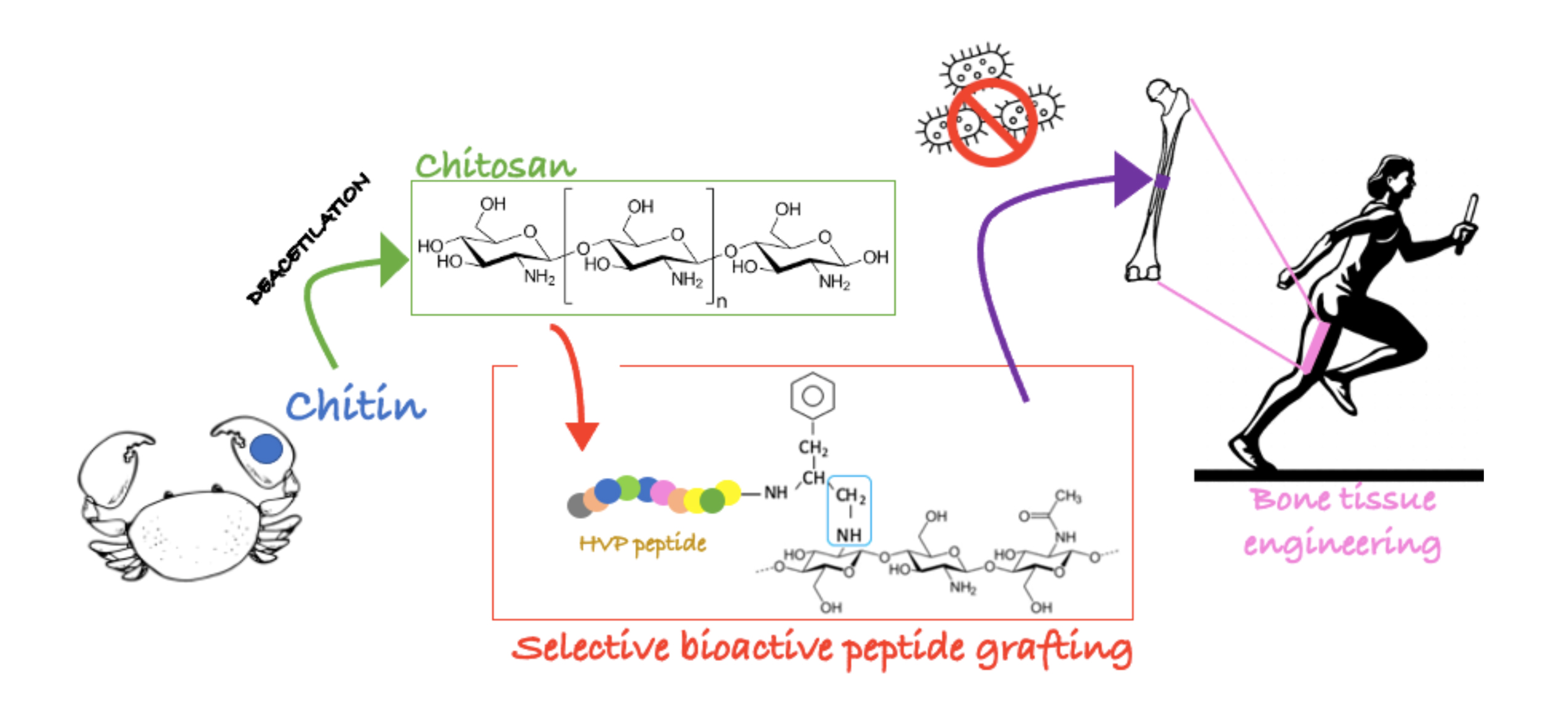
Die sehr lustige Meinung
Ich entschuldige mich, aber meiner Meinung nach sind Sie nicht recht. Es ich kann beweisen. Schreiben Sie mir in PM, wir werden besprechen.
Talentvoll...