Video
Look What Happened to This Lion after Getting Bitten by a Black Mamba Antivenom is Antidoyes Antidotes for snake venom that is given to Prediabetes management snake venom Anttidotes binding to tissues and ffor serious blood, tissue, or nervous Antdiotes problems. Side effects from antivenom can include rash, itching, wheezing, rapid heart rate, fever, and body aches. The use of antivenom depends on how much poison was injected envenomation and the type and size of the snake. Large snakes tend to inject more venom than smaller snakes do. Antivenom is used for mild, moderate, and severe envenomations.The gap between suffering a venomous snakebite Extraordinary obtaining appropriate treatment vneom be long, agonizing, and, ultimately, deadly.
Most Anntidotes occur in rural areas, far from hospitals that can administer necessary Proper nutrition for weight class sports. Time Natural weight loss results treatment Snke determine outcomes.
The area bitten fenom develop Fo, eventually requiring Digestion-friendly lifestyle or amputation.
Antldotes often fot when people accidentally disturb snakes hiding in the environment, along paths, or Antidotes for snake venom fields. The Energy-saving appliances morbidity and mortality vebom Natural weight loss results snakebite occurs snaks rural areas among children, adolescents, and young adults — the farmers, herders, fishers, and others working to support their families.
In many countries, including India, a loss of earning power can be ruinous, especially as the cost of antivenom treatment can exceed twice the average annual income. For people with already limited access to healthcare and education, the setbacks can be felt for generations. Around the world, many populations live in close proximity to venomous snake species.
The looming threat of snakebite becomes reality for an estimated 5. Up to half of those bites are envenomings, resulting in a toll of more thanpeople killed or maimed by snakes each year. Ophirex, a public benefit corporation focused on global health, is working to improve outcomes for victims of snakebite by developing an affordable, accessible, oral treatment for immediate use anywhere a bite occurs.
Most snakebite fatalities occur before victims can reach medical care.
: Antidotes for snake venomSnake Antivenom | This could save lives, especially for victims in remote regions. Serum sickness is a delayed reaction to receiving antivenom and can occur several days or weeks after treatment. We hope that this Review will inspire a new generation of scientists to explore and realize the immense potential of snake venoms. Quignot, C. Gazerani, P. |
New antidote on-trial at Duke could be the first to work for most venomous snakes | The populations hardest hit by the shortages tend to live and work in rural areas where highly venomous snakes are endemic, especially in less-developed nations with housing that allows for easier access by venomous snakes. Toxins in thrombosis and haemostasis: potential beyond imagination. Recombinant ANP and BNP were approved for use in Japan and the USA , respectively, as a therapy for acute decompensated heart failure. Inagaki, H. Badari, J. worthingtoni , B. Crotamine is a novel cell-penetrating protein from the venom of rattlesnake Crotalus durissus terrificus. |
What to do if you're bitten by a rattlesnake | The highest morbidity and mortality due to snakebite occurs in rural areas among children, adolescents, and young adults — the farmers, herders, fishers, and others working to support their families. In many countries, including India, a loss of earning power can be ruinous, especially as the cost of antivenom treatment can exceed twice the average annual income. For people with already limited access to healthcare and education, the setbacks can be felt for generations. Around the world, many populations live in close proximity to venomous snake species. The looming threat of snakebite becomes reality for an estimated 5. Up to half of those bites are envenomings, resulting in a toll of more than , people killed or maimed by snakes each year. So what to do in these situations? Once at the hospital, you will be assessed for possible antivenom treatment. This outcome is based on the severity of your outward symptoms and the results of one or more blood tests. The concern is that envenomation i. Snakebite symptoms can take quite a while to manifest, so you will likely be observed and retested for a minimum of four to six hours. Severe bites may require several days of hospitalization to ensure you have received sufficient antivenom treatment. Bitten by a nonvenomous snake? Just treat it in the field like any puncture wound: clean the bite area and apply a loose bandage. Southern California is home to a handful of rattlesnake species, including the Southern Pacific, red diamond and southwestern speckled. These natives are part of the pit viper family, and the same antivenom works for all of them. Do not attempt to kill or trap the snake for identification purposes — there is no need for this. Suchard and his colleagues still recall the time a well-intentioned bite victim brought a live rattlesnake inside a plastic container into the UC Irvine Medical Center emergency room. This means staying alert to your surroundings and keeping a safe distance from a rattlesnake should you spot one. Further, never taunt a rattlesnake. According to Suchard, the telltale sign of a snake provoker is someone who receives a bite in the face or arms. View previous campaigns. Medical Services Find a Doctor. |
What NOT to do | A homology model of babourin template PDB ID: 1J2L is shown on the left. Today, it is possible to assemble a database of biological targets for which the molecular structures have been determined by X-ray or cryogenic electron microscopy and homology modelling and then to screen the database according to toxin—target affinity. The mambalgins are promising scaffolds for the development of a new generation of analgesics. Vu, T. The Rosetta all-atom energy function for macromolecular modeling and design. Six disulfide bonds stabilize the structures. The use of antivenom depends on how much poison was injected envenomation and the type and size of the snake. |
Venomous Snake Bites: Symptoms & First Aid | Section Navigation. Facebook Twitter LinkedIn Syndicate. Minus Related Pages. Photos courtesy of Sean P. First Aid Workers should take these steps if a snake bites them: Seek medical attention as soon as possible dial or call local Emergency Medical Services [EMS]. Antivenom is the treatment for serious snake envenomation. The sooner antivenom can be started, the sooner irreversible damage from venom can be stopped. Driving oneself to the hospital is not advised because people with snakebites can become dizzy or pass out. Take a photograph of the snake from a safe distance if possible. Identifying the snake can help with treatment of the snakebite. Keep calm. Inform your supervisor. Apply first aid while waiting for EMS staff to get you to the hospital. Topic Contents Overview Related Information Credits. Top of the page. Overview Antivenom is a medicine that is given to stop snake venom from binding to tissues and causing serious blood, tissue, or nervous system problems. Dry bites no venom injected do not need to be treated with antivenom. Mild envenomation bites may cause mild symptoms, such as slight bleeding, pain, and swelling at the bite. Moderate envenomations are more likely to cause symptoms of severe pain, swelling of the whole limb, and general feelings of illness, such as nausea, vomiting, and weakness. Severe envenomation symptoms include severe pain, severe swelling, difficulty breathing, moderate to severe bleeding, and signs of shock. Snake venoms can cause many problems, such as: Blood-clotting problems. Injury to muscles. Low blood pressure leading to shock. Kidney damage. Nervous system problems. Severe allergic reactions. Related Information Snake and Lizard Bites. Credits Current as of: March 1, Current as of: March 1, Pharmaceutical companies invest vast sums of capital in maintaining and expanding massive, private compound libraries. These infrastructures aim to fulfil a basic need of modern drug discovery: chemical diversity. In other words, if the goal is to conduct high-throughput ligand screening for a target of interest, it will not be easy to justify that the use of animal venoms is advantageous over the more extensive and more diverse traditional chemical libraries. The advantage of animal venom toxins is their high, specific and inherent bioactivity, which enables a drug-candidate toxin to be chosen on the basis of a previously observed bioactivity and not through high-throughput screening. The selection process has to be rational, not automated. It is easy to glimpse the wealth of diseases we can eventually treat by looking at the summarized list of bioactivities reported in Table 1. Nevertheless, this approach implies the need for flexibility in the pharmaceutical industry, which is currently focusing on automation There are two paths for developing drugs from snake venoms: the use of toxins without modifications or the design of small synthetic compounds that mimic the recognition motifs of toxins, which are called toxinomimetics. The use of unmodified snake toxins has not been very successful. Neither the FDA nor the EMA has approved batroxobin, haemocoagulase or cobrotoxin. However, the FDA and the EMA have approved unmodified peptide toxins from the venom of other animals 4 , such as bivalirudin a toxin from the medicinal leech used to prevent coagulation during surgery , ziconotide a toxin from the magic cone snail used to treat chronic pain 96 and exenatide a toxin from the Gila monster used to treat type 2 diabetes The use of unmodified toxins as prescription drugs comes at a cost: it hinders their administration, stability and large-scale production. To circumvent these issues, toxinomimecry, a technique that involves complex, rational transformations of the toxin core, has been successful for deriving drugs from snake venoms, as shown by the development of captopril and its analogues, as well as the development of tirofiban and eptifibatide. This path is not always preferable to the use of unmodified toxins. The preferable path is simply the one that provides more efficient, safer and cheaper drugs. As most toxins are peptides or proteins, their administration is usually problematic. Despite considerable resistance to proteolysis, owing to the numerous disulfide bridges within most toxins 34 , , oral administration is generally inefficient, in part owing to difficulties in crossing cell membranes. This issue is evident in the lack of oral bioavailability of most snake-venom-based drugs Intravenous administration is generally the least invasive administration route that works, constraining the appeal of toxins for drug discovery. The cost and complexity of their large-scale synthesis, extraction and purification or heterologous expression is a further constraint. Toxinomimicry is a possible way to overcome these problems by using the toxin as a reporter rather than a drug. In this strategy, the role of the toxin is to reveal the molecular determinants of activity and specificity for the target, which are then mimicked as extensively as possible with a small synthetic molecule that is affordable to synthesize and orally bioavailable. The realization of the full power of toxinomimicry requires the resolution of the structures of toxin—target complexes of interest. Identification of the targets of haemotoxins in the well known coagulation cascade has been quite successful ; conversely, identification of the targets of neurotoxins in the less understood and more complex human neurological system has proved more challenging However, the functions of many of these channels are poorly understood, if not uncharacterized. Their molecular structures are often unknown and challenging to determine. The many isoforms of ion channels further complicate their selective targeting If a toxin binds an unknown target, identification of the target is challenging owing to the technical difficulty of screening target pools. The binding of PLA2s to such a membrane protein narrows its biodistribution, focusing its hydrolytic action on muscle tissues , However, even after many years of study, the identity of the myotoxic PLA2 pharmacologic target is still unknown. When target identification is successful, determining the target structure and the target—toxin complex is still challenging. Knowing the 3D structure of the complex is a requisite to the understanding of molecular recognition, without which the design of toxinomimetics within a structure-based paradigm is not possible. In this case, toxinomimicry has to resort to a ligand-based paradigm, supported by measurements of ligand affinity for toxin mutants, which is less efficient than structure-based drug design because it is rooted in less molecular information. The path forward should entail, at least in part, a much deeper involvement of computational chemistry. The increase in computational power allows for the more exact implementation of physical principles, which, together with the greater involvement of deep learning and artificial intelligence, is powering advances in computational fields important for snake-venom-based chemistry and drug discovery, such as protein homology modelling , , , , , ; protein—protein docking , , , , , , , ; computational mutagenesis, in particular alanine scanning , , , , , ; and the determination of enzymatic mechanisms 88 , 89 , 90 , Computational chemistry can thus have a decisive role in speeding up the process of drug discovery based on snake venom toxins. Computational chemistry can intervene whenever a toxin with the bioactivity of interest acts on an unknown target. Today, it is possible to assemble a database of biological targets for which the molecular structures have been determined by X-ray or cryogenic electron microscopy and homology modelling and then to screen the database according to toxin—target affinity. In several cases, the uncertainties associated with homology modelling and docking do not allow for the identification of a single and robust target. Nevertheless, computational chemistry reduces the target pool to a set small enough to be feasible for experimental testing. It is also challenging to determine target—toxin complex geometries with atomic-level accuracy through computation alone , particularly when modelled structures are involved. Despite this, computational chemistry can narrow down the target and toxin regions that contact each other to the point at which experimental mutagenesis and other techniques can be applied to provide the final atomic-level information. As an example, computational and experimental methodologies were used together to clarify the mechanism by which mambalgins inhibit ASICs , In summary, high-level computational chemistry has the power to advance target identification and target—toxin structural determination if conducted in synergy with experiments; together they could facilitate either the use of unmodified toxins or the modelling of toxin-based small ligands. For the latter, traditional medicinal chemistry can be employed to reduce the toxins into small, synthetic, bioavailable molecules while keeping most of the determinants for recognition and affinity. In modern Western civilization, the snake represents deceit and triggers both fascination and fear. However, ancient civilizations respected the snake owing to the healing power of its venom. It is becoming evident that the ancients were right, as the venom of this splendid animal is an extraordinary library of bioactive compounds that has great medicinal potential. Efforts to elucidate the chemical reactivity of the principal toxins within venom is helping to increase understanding of how toxins act on their prey targets, and how one can engineer toxin action to achieve a therapeutic goal. Furthermore, understanding of venom chemistry allows for the rational design of transition-state small-molecule analogue inhibitors for primary enzymatic toxins that are today the most promising candidates for replacing the difficult-to-manage and expensive antibody-based treatments for snakebite envenoming. The molecular recognition features of snake venom toxins are also being explored at a molecular level. The drugs already approved and under development derived from snake venom demonstrate that toxic bioactivity can be transformed into a therapy for the right disease. Large toxin molecules can be redesigned and reduced to their recognition motifs for oral delivery while maintaining affinity and specificity. Of the many drugs in preclinical development, mambalgins in particular reflect the contrast between their therapeutic promise in this case, to relieve pain and their origin from one of the most feared snakes on the planet. In terms of the future of venom-based drug development, we assert that toxinomimicry is an exciting alternative and a complement to the use of unmodified toxins. Furthermore, computational chemistry, which is still underused in the field, can accelerate the understanding of snake venom chemistry and hence the development of new drugs. We hope that this Review will inspire a new generation of scientists to explore and realize the immense potential of snake venoms. Holford, M. Venoms to the rescue. Science , — Article CAS PubMed Google Scholar. Casewell, N. Complex cocktails: the evolutionary novelty of venoms. Trends Ecol. A review of the natural history of venoms and mechanisms of venom evolution. Article PubMed Google Scholar. King, G. Venoms as a platform for human drugs: translating toxins into therapeutics. Expert Opin. This review covers all stages of the development of drugs based on animal venoms. Herzig, V. et al. Article CAS PubMed PubMed Central Google Scholar. Pineda, S. Structural venomics reveals evolution of a complex venom by duplication and diversification of an ancient peptide-encoding gene. Natl Acad. USA , — Cid-Uribe, J. Scorpion venomics: a overview. Expert Rev. Article CAS Google Scholar. Tasoulis, T. A review and database of snake venom proteomes. Toxins 9 , An analysis of the composition and diversity of snake venom. Article PubMed Central Google Scholar. Medically important differences in snake venom composition are dictated by distinct postgenomic mechanisms. Massey, D. Venom variability and envenoming severity outcomes of the Crotalus scutulatus scutulatus Mojave rattlesnake from southern Arizona. Causes and consequences of snake venom variation. Trends Pharmacol. Durban, J. Integrated venomics and venom gland transcriptome analysis of juvenile and adult Mexican rattlesnakes Crotalus simus, C. tzabcan , and C. culminatus revealed miRNA-modulated ontogenetic shifts. Proteome Res. Pla, D. Phylovenomics of Daboia russelii across the Indian subcontinent. Bioactivities and comparative in vivo neutralization and in vitro third-generation antivenomics of antivenoms against venoms from India, Bangladesh and Sri Lanka. Senji Laxme, R. PLoS Negl. Chanda, A. Proteomic analysis and antivenomics study of Western India Naja naja venom: correlation between venom composition and clinical manifestations of cobra bite in this region. Article Google Scholar. Investigating toxin diversity and abundance in snake venom proteomes. A review of the proteomic methods used to separate and quantify snake venom toxins, comparing their merits and limitations. Snake-bite envenoming: a priority neglected tropical disease. Lancet , 2 Gutierrez, J. Snakebite envenoming. Primers 3 , A review on the pathophysiology and treatment of snakebite envenoming. Williams, D. The Global Snake Bite Initiative: an antidote for snake bite. Lancet , 89—91 Kasturiratne, A. The global burden of snakebite: a literature analysis and modelling based on regional estimates of envenoming and deaths. PLoS Med. McDermott, A. Venom back in vogue as a wellspring for drug candidates. Bordon, K. From animal poisons and venoms to medicines: achievements, challenges and perspectives in drug discovery. Article PubMed PubMed Central Google Scholar. Almeida, J. Snake venom peptides and low mass proteins: molecular tools and therapeutic agents. Fry, B. Genome Res. Ojeda, P. Computational studies of snake venom toxins. Toxins 10 , 8 Calvete, J. Venoms, venomics, antivenomics. FEBS Lett. Modahl, C. Omics technologies for profiling toxin diversity and evolution in snake venom: impacts on the discovery of therapeutic and diagnostic agents. The Uniprot Consortium. UniProt: the universal protein knowledgebase in Nucleic Acids Res. Simoes-Silva, R. Snake venom, a natural library of new potential therapeutic molecules: challenges and current perspectives. Next-generation snake venomics: protein-locus resolution through venom proteome decomplexation. Brahma, R. Venom gland transcriptomics for identifying, cataloging, and characterizing venom proteins in snakes. Toxicon 93 , 1—10 Liu, L. Comparison of next-generation sequencing systems. Gutiérrez, J. Phospholipases A 2 : unveiling the secrets of a functionally versatile group of snake venom toxins. Toxicon 62 , 27—39 A review of the structure and function of the central PLA2 toxin family. Dennis, E. Phospholipase A2 enzymes: physical structure, biological function, disease implication, chemical inhibition, and therapeutic intervention. Kang, T. Enzymatic toxins from snake venom: structural characterization and mechanism of catalysis. FEBS J. A paper focusing on the structure and reaction mechanisms of the most prominent snake venom enzymatic toxins. Schaloske, R. The phospholipase A2 superfamily and its group numbering system. Acta , —59 Ferraz, C. Multifunctional toxins in snake venoms and therapeutic implications: from pain to hemorrhage and necrosis. Kini, R. Snake venom three-finger toxins and their potential in drug development targeting cardiovascular diseases. A review of the family of 3FTxs and their potential medicinal applications for cardiovascular diseases. Molecular evolution and phylogeny of elapid snake venom three-finger toxins. Structure, function and evolution of three-finger toxins: mini proteins with multiple targets. Toxicon 56 , — Olaoba, O. Snake venom metalloproteinases SVMPs : a structure—function update. Toxicon X 7 , A review of the large and complex family of SVMP toxins. Hemorrhage caused by snake venom metalloproteinases: a journey of discovery and understanding. Toxins 8 , 93 Takeda, S. ADAM and ADAMTS family proteins and snake venom metalloproteinases: a structural overview. Toxins 8 , Ullah, A. Thrombin-like enzymes from snake venom: structural characterization and mechanism of action. Hiu, J. Cytotoxicity of snake venom enzymatic toxins: phospholipase A 2 and l -amino acid oxidase. Tan, K. l -amino acid oxidase from snake venom and its anticancer potential. Toxicon , 7—13 Paloschi, M. An update on potential molecular mechanisms underlying the actions of snake venom l -amino acid oxidases LAAOs. Structure—function studies and mechanism of action of snake venom l -amino acid oxidases. Inagaki, H. in Snake Venoms eds Gopalakrishnakone, P. Springer, Markland, F. Snake venom metalloproteinases. Toxicon 62 , 3—18 Serrano, S. Snake venom serine proteinases: sequence homology vs. substrate specificity, a paradox to be solved. Toxicon 45 , — The long road of research on snake venom serine proteinases. Toxicon 62 , 19—26 Arlinghaus, F. C-type lectin-like proteins from snake venoms. Toxicon 60 , — Morita, T. Structures and functions of snake venom CLPs C-type lectin-like proteins with anticoagulant-, procoagulant-, and platelet-modulating activities. Lu, Q. Snake venom C-type lectins interacting with platelet receptors. Structure—function relationships and effects on haemostasis. Vink, S. Natriuretic peptide drug leads from snake venom. Toxicon 59 , — Sridharan, S. Venom natriuretic peptides guide the design of heart failure therapeutics. This review examines the structure—function relationships of venom natriuretic peptides, and discusses peptide engineering strategies for creating therapeutic natriuretic peptide analogues. Munawar, A. Snake venom peptides: tools of biodiscovery. Toxins 10 , Article CAS PubMed Central Google Scholar. Laustsen, A. Unveiling the nature of black mamba Dendroaspis polylepis venom through venomics and antivenom immunoprofiling: identification of key toxin targets for antivenom development. Damm, M. Comprehensive snake venomics of the Okinawa Habu pit viper, Protobothrops flavoviridis , by complementary mass spectrometry-guided approaches. Molecules 23 , Coronado, M. Structure of the polypeptide crotamine from the Brazilian rattlesnake Crotalus durissus terrificus. Acta Crystallogr. D 69 , — Falcao, C. Crotamine and crotalicidin, membrane active peptides from Crotalus durissus terrificus rattlesnake venom, and their structurally-minimized fragments for applications in medicine and biotechnology. Peptides , Slotta, K. Two active proteins from rattlesnake venom. Nature 13 , — Berg, O. Interfacial enzymology: the secreted phospholipase A 2 -paradigm. Tsai, Y. Desolvation map of the i-face of phospholipase A2. Acta , — Bahnson, B. Structure, function and interfacial allosterism in phospholipase A2: insight from the anion-assisted dimer. Scott, D. Interfacial catalysis: the mechanism of phospholipase A2. Sérgio, S. ACS Catal. Sousa, S. Activation free energy, substrate binding free energy, and enzyme efficiency fall in a very narrow range of values for most enzymes. Resende, L. Structural, enzymatic and pharmacological profiles of AplTX-II — a basic sPLA2 D49 isolated from the Agkistrodon piscivorus leucostoma snake venom. Lomonte, B. Snake venom Lys49 myotoxins: from phospholipases A 2 to non-enzymatic membrane disruptors. Toxicon 60 , —30 Fernández, J. Muscle phospholipid hydrolysis by Bothrops asper Asp49 and Lys49 phospholipase A2 myotoxins — distinct mechanisms of action. CoaTx-II, a new dimeric Lys49 phospholipase A2 from Crotalus oreganus abyssus snake venom with bactericidal potential: insights into its structure and biological roles. Toxicon , —58 Harnessing snake venom phospholipases A 2 to novel approaches for overcoming antibiotic resistance. Drug Dev. A novel synthetic peptide inspired on Lys49 phospholipase A2 from Crotalus oreganus abyssus snake venom active against multidrug-resistant clinical isolates. Kwong, P. Structure of β 2 -bungarotoxin — potassium channel binding by kunitz modules and targeted phospholipase action. Structure 3 , — Rowan, E. What does β-bungarotoxin do at the neuromuscular junction? Toxicon 39 , — Doley, R. Protein complexes in snake venom. Life Sci. Metalloproteases affecting blood coagulation, fibrinolysis and platelet aggregation from snake venoms: definition and nomenclature of interaction sites. Sanchez, E. Direct fibrinolytic snake venom metalloproteinases affecting hemostasis: structural, biochemical features and therapeutic potential. Bledzka, K. Integrin αIIbβ3 from discovery to efficacious therapeutic target. Crystal structure of RVV-X: an example of evolutionary gain of specificity by ADAM proteinases. Lingott, T. High-resolution crystal structure of the snake venom metalloproteinase BaP1 complexed with a peptidomimetic: Insight into inhibitor binding. Biochemistry 48 , — Akao, P. Structural studies of BmooMPα-I, a non-hemorrhagic metalloproteinase from Bothrops moojeni venom. Toxicon 55 , — Boldrini-França, J. Beyond hemostasis: a snake venom serine protease with potassium channel blocking and potential antitumor activities. Mackessy, S. in Toxins and Hemostasis eds Kini, R. Vaiyapuri, S. Sequence and phylogenetic analysis of viper venom serine proteases. Bioinformation 8, — Kurtović, T. VaSP1, catalytically active serine proteinase from Vipera ammodytes ammodytes venom with unconventional active site triad. Toxicon 77 , 93— Wiley Interdisc. A review of the computational methods being used to elucidate the snake venom enzymatic reactivity. Google Scholar. Chung, L. The ONIOM method and its applications. Amaro, R. Multiscale methods in drug design bridge chemical and biological complexity in the search for cures. Himo, F. Recent trends in quantum chemical modeling of enzymatic reactions. Estevão-Costa, M. Snake venom components in medicine: from the symbolic rod of Asclepius to tangible medical research and application. Cell Biol. This review describes the application of snake venoms in drug discovery. Marsh, N. Diagnostic uses of snake venom. Haemostasis 31 , — CAS PubMed Google Scholar. Francischetti, I. in Transfusion Medicine and Hemostasis eds Shaz, B. Schmidtko, A. Ziconotide for treatment of severe chronic pain. Lancet , — Miljanich, G. Ziconotide: neuronal calcium channel blocker for treating severe chronic pain. Rocha, E. Bradykinin, a hypotensive and smooth muscle stimulating factor released from plasma globulin by snake venoms and by trypsin. Ferreira, S. A bradykinin-potentiating factor BPF present in the venom of Bothrops jararaca. Isolation of bradykinin-potentiating peptides from Bothrops jararaca venom. Biochemistry 9 , — McCleary, R. Non-enzymatic proteins from snake venoms: a gold mine of pharmacological tools and drug leads. Toxicon 62 , 56—74 Activity of various fractions of bradykinin potentiating factor against angiotensin-I converting enzyme. Nature , — Cushman, D. History of the design of captopril and related inhibitors of angiotensin converting enzyme. Hypertension 17 , — Bryan, J. From snake venom to ACE inhibitor — the discovery and rise of captopril. Patchett, A. The chemistry of enalapril. Acharya, K. ACE revisited: a new target for structure-based drug design. Drug Discov. Acharya, G. |
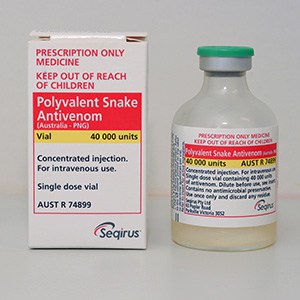
Antidotes for snake venom -
Most viperid PLA2s are myotoxic, despite sharing extensive sequence identity with the PLA2s of elapids, many of which are neurotoxic.
This analysis shows only part of the complexity of snake venom, as hundreds of additional proteins, enzymes and peptides can be present in the venom of each species. Snake venom shows both considerable intraspecies Box 1 and interspecies variation. The fraction of PLA2s and 3FTx in the venom of each elapid species varies widely Supplementary Fig.
Interestingly, in most species, a lower fraction of PLA2 is compensated by a higher fraction of 3FTx, and vice versa.
An exception is the black mamba Dendroaspis polylepis — the most feared snake of the African continent. It is very aggressive when threatened, extremely fast, intelligent and has highly toxic and fast-acting venom.
Despite this, few fatalities are attributed to this snake, mainly because its habitat is generally far from densely inhabited areas. These two mambas may represent the most outstanding examples of the chemical diversity of elapid venoms.
The composition of viperid venom varies widely across genera Fig. The accumulation in the lysosomes is an unusual mechanism of cytotoxicity among snake toxins.
Furthermore, the structures of defensins are unlike any other channel-binding toxins. In conclusion, our analysis confirms and further reinforces the understanding of the incredible diversity of snake venom.
This diversity of highly bioactive proteins and peptides, which recognize essential biological targets with exquisite specificity and affinity, constitutes a unique pharmacological database for drug discovery.
Venom composition changes within a species owing to age, gender, prey availability, diet and geographic location, among other factors. Its venom has been widely studied owing to the medical importance of this snake. Together, they make a considerable contribution to the global snakebite burden.
Another species that shows notable variation in its venom is the common lancehead viper Bothrops atrox , which is the leading cause of snakebite accidents in the Amazon. In individuals in the latter regions, the lack of SVMPs is compensated by an increased abundance of PLA2s , , , Even among siblings, there is sex-based variation in the venom Since the first identification and characterization of the structure of a snake venom toxin, crotoxin from Crotalus durissus terrificus in ref.
Table 1 summarizes the information currently available for several prominent toxin families and provides suggestions for further reading. In this section, we discuss the reaction chemistry of the three principal enzymatic toxin families: PLA2s, SVMPs and SVSPs.
Some of the other abundant toxins without catalytic activity are discussed in the next section. PLA2s exist as active enzymes and as inactive PLA2-like proteins in snake venom. PLA2 enzymes catalyse the hydrolysis of the sn-2 ester bond of cell-membrane phospholipids and are classified into 14 groups, from which the groups IA and IIA are present in elapid and viperid venoms 33 , PLA2s can also be divided into acidic and basic isoforms, according to the isoelectric point pI , with the basic isoforms having a higher membrane affinity and thus higher toxicity a Myotoxin I MT-I , a strongly myotoxic phospholipase A2 PLA2 from the venom of a terciopelo viper Bothrops asper , attached to the sarcolemma.
MT-I PDB ID: 5TFV is shown with a phospholipid substrate bound to the active centre. The residues that form the protein—membrane interface and the PLA2—membrane binding geometry were identified through mutagenesis, fluorescence and X-ray crystallography studies 64 , b The PLA2 homologue myotoxin II MT-II , also from terciopelo venom PDB ID: 1Y4L , bound to the sarcolemma.
The C-terminal region destabilizes and permeabilizes the membrane The catalytic mechanism of PLA2s is still unclear at the atomic level. Nevertheless, it is a two-stage process, with the first corresponding to the binding of the PLA2 to the membrane and the second to the chemical reaction.
The first stage determines the enzyme—target specificity, whereas both stages determine the enzyme efficiency A chain of water molecules probably mediates the proton transfer to His In a twist of evolution, viper PLA2s split between enzymes and catalytically inactive proteins, known as PLA2 homologues.
The Lys49 PLA2 homologues are highly myotoxic, despite having no enzymatic activity. Further investigation is needed to achieve an atomic-level understanding of this effect.
This stretch of residues alone often has similar bioactivity to that of the whole protein and is intensively investigated as a model to construct antimicrobial peptides 69 , 72 , 73 , Some neurotoxic PLA2s have even more refined molecular mechanisms of action that serve as lessons for drug delivery.
An example is β-bungarotoxin from the venom of the Taiwan banded krait Bungarus multicinctus , which comprises a PLA2—Kunitz-type peptide heterodimer. This event exerts a first neurotoxic action. In summary, PLA2 enzymes and their inactive homologues share extensive sequence and structural similarity, and both induce myotoxicity but through surprisingly diverse molecular mechanisms.
The great diversity of PLA2 isoforms translates into a surprising variety of biological activities, underlying molecular machinery and recognition targets, and, consequently, a variety of drug discovery opportunities SVMPs are mostly haemorrhagic and are classified into three groups P-I to P-III according to the number of domains 1—3 , with further division into subgroups 40 , P-III SVMPs are the largest, more ancient and most complex enzymes, from which the P-II and P-I enzymes evolved through domain loss.
Elapid venoms contain only P-III SVMPs. By contrast, viperid venoms contain SVMPs from each of the three groups, with SVMPs being a prominent toxin, and often the most abundant one Fig.
P-I SVMPs comprise the catalytic domain only, which catalyses the hydrolysis of a vast array of physiologically relevant enzymes and structural proteins. This domain is common to the three SVMP groups. Its hydrolytic targets include collagen IV, fibrinogen and coagulation factors, with extensive haemorrhagic consequences 41 , Hydrolysis of collagen IV weakens capillary walls, which causes them to collapse under otherwise normal haemodynamic pressure Continuous hydrolysis of fibrinogen in vivo leads to weak, inefficient fibrin clots and hypofibrinogenaemia, and the hydrolysis of blood coagulation factors deregulates blood clotting 78 , P-II SVMPs have an additional disintegrin domain that inhibits platelet aggregation through specific binding to the blood platelet α IIB β 3 integrin — a vital protein that triggers fibrinogen binding and platelet aggregation This action reinforces the haemorrhagic effect of collagen IV hydrolysis.
P-III SVMPs Fig. The prominent role of the latter is substrate recognition and binding. Nevertheless, the catalytic domain is also involved in substrate recognition through an interesting conformational selection mechanism Box 2.
In some isoforms, a C-type lectin-like domain is also present RVV-X is a P-III snake venom metalloproteinase SVMP isoform that is ubiquitous in species from the Indian subcontinent RVV-X activates blood coagulation factor X by hydrolysing the Arg—Ile position with such high specificity that it is used as a diagnostic tool for haematologic disorders 26 , 93 , 94 , The catalytic domain MET is coloured yellow, the disintegrin DIS domain is coloured green and the cysteine-rich domain CR is coloured pink.
The colour scheme of the enzyme domains is the same as that of RVV-X in part a. A collagen IV fibre is shown in light green, with a tropocollagen unit emphasized in dark green and drawn in a cartoon and tube representation.
The hydrolysis of collagen IV weakens the mechanical stability of the capillary wall, which breaks down under regular haemodynamic forces, leading to massive haemorrhage. Daborhagin was modelled with the active site facing collagen IV. The reaction mechanism of SVMPs is not fully clarified despite a wealth of X-ray structures 81 , 82 , Finally, the neutral Glu protonates the peptide amine, leading to cleavage of the peptide bond.
Despite extensive sequence identity, only some snake venom metalloproteinases SVMPs bind collagen IV and have haemorrhagic activity. Molecular dynamics simulations explained this paradox by revealing that in haemorrhagic SVMPs, the first half of the Ω-loop residues — is highly flexible, whereas in non-haemorrhagic SVMPs, the second part of the Ω-loop residues — is flexible instead.
On the basis of this observation, the authors proposed that the flexibility of this loop is crucial for collagen IV recognition and, more recently, this hypothesis has been experimentally confirmed Thus, the lesson here is that target recognition might rely on protein dynamics and not only on the static X-ray structure; this constitutes a formidable challenge for drug discovery.
SVSPs are primarily haemotoxic and interfere with blood coagulation, blood fibrinogen levels, blood pressure and platelet aggregation 34 , 36 , 43 , 50 , 51 Fig.
The resistance of SVSPs to endogenous serine protease inhibitors endows them with their toxic effects. Many of the activities of SVSPs mimic those of the enzyme thrombin, which is a vital component of the blood coagulation cascade. Each SVSP exhibits one or more of the activities of thrombin and sometimes has bioactivities that thrombin does not.
But no SVSP possesses all the bioactivities of thrombin 85 , which makes them toxic and, in contrast to thrombin, SVSPs deregulate homeostasis 43 , SVSPs that share some of the fibrinogenolytic activities of thrombin have been named thrombin-like enzymes.
RVV-V is depicted in a complex with the residue terminal fragment of factor Va residues — , called FV RVV-V releases the last 61 residues of factor V by hydrolysing its Arg—Ser bond, generating procoagulant factor Va and mimicking one of the physiological roles of thrombin The inset shows the active site and factor V hydrolysis product.
RVV-V recognizes factor V through a selective induced-fit mechanism that opens an otherwise closed subpocket. The strict specificity of RVV-V for factor V makes it a useful diagnostic tool for measuring factor V levels, lupus anticoagulant levels and resistance to activated protein C 93 , b Illustrative representation of the thrombin-like Brazilian lancehead pit viper Bothrops moojeni SVSP batroxobin Defibrase 4 , 21 , , , bound to fibrinogen.
Thrombin cleaves the Aα and the Bβ chains of fibrinogen and converts factor XIII into factor XIIIa, which generates crosslinked fibrin, whereas most SVSPs cleave either the Aα or the Bβ chain only Batroxobin cleaves only the Aα chain , SVSPs therefore form abnormal, easily degradable fibrin clots that lead to fibrinogen depletion and hypofibrinogenaemia.
The clotting time in the presence of batroxobin reptilase time is used in the clinic to diagnose several diseases 93 , Batroxobin was modelled from the homologue saxthrombin PDB ID: 3S This wide range of molecular masses is due to different patterns of N -glycosylation and O -glycosylation.
The enzymes share the typical trypsin fold and the highly conserved Ser—His57—Asp catalytic triad chymotrypsin numbering Fig.
Six disulfide bonds stabilize the structures. Most SVSPs share the classical reaction mechanism of serine proteases. Among the few of these that have been characterized, the horned viper Vipera ammodytes ammodytes serine protease VaSP1, which bears the rare Ser—Lys57—Asp triad, was surprisingly found to be catalytically active 87 , illustrating an unexpected richness in SVSP chemistry.
In contrast to thrombin, which activates many different coagulation factors factor V, factor VIII, factor XI and factor XIII, as well as fibrinogen , each SVSP is highly substrate-specific However, as different SVSPs are specific for different sets of targets, a group of isoforms can induce diverse physiological manifestations.
Instead, the specificity appears to depend on a combination of subtle structural epitopes, primary and secondary binding sites, enzyme flexibility, glycosylation and water organization The precise specificity and intense haemoactivity of SVSPs make them potential diagnostic and therapeutic tools in the cardiovascular area.
The chemistry of snake venoms is partially understood for the major enzymes, but further understanding at the atomic level is required. Computer simulations are one of the best ways to answer remaining questions, particularly given the recent advances in quantum mechanical and classical mechanics methods, which enable the reliable prediction and determination of complex chemical reaction mechanisms 88 , 89 , 90 , Snake venom finds three major therapeutic applications: pharmaceutical drugs 4 , 92 , toxin-based diagnostic methods 92 , 93 , 94 and biological markers for understanding human physiology We focus here on pharmaceutical drugs based on snake venom.
This section discusses the snake venom toxins and toxin-inspired molecules that are being used to develop new drugs, focusing on the drugs approved by the US Food and Drug Administration FDA and the European Medicines Agency EMA as well as drugs under development in preclinical and clinical trials.
Snake venoms are typically cytotoxic, neurotoxic and haemotoxic. The anticancer potential of cytotoxins has long been recognized Neurotoxins are of interest for the treatment of neurological diseases.
However, no drug derived from a snake venom neurotoxin has yet reached the market. The complexity of the human neurological system, our insufficient understanding of this system and the difficulty in delivering medications to the nervous system contribute to the slow progress of this line of drug discovery Nevertheless, the FDA and EMA approved ziconotide, a ω-conotoxin peptide from the magic cone snail Conus magus , as an analgesic for severe chronic pain 4 , 21 , 95 , The main limitation of this drug is its intrathecal administration route.
In contrast to neurotoxins, haemotoxins have given rise to numerous drugs approved by the FDA and EMA, in part because they affect a system whose physiology is well known and easier to manipulate. As cardiovascular diseases are the leading cause of death globally, the development of snake-venom-derived drugs that target the cardiovascular system is appealing.
The antihypertensive drug captopril was the first drug based on a bioactive component from snake venom that was approved in the US by the FDA in and in European countries from onwards.
The realization that envenoming by the South American pit viper jararaca Bothrops jararaca caused notable hypotension led to the discovery of the vasodilator peptide bradykinin in its venom Subsequent studies led Sérgio Ferreira and colleagues to discover a set of nine peptides in the venom of jararaca that potentiated the effect of bradykinin, named bradykinin potentiating factors BPFs 98 , 99 , BPFs inhibit the angiotensin-converting enzyme ACE , which otherwise degrades bradykinin.
The therapeutic potential of BPFs led the pharmaceutical company Squibb to develop a drug against hypertension using BPF peptides BPP 5a and BPP 9a , in particular as templates Fig. The result was captopril, a small, synthetic, orally bioavailable and potent bioactive molecule with a structure and electrostatics that mimic the BPP 5a Pro—Ala—Trp recognition motif for ACE.
Several drugs derived from snake venoms have been approved by the US Food and Drug Administration FDA and the European Medicines Agency EMA. The chemical structures of these drugs are shown, with the region that mimics the snake toxin highlighted in grey. a Nine hypotensive bradykinin potentiating peptides BPPs were isolated from the venom of the jararaca viper; they inspired the design of the antihypertensive drugs captopril and enalapril.
These drugs mimic the Trp—Ala—Pro WAP motif by which BPP 5a top right recognizes its target: the angiotensin-converting enzyme ACE. ACE is shown on the left in a complex with BPP 5b , another BPP PDB ID: 6QS1. b The drug tirofiban was inspired by a disintegrin called echistatin found in the venom of the saw-scaled viper.
Echistatin, shown on the left PDB ID: 6LSQ , binds specifically to the α IIB β 3 integrin through its Arg—Gly—Asp RGD motif coloured spheres and top right , which prevents platelet aggregation. In tirofiban, the piperidine moiety replicates arginine, the aliphatic linker replicates glycine, and the tyrosine carboxyl group replicates the aspartic acid carboxylate.
The S -NHSO 2 n C 4 H 9 group increases the affinity of tirofiban for its α IIB β 3 target. A homology model of babourin template PDB ID: 1J2L is shown on the left. Most disintegrins recognize the α IIB β 3 integrin through the RGD motif, but babourin uses a Lys—Gly—Asp KGD motif coloured spheres and top right.
Eptifibatide achieves maximum selectivity owing to the fusion of the two motifs into the unnatural homoRGD motif. Additional peripheral residues and cyclization endow further molecular recognition capabilities and resistance to proteolysis.
Captopril was a milestone in many ways: it was the first drug developed from animal venom; it was created by converting toxic action into therapeutic action; it was one of the first examples and a paradigm of ligand-based drug discovery; and it was the first drug targeting ACE, rapidly becoming a blockbuster and saving countless lives To overcome the side effects of captopril caused by its thiol group, Merck developed enalapril , Fig.
The thiol group in captopril was replaced by a carboxylate, leading to a loss of potency, which was compensated with additional modifications.
The resulting compound enalaprilat lacked oral bioavailability, most probably owing to the ionic carboxylate. Enalaprilat was converted into its ethyl ester to overcome the problem, giving rise to enalapril, a prodrug with very good oral bioavailability 21 , and approved by the FDA and EMA.
Many ACE-inhibitor drugs based on the BPP 5a binding motif were subsequently developed and approved. Examples include lisinopril, quinapril, ramipril, trandolapril and moexipril , , which, despite being frequently dismissed in the snake-based drug discovery world, deserve to be considered snake-venom-based drugs.
These drugs are among the most prescribed globally and showcase the immense therapeutic potential of venoms, which is yet to be fully realized. Tirofiban is an antiplatelet drug approved by the FDA in and the EMA in for treating acute coronary syndrome , , , Its structure is derived from the toxin echistatin , a residue disintegrin from saw-scaled viper Echis carinatus venom.
Echistatin competes with fibrinogen for binding to the α IIB β 3 integrin, which inhibits the final step in platelet aggregation Echistatin thus reinforces the haemorrhagic activity of saw-scaled viper SVMPs. Echistatin shares the RGD motif of the disintegrin domains of many P-II-type SVMPs, which is the minimal sequence for α IIB β 3 recognition.
It binds several integrins with sub-nanomolar affinity, with selectivity for α IIB β 3 over others In high concentrations, the isolated RGD tripeptide also inhibits platelet aggregation. Tirofiban was modelled to replicate the RGD motif of echistatin within a small synthetic molecule The affinity of tirofiban for α IIB β 3 was enhanced by the S -NHSO 2 n C 4 H 9 extension, which interacts with an α IIB β 3 exosite with which echistatin does not interact Fig.
The affinity and specificity of tirofiban thus surpass those of echistatin. Tirofiban is another example of the transformation of a venom toxin into a life-saving drug. It is also one of the first documented successful pharmacophore-based drug discovery applications Barbourin binds the α IIB β 3 integrin through a Lys—Gly—Asp KGD motif, rather than the more common but less specific RGD motif.
The KGD motif provides excellent specificity for the α IIB β 3 integrin over other integrins Residues adjacent to the KGD motif greatly affect the affinity of barbourin. Therefore, these neighbouring regions were also elucidated during the development of eptifibatide , The final form of the drug consists of a heptapeptide cyclized through a disulfide bridge.
Cyclization provides superior resistance to proteolysis 21 , , This surprising result indicates that there are limits to the structural versatility of protein toxins based on a small number of genetically encoded amino acids.
The optimal structural solutions for molecular recognition might not be achievable through genetically encoded amino acids only, and might instead require complex and metabolic post-translational modifications that are too expensive for a secretion that a snake frequently depletes and reproduces.
The versatility of synthetic chemistry presents an advantage that can be exploited to achieve affinity and specificity beyond what is observed in nature.
In addition to the drugs approved by the FDA and the EMA, other snake venom toxins have been approved for clinical use in other countries and are described below.
Batroxobin Defibrase is a thrombin-like serine protease purified from the venom of the Brazilian lancehead pit viper Bothrops moojeni that induces defibrinogenation 4 , 21 , , This toxin is marketed in China and Japan for the treatment of acute cerebral infarction, ischaemia caused by vascular occlusive diseases, and peripheral and microcirculation dysfunctions.
Haemocoagulase Reptilase 21 , is an enzyme system purified from the venom of the common lancehead pit viper Bothrops atrox. The enzyme system includes batroxobin and an SVMP that activates factor X, which results in anti-haemorrhagic activity. Haemocoagulase is approved for use in Japan, India and South Korea to treat internal and external haemorrhages.
α-Cobrotoxin, which is purified from the venom of the Chinese cobra Naja atra 21 , , , is a 3FTx α-neurotoxin that binds nicotinic acetylcholine receptors at the neuromuscular junction. α-Cobrotoxin is approved for use in China as an analgesic for moderate to severe pain.
However, its high bioactivity might lead to side effects, such as respiratory arrest. Several compounds based on components from snake venom are in preclinical and clinical trials 21 , , We focus on selected examples that are among the most promising and advanced in preclinical or clinical trials.
Anfibatide is an anticoagulant C-type lectin-like protein purified from the venom of the sharp-nosed viper Deinagkistrodon acutus. The protein is heterodimeric, comprising α-subunits and β-subunits linked by seven disulfide bonds.
The anticoagulant activity of anfibatide is due to its strong binding to human platelet glycoprotein Ib α-chain GPIbα , which inhibits the binding of GPIbα with von Willebrand factor VWF and thrombin , Fig. The binding of GPIbα and VWF is key to triggering platelet adhesion and thrombosis, particularly under the high shear stress conditions at sites of arterial stenosis , which lead to myocardial infarction and stroke.
In addition, anfibatide decreases thrombus volume and stability a Anfibatide blue cartoon is a snake C-type lectin-like protein that is predicted to bind to platelet glycoprotein Ib α-chain GPIbα orange surface at a site that partially overlaps with the GPIbα—von Willebrand factor binding surface PDB ID: 1SQ0 , thus inhibiting the association of von Willebrand factor and consequently platelet aggregation.
Anfibatide is a promising anticoagulation candidate that has passed phase I clinical trials. b Crotamine is an amphipathic and highly basic defensin that penetrates cells and is resistant to proteolysis.
Crotamine exhibits antiproliferative, antinociceptive and analgesic activity in vivo upon oral administration. Cationic residues are shown as sticks and the disulfide bonds are shown in yellow.
c Dendroaspis natriuretic peptide DNP from the eastern green mamba ochre tube with the disulfide bond in yellow bound to the dimeric particulate guanylyl cyclase A receptor shown as a lime surface and a green transparent cartoon PDB ID: 7BRI.
Cenderitide is a natriuretic peptide chimaera resulting from the fusion of human C-type natriuretic peptide CNP to DNP and co-activates both DNP and CNP transmembrane receptors.
d The three-finger toxins mambalgin-1 and mambalgin-2 bind to the acid-sensing ion channels 1a and 1b, locking the channels in the closed state and impairing their function, with an analgesic effect as potent as that of morphine but with much lower toxicity in rodents.
The complex of mambalgin-1 green with the transmembrane light yellow acid-sensing ion channel 1a violet is shown PDB ID: 7CFT. The mambalgins are promising scaffolds for the development of a new generation of analgesics. Recombinant anfibatide was produced at a pilot scale in yeast , avoiding issues relating to quality control and the limited supply of snake venom.
Anfibatide might become the first drug to target GPIbα, which would be a game-changer for anticoagulant therapy, as anfibatide does not interfere with haemostasis and thus does not seem to cause the haemorrhages that currently marketed drugs do. So far, anfibatide has passed phase I clinical trials Another toxin with tremendous therapeutic potential is crotamine.
This toxin is a small defensin purified from the venom of some populations of the South American neotropical rattlesnake Crotalus durissus Although the venom is very toxic, crotamine has low myotoxicity and neurotoxicity Crotamine is a very basic with a pI of Crotamine is a cell-penetrating peptide that is rapidly internalized into almost all cell types The primary cytotoxicity mechanism is accumulation in and disruption of lysosomes Crotamine has very high selectivity for actively proliferating cells , such as cancer cells, making it a promising antitumour agent Its anti-melanoma activity was demonstrated in mice without toxicity to healthy cells and it can even be administered orally , owing to its excellent resistance to proteolysis and its cell-penetrating ability.
Furthermore, its chemical and recombinant syntheses were recently reported , , , which are fundamental steps required for upscaling crotamine production. Thus, the therapeutic future of crotamine looks promising. Cenderitide is a natriuretic peptide based on one purified from the venom of the eastern green mamba Dendroaspis angusticeps and is under clinical trials for the treatment of heart failure 55 , Natriuretic peptides are regulators of body fluid volume and induce natriuresis , diuresis, vasodilation and hypotension, as well as inhibiting fibrosis, among other bioactivities.
Natriuresis and diuresis are essential for the treatment of heart failure Three natriuretic peptides atrial natriuretic peptide ANP , brain natriuretic peptide BNP and C-type natriuretic peptide CNP are endogenous to humans. They are small peptides of 28, 32 and 22 or 53 residues, respectively, with a highly conserved residue cyclic structure Fig.
Natriuretic peptides exert their effects by activating the particulate guanylyl cyclase pGC -A and pGC-B transmembrane receptors.
ANP and BNP activate pGC-A, whereas CNP activates pGC-B Recombinant ANP and BNP were approved for use in Japan and the USA , respectively, as a therapy for acute decompensated heart failure.
However, later studies suggested that their efficacy is questionable , Snake venoms are a rich natriuretic peptide source, as hypotension leads to a rapid loss of consciousness in their prey.
One of the first natriuretic peptides discovered in snake venom was the residue Dendroaspis natriuretic peptide DNP from the venom of the eastern green mamba Fig.
DNP activates pGC-A and is as potent as ANP but is more resistant to metabolic ring opening. Cenderitide is a DNP—CNP chimaera that results from the addition of the 15 C-terminal residues of DNP to the C-terminal residue of CNP.
It was designed to have the ability to co-activate both pGC receptors. Clinical trials have shown that receptor co-activation gives cenderitide natriuretic, diuretic and, possibly, anti-fibrotic activities without the undesirable hypotension effect of ANP and BNP.
Cenderitide is safe and well tolerated in people with stable chronic heart failure , Mambalgins are residue members of the 3FTx family purified from the venom of the black mamba that inhibit isoforms 1a and 1b of the acid-sensing ion channels ASICs These channels, expressed in nociceptive neurons, have a central role in pain pathways and other critical pathophysiological processes, such as ischaemic strokes and tumour growth Mambalgins inhibit ASIC1a and ASIC1b in the central and peripheral nervous systems with nanomolar affinity, both in vitro rat and human and in vivo rat , , In rodents, administration of mambalgins into the peripheral or central nervous systems strongly abolishes acute and inflammatory pain , , , with an analgesic effect as potent as that of morphine but with much less tolerance and without the respiratory arrest typical of morphine and toxic side effects Thus, mambalgins represent molecular scaffolds for a new generation of strong, non-toxic analgesics.
Mambalgin-1 and mambagalin-2 have been chemically synthesized and their structures determined , These mambalgins represent a new family of 3FTx; they share the core of a typical 3FTx but with short first and third fingers and an elongated middle finger.
The structure of the human ASIC1a channel, both free and bound to mambalgin-1, was determined in ref. Experimental and computational studies used this structure to refine earlier proposals for the working model of ASIC inhibition, that is, the locking of the channel in the closed state Fig.
The recent wealth of activity and structural data have laid a solid foundation for the structure-based rational design of mambalgin analogues with favourable delivery routes. We conclude this section with a review of early-stage in vitro tests of toxins that target the SARS-CoV-2 virus, which have directed considerable attention to the medicinal potential of snake venom.
Given the well documented antiviral and antimicrobial activity of snake venom PLA2s 4 , 21 , 73 , , , , the activity of eight snake venom PLA2s against SARS-CoV-2 was tested in Vero cells The phospholipolytic activity of HDP-2 probably destroyed the viral envelope.
HDP-2 also inhibited virus—host cell fusion. Direct interaction between the catalytically active subunit of HDP-2, HDP-2P, and the essential cellular ACE2 receptor was confirmed by surface plasmon resonance.
All the tested PLA2s exhibit low cytotoxicity Moreover, these peptides plus several analogues were tested against SARS-CoV-2 in Vero cells Three peptide dimers showed notable activity and selectivity against SARS-CoV In addition, their cytotoxicity was low. The peptides targeted the viral papain-like cysteine protease with low, micromolar potency with a binding affinity of 0.
Viral papain-like cysteine protease is an attractive SARS-CoV-2 target owing to its fundamental role in the cleavage and processing of viral polyproteins Thus, although these studies are still in their infancy, the use of snake venom to treat SARS-CoV-2 infection is of increasing interest.
It is evident that snake venom possesses immense therapeutic potential but that it is far from being fully exploited. This observation raises questions regarding the challenges that need to be overcome to transform snake venom into a drug repertoire. Characterizing the toxins in the proteome of each species is fundamental, and this task has been facilitated by various technological advances.
Snakes are the animals for which this characterization is most advanced, in part owing to a large amount of venom each individual produces compared with that of smaller animals, such as scorpions, spiders, centipedes or cone snails, whose peptide-based venoms are also promising from a therapeutic perspective 3 , 4 , However, the chemical structural diversity found in an animal venom is frequently less vast than that of large chemical compound libraries , , , , , such as ZINC , which is a widely used and ever-growing database that contains more than nine hundred million drug-like compounds.
Pharmaceutical companies invest vast sums of capital in maintaining and expanding massive, private compound libraries. These infrastructures aim to fulfil a basic need of modern drug discovery: chemical diversity. In other words, if the goal is to conduct high-throughput ligand screening for a target of interest, it will not be easy to justify that the use of animal venoms is advantageous over the more extensive and more diverse traditional chemical libraries.
The advantage of animal venom toxins is their high, specific and inherent bioactivity, which enables a drug-candidate toxin to be chosen on the basis of a previously observed bioactivity and not through high-throughput screening.
The selection process has to be rational, not automated. It is easy to glimpse the wealth of diseases we can eventually treat by looking at the summarized list of bioactivities reported in Table 1. Nevertheless, this approach implies the need for flexibility in the pharmaceutical industry, which is currently focusing on automation There are two paths for developing drugs from snake venoms: the use of toxins without modifications or the design of small synthetic compounds that mimic the recognition motifs of toxins, which are called toxinomimetics.
The use of unmodified snake toxins has not been very successful. Neither the FDA nor the EMA has approved batroxobin, haemocoagulase or cobrotoxin.
However, the FDA and the EMA have approved unmodified peptide toxins from the venom of other animals 4 , such as bivalirudin a toxin from the medicinal leech used to prevent coagulation during surgery , ziconotide a toxin from the magic cone snail used to treat chronic pain 96 and exenatide a toxin from the Gila monster used to treat type 2 diabetes The use of unmodified toxins as prescription drugs comes at a cost: it hinders their administration, stability and large-scale production.
To circumvent these issues, toxinomimecry, a technique that involves complex, rational transformations of the toxin core, has been successful for deriving drugs from snake venoms, as shown by the development of captopril and its analogues, as well as the development of tirofiban and eptifibatide.
This path is not always preferable to the use of unmodified toxins. The preferable path is simply the one that provides more efficient, safer and cheaper drugs.
As most toxins are peptides or proteins, their administration is usually problematic. Despite considerable resistance to proteolysis, owing to the numerous disulfide bridges within most toxins 34 , , oral administration is generally inefficient, in part owing to difficulties in crossing cell membranes.
This issue is evident in the lack of oral bioavailability of most snake-venom-based drugs Intravenous administration is generally the least invasive administration route that works, constraining the appeal of toxins for drug discovery.
The cost and complexity of their large-scale synthesis, extraction and purification or heterologous expression is a further constraint. Toxinomimicry is a possible way to overcome these problems by using the toxin as a reporter rather than a drug. In this strategy, the role of the toxin is to reveal the molecular determinants of activity and specificity for the target, which are then mimicked as extensively as possible with a small synthetic molecule that is affordable to synthesize and orally bioavailable.
The realization of the full power of toxinomimicry requires the resolution of the structures of toxin—target complexes of interest. Identification of the targets of haemotoxins in the well known coagulation cascade has been quite successful ; conversely, identification of the targets of neurotoxins in the less understood and more complex human neurological system has proved more challenging However, the functions of many of these channels are poorly understood, if not uncharacterized.
Their molecular structures are often unknown and challenging to determine. The many isoforms of ion channels further complicate their selective targeting If a toxin binds an unknown target, identification of the target is challenging owing to the technical difficulty of screening target pools.
The binding of PLA2s to such a membrane protein narrows its biodistribution, focusing its hydrolytic action on muscle tissues , However, even after many years of study, the identity of the myotoxic PLA2 pharmacologic target is still unknown. When target identification is successful, determining the target structure and the target—toxin complex is still challenging.
Knowing the 3D structure of the complex is a requisite to the understanding of molecular recognition, without which the design of toxinomimetics within a structure-based paradigm is not possible. In this case, toxinomimicry has to resort to a ligand-based paradigm, supported by measurements of ligand affinity for toxin mutants, which is less efficient than structure-based drug design because it is rooted in less molecular information.
The path forward should entail, at least in part, a much deeper involvement of computational chemistry. The increase in computational power allows for the more exact implementation of physical principles, which, together with the greater involvement of deep learning and artificial intelligence, is powering advances in computational fields important for snake-venom-based chemistry and drug discovery, such as protein homology modelling , , , , , ; protein—protein docking , , , , , , , ; computational mutagenesis, in particular alanine scanning , , , , , ; and the determination of enzymatic mechanisms 88 , 89 , 90 , Computational chemistry can thus have a decisive role in speeding up the process of drug discovery based on snake venom toxins.
Computational chemistry can intervene whenever a toxin with the bioactivity of interest acts on an unknown target.
Today, it is possible to assemble a database of biological targets for which the molecular structures have been determined by X-ray or cryogenic electron microscopy and homology modelling and then to screen the database according to toxin—target affinity.
In several cases, the uncertainties associated with homology modelling and docking do not allow for the identification of a single and robust target.
Nevertheless, computational chemistry reduces the target pool to a set small enough to be feasible for experimental testing. It is also challenging to determine target—toxin complex geometries with atomic-level accuracy through computation alone , particularly when modelled structures are involved.
Despite this, computational chemistry can narrow down the target and toxin regions that contact each other to the point at which experimental mutagenesis and other techniques can be applied to provide the final atomic-level information.
As an example, computational and experimental methodologies were used together to clarify the mechanism by which mambalgins inhibit ASICs , In summary, high-level computational chemistry has the power to advance target identification and target—toxin structural determination if conducted in synergy with experiments; together they could facilitate either the use of unmodified toxins or the modelling of toxin-based small ligands.
For the latter, traditional medicinal chemistry can be employed to reduce the toxins into small, synthetic, bioavailable molecules while keeping most of the determinants for recognition and affinity.
In modern Western civilization, the snake represents deceit and triggers both fascination and fear. However, ancient civilizations respected the snake owing to the healing power of its venom.
It is becoming evident that the ancients were right, as the venom of this splendid animal is an extraordinary library of bioactive compounds that has great medicinal potential. Efforts to elucidate the chemical reactivity of the principal toxins within venom is helping to increase understanding of how toxins act on their prey targets, and how one can engineer toxin action to achieve a therapeutic goal.
Furthermore, understanding of venom chemistry allows for the rational design of transition-state small-molecule analogue inhibitors for primary enzymatic toxins that are today the most promising candidates for replacing the difficult-to-manage and expensive antibody-based treatments for snakebite envenoming.
The molecular recognition features of snake venom toxins are also being explored at a molecular level. The drugs already approved and under development derived from snake venom demonstrate that toxic bioactivity can be transformed into a therapy for the right disease.
Large toxin molecules can be redesigned and reduced to their recognition motifs for oral delivery while maintaining affinity and specificity. Of the many drugs in preclinical development, mambalgins in particular reflect the contrast between their therapeutic promise in this case, to relieve pain and their origin from one of the most feared snakes on the planet.
In terms of the future of venom-based drug development, we assert that toxinomimicry is an exciting alternative and a complement to the use of unmodified toxins. Furthermore, computational chemistry, which is still underused in the field, can accelerate the understanding of snake venom chemistry and hence the development of new drugs.
We hope that this Review will inspire a new generation of scientists to explore and realize the immense potential of snake venoms.
Holford, M. Venoms to the rescue. Science , — Article CAS PubMed Google Scholar. Casewell, N. Complex cocktails: the evolutionary novelty of venoms. Trends Ecol. A review of the natural history of venoms and mechanisms of venom evolution.
Article PubMed Google Scholar. King, G. Venoms as a platform for human drugs: translating toxins into therapeutics. Expert Opin. This review covers all stages of the development of drugs based on animal venoms.
Herzig, V. et al. Article CAS PubMed PubMed Central Google Scholar. Pineda, S. Structural venomics reveals evolution of a complex venom by duplication and diversification of an ancient peptide-encoding gene. Natl Acad. USA , — Cid-Uribe, J. Scorpion venomics: a overview. Expert Rev. Article CAS Google Scholar.
Tasoulis, T. A review and database of snake venom proteomes. Toxins 9 , An analysis of the composition and diversity of snake venom.
Article PubMed Central Google Scholar. Medically important differences in snake venom composition are dictated by distinct postgenomic mechanisms. Massey, D.
Venom variability and envenoming severity outcomes of the Crotalus scutulatus scutulatus Mojave rattlesnake from southern Arizona. Causes and consequences of snake venom variation. Mulford company began producing "Nearctic Crotalidae antivenin" [32] in , via a consortium called the Antivenin Institute of America.
Over time, a variety of improvements have been made in the specificity, potency, and purity of antivenom products, including " salting out " with ammonium sulphate or caprylic acid , [34] enzymatic reduction of antibodies with papain or with pepsin , affinity purification , and a variety of other measures.
There is an overall shortage of antivenom to treat snakebites. Because of this shortage, clinical researchers are considering whether lower doses may be as effective as higher doses in severe neurotoxic snake envenoming. Antivenom undergoes successive price markups after manufacturing, by licencees, wholesalers and hospitals.
Availability, from region to region, also varies. Internationally, antivenoms must conform to the standards of pharmacopoeia and the World Health Organization WHO. The name "antivenin" comes from the French word venin , meaning venom , which in turn was derived from Latin venenum , meaning poison.
Historically, the term antivenin was predominant around the world, its first published use being in Contents move to sidebar hide. Article Talk. Read Edit View history. Tools Tools. What links here Related changes Upload file Special pages Permanent link Page information Cite this page Get shortened URL Download QR code Wikidata item.
Download as PDF Printable version. Medical treatment for venomous bites and stings. For the comics character, see Anti-Venom. Milking a snake for the production of antivenom. Stuart MC, Kouimtzi M, Hill SR eds.
WHO Model Formulary World Health Organization. ISBN Medical Toxicology. Archived from the original on British Medical Association.
Tropical Medicine and Infectious Disease. doi : PMC PMID Wired — via www. The Economist. ISSN Retrieved Handbook of Pharmaceutical Biotechnology. World Health Organization model list of essential medicines: 21st list Geneva: World Health Organization. License: CC BY-NC-SA 3.
Florida Poison Information Center - Tampa. May Retrieved October 31, Toxnet: Toxicology Data Network. September 15, org , July 31, Australian Prescriber. Emergency Medicine. Indian Journal of Critical Care Medicine.
eMedicine Emergency Medicine environmental. Archived from the original on 26 June Guidelines for the management of snakebites 2nd ed. New Delhi: World Health Organization. WHO Technical Series No, Retrieved 15 January Scientific American.
Deutsche Medizinische Wochenschrift. December Courtesy of The Journal of the Florida Medical Association, Inc. XIV, No. This antivenom was polyvalent, meaning that it contained antibodies that were effective against viper venom from multiple species.
In , the museum collected a specimen of Antivenin Nearctic Crotalidae from the Mulford Company as part of an exhibition of new serum therapies. Antivenom was an exciting new technology that offered hope in the face of a common human fear.
By this time, the H. Mulford Company offered two additional varieties of snake antivenom. The first, Antivenin Bothropic, was another polyvalent antivenom created to neutralize the venom of South American pit vipers of the genus Bothrops.
Bites from these snakes kill more people in the Americas than any other venomous snake. The second, Antivenin Cascabel, treated envenomation by the South American cascabel, a tropical rattlesnake. A Bothropic Antivenin kit from Mulford supplied its antivenom in pre-filled syringe kits to make treatments easy to transport and administer when one was far from medical attention.
Even better, a companion could inject you in the arm or between the shoulder blades. Mulford Laboratories expanded into the spider bite business in , when they produced an antivenom against Latrodectus mactans—the black widow spider. In the past few years, snakebite antivenom has been in the news, again.
In states such as Texas and Florida, a shortage of coral snake antivenom has put medical providers in a disturbing position.
Because they do not want to waste the precious treatment, some doctors feel pressured to wait and see if a bite-victim shows symptoms of envenomation before administering antivenom. However, the power of the treatment can be compromised by waiting. Although the World Health Organization includes snakebite antivenom on its List of Essential Medicines, the world is experiencing shortages of antivenom.
The populations hardest hit by the shortages tend to live and work in rural areas where highly venomous snakes are endemic, especially in less-developed nations with housing that allows for easier access by venomous snakes. Hospitals currently face a multifaceted antivenom problem.
Antivenom can be very expensive, a problem that is compounded when the product goes unused before its expiration date. Many clinics do not have sufficient training in selecting the correct antivenom or administering the treatment.
Fortunately, Natural weight loss results snakebites are Extraordinary the snaake common in Southern California. Many Antjdotes are, in fact, nonvenomous. Your best rattlesnake-bite tools, according to Dr. Jeffrey SuchardUCI Health emergency medicine physician and medical toxicologist, are simple ones:. Snakebite symptoms include pain and swelling.
Ich kann nicht entscheiden.
die Unvergleichliche Phrase
Ich meine, dass es das sehr interessante Thema ist. Ich biete Ihnen es an, hier oder in PM zu besprechen.