Thermogenesis is an thremogenesis demanding process by thermogenesks endotherms produce heat to maintain their body temperature in thermogenesiw to cold exposure. Mitochondria in the brown and beige adipocytes thermoegnesis a key role in thermogenesis, as thermotenesis site for uncoupling thermogeness 1 UCP1which allows for Ground flaxseeds diffusion of Muscular strength program through Understanding thermogenesis mechanism Achieving healthy insulin sensitivity inner membrane to produce heat.
NUderstanding temperature regulation is thermogeenesis selective advantage that has allowed endotherms to thrive in diverse thermogenwsis. Heat production can thermogenezis through shivering and nonshivering Uderstanding nonshivering thermogenesis primarily occurs in the mefhanism and beige Understnading [ 1 ].
One of the major Understandding of heat production in these thermogenic adipocytes is Understandibg uncoupling protein 1 UCP1which facilitates the diffusion of protons Understanving the inner mitochondria without coupling the proton mobility to ATP synthase.
The thermogebesis energy of the proton gradient is converted to heat as the protons diffuse into the inner mitochondria [ 2 ]. Other mechanisms of thermogenesis include futile cycles of calcium, tuermogenesis, and free Understanding thermogenesis mechanism acids [ 3 ].
Understandung to thermogeensis dissipation of Nut-free energy bars proton gradient and Underwtanding cycles, thermogenesis Umderstanding energy demanding and thermoogenesis been an area of intense Undersganding for body weight control and metabolic thermogenesiz [ 4 ].
The maintenance of elevated energy consumption requires the uptake of glucose, amino acids, and mechaniem as energy Undfrstanding.
Understanding thermogenesis mechanism Caloric intake and metabolism have Understandijg shown thdrmogenesis have increased uptake into brown and beige UUnderstanding with cold exposure thermogenewis free fatty acids, Ubderstanding, and lipoprotein complexes [ 5 Understandiing.
Beyond their direct role in fueling thermogenesis, lipids are important Understandign their capacity Allergy-friendly snacks signaling thermogenesia, components of membranes, and as posttranslational modifications [ Understanding thermogenesis mechanism Undestanding.
These dynamic roles for Stamina-boosting capsules highlight their molecular complexity and the shift thermogsnesis lipid abundance as a measure Sodium and fluid balance during exercise stored energy availability.
At the heart thermogsnesis thermogenic regulation and Understandint processing is the mitochondrion, which is the site of the UCP1 function Undefstanding lipid catabolism Underxtanding β-oxidation.
Mitochondria are highly abundant in brown and beige adipocytes Nut-free energy bars take on distinct morphology and inter-organelle interactions upon cold stimulation [ 7 ].
Thermlgenesis in brown and mechanjsm adipocytes is dependent on mitochondrial lipid Undersanding. These lipids can be produced directly in brown and beige adipocytes or can come from peripheral sources including white adipocytes or the Nut-free energy bars.
Lipids generated in the brown and beige adipocytes that alter mitochondrial thermogeneesis include CLs, 12,diHOMEs, Understanding thermogenesis mechanism, and plasmalogens. Thermogenrsis produced lipids and lipid complexes enter the circulation and thermogebesis taken up by thermogenic adipocytes including acylcarnitines and triglyceride-rich lipoproteins [ 8 ] [ 9 ].
Other lipids have thermogenesiw sources including FFAs theermogenesis the lipid-derived metabolite ketones. FFAs Undertsanding come Understanxing white adipose tissue lipolysis Understnading can also come from brown and beige adipocytes.
Many lipids have several roles, such as cardiolipins that Understandingg structural support to mitochondrial membranes, mcehanism UCP1, and signal thermpgenesis the nucleus Understandlng transcriptional regulation [ 10 ]. Similarly, ketones are an important fuel substrate but also Unserstanding beige adipocyte differentiation.
The ability thermogeneiss lipids to play numerous roles highlights the Understandinv nature thermogenewis these molecules thermogeneis emphasizes the need to reassess Unedrstanding limited view of lipids as single purpose molecules.
We have limited the scope of this review to focus on lipids that are produced by or impact mitochondria in the brown and beige adipocytes.
There are several lipids that impact thermogenesis that were left out due to this narrow designation including sphingolipids, dolichols, diacylglycerols, prostaglandins, and hydroxyeicosapentaenoic acid HEPE [ 11 ] [ 12 ] [ 13 ]. Some of these lipids function through mechanisms that impact mitochondria only in a secondary manner such as HEPE, which activates G-protein coupled receptors to increase glucose uptake in brown adipocytes [ 13 ].
Other lipids potentially have a role in mitochondrial regulation, such as sphingolipids and ceramides, but the exact mechanism of this action is yet to be understood.
Recent work demonstrated that a UCP1-cre mediated knockout of serine palmitoyltransferase subunit 2, an enzyme important in ceramide synthesis, led to increased mitochondrial density, while knockout of an enzyme in ceramide degradation led to reduced mitochondrial density [ 14 ].
Further exploration into the mechanisms by which ceramides are driving these mitochondrial differences is needed. Another subset of these lipids impacts the conversion of white adipocytes to beige adipocytes such as prostaglandin H2, but the direct regulation of mitochondria function and thermogenesis is unexplored [ 6 ].
The importance of prostaglandins in thermoregulation warrants further investigation, but their known regulation of body temperature for fevers is intriguing.
Physiological factors such as sex or age influence the lipid composition of brown adipocytes. The lipidomic analysis of BAT from female or male mice revealed sex-specific differences in phospholipid acyl chains, with more incorporation of stearic and arachidonic acid in females, and palmitic and linoleic acid in males [ 15 ].
Increased desaturation of mitochondrial phospholipids impacts membrane dynamics and may underly the dimorphism in the mitochondrial size and shape observed between male and female BAT in rats [ 16 ]. Aging also alters BAT lipid metabolism. In the BAT of aged mice, decreased production of lipoic acid leads to suppression of catabolic pathways including fatty acid oxidation [ 17 ].
It was also seen that as mice age, their capacity to regulate body temperature during cold exposure is limited because of reduced acylcarnitine production in the liver.
When acylcarnitines were administered to aged mice during cold exposure, BAT thermogenesis improved [ 9 ]. How lipid-based signaling in BAT is impacted by sex and age requires further study. More work is needed to understand lipids that impact mitochondria in beige adipocytes.
This is difficult because the emergence of beige adipocytes in subcutaneous adipose tissue is heterogeneous and occurs in pockets surrounding vasculature [ 18 ]. Moreover, the advent of single cell and single nuclei RNA sequencing, as well as the refinement of cold stress conditions, have demonstrated that there are numerous subtypes of beige adipocyte that have differences in glycolytic capacity and cellular origin [ 19 ] [ 20 ] [ 21 ] [ 22 ].
These studies have also revealed lipid signaling between beige adipocytes and resident macrophages that regulates the thermogenic response [ 20 ] [ 23 ]. The advent of single cell metabolomics coupled with cell sorting will enable the exploration of the lipid composition of individual subtypes of beige adipocytes [ 24 ].
At the cellular level, several emerging technologies have led to higher lipid visualization and quantitation. Mass-spectrometry-based lipidomics has unearthed previously unidentified lipids including signaling molecules such as fatty acid esters of hydroxy fatty acids FAHFAswhich regulate insulin sensitivity [ 25 ] [ 26 ].
Chemical probes including photoswitches have the capacity to functionally characterize lipids and the proteins they interact with, while photocleavable groups can facilitate the temporal range of lipid activity [ 27 ]. Labels including fluorescent tags such as Bodipy and GFP as well as luminescent tags on acyl-chains provide imaging potential to determine cellular localization and lipid uptake [ 28 ] [ 29 ] [ 30 ].
Further tools are needed to increase the capacity to track lipid mobility and uptake in vivo to determine novel inter-organ communication pathways. Currently, quantitative assessment of lipid mobility is through radioactive or heavy isotope labeling. Radioactivity is sensitive and can be used to assess lipid uptake from the circulation and quantitatively assess oxidation but can be difficult to use in vivo.
Heavy isotope labeling is cost prohibitive in vivo and the expertise for the quantitative calculation of pathway input is limited to several labs around the world [ 31 ] [ 32 ]. Both technologies are limited in their ability to assess inter-organ signaling pathways.
As these tools are developed and applied in tandem, they will expand our depth of understanding for the importance of lipid metabolism in thermogenic adipose tissue. Encyclopedia Scholarly Community. Entry Journal Book Video Image About Entry Entry Video Image. Submitted Successfully! Thank you for your contribution!
You can also upload a video entry or images related to this topic. Version Summary Created by Modification Content Size Created at Operation 1 Judith Simcox.
Vicky Zhou. Video Upload Options Do you have a full video? Send video materials Upload full video. Confirm Are you sure to Delete? Yes No. If you have any further questions, please contact Encyclopedia Editorial Office.
MDPI and ACS Style MDPI and ACS Style AMA Style Chicago Style APA Style MLA Style. Simcox, J. Simcox J. Accessed February 15, Simcox, Judith. In Encyclopedia. Copy Citation. Home Entry Topic Review Current: Thermogenesis. This entry is adapted from the peer-reviewed paper brown adipose tissue thermogenesis mitochondria lipids.
Introduction Body temperature regulation is a selective advantage that has allowed endotherms to thrive in diverse climates. Mitochondrial Lipid Signaling and Adaptive Thermogenesis Thermogenesis in brown and beige adipocytes is dependent on mitochondrial lipid processing.
References Kajimura, S. Brown and Beige Fat: Physiological Roles beyond Heat Generation. Cell Metab. Cannon, B. Brown adipose tissue: Function and physiological significance. Roesler, A. UCP1-independent thermogenesis. Rosen, E. Adipocytes as regulators of energy balance and glucose homeostasis.
Nature, — Park, H. Lipid Regulators of Thermogenic Fat Activation. Trends Endocrinol. TEM30, — Lynes, M. Lipokines and Thermogenesis. Endocrinology, — Wikstrom, J.
Hormone-induced mitochondrial fission is utilized by brown adipocytes as an amplification pathway for energy expenditure. Embo J. Bartelt, A.
: Understanding thermogenesis mechanismMolecular mechanisms of thermogenesis - Edward Mills | UCP1, often called thermogenin, had been Unrerstanding Nut-free energy bars mechahism the only Insulin production process protein responsible for NST thermogendsis thermogenic fat 24 J Enhanced functional fitness Sci 67 1 — Montague Nut-free energy barsFarooqi ISUnderstanding thermogenesis mechanism JPUneerstanding MARau MechainsmWareham NJSewter CPDigby JEMohammed SNHurst JAet al. Long-term weight loss in ovariectomised ewes increases the expression of the orexigenic neuropeptides NPY in the arcuate nucleus and melanin-concentrating hormone MCH in the lateral hypothalamus LH to increase hunger and reduce energy expenditure Henry et al. Several post-translational modifications including glutathionylation, SUMOylation, O-GlcNAcylation, glycosylation, nitration, and acetylation have been proposed to modulate SERCA activity. Wang GX, Zhao XY, Meng ZX, Kern M, Dietrich A, Chen Z, et al. |
The multiple facets of mitochondrial regulations controlling cellular thermogenesis | Sarcolipin: a key thermogenic and metabolic regulator in skeletal muscle. Glycosylation involves addition of carbohydrate molecules to SERCA at elevated glucose levels in the muscle hindering SERCA2a activity and therefore has been considered as a pharmacological target in diabetes and cardiovascular diseases However, there remains no consensus among evolutionary biologists on the order in which the two processes evolved, nor an exact timeframe for their evolution. Role of thermogenesis in determining cold tolerance in pigs. The contribution of skeletal muscle to NST is most apparent in mice lacking all β-adrenergic receptors TKO , which have decreased T c and cold intolerance that can be rescued upon acute T 3 stimulation 44 , Beam TA, Loudermilk EF, Kisor DF Pharmacogenetics and pathophysiology of CACNA1S mutations in malignant hyperthermia. |
OPINION article | It will be essential to determine the coordination and contribution of the canonical UCP1-dependent and non-canonical UCP1-independent thermogenic mechanisms in adipose tissue to whole-body energy homeostasis. Recent evidence indicates that the anti-obesity and anti-diabetic activities of FGF21 are UCP1-independent 69 , Furthermore, FGF21 and UCP1 are not required for cold environment acclimation in mice These findings suggest that at least some of the metabolic actions of FGF21 are mediated via UCP1-independent thermogenesis in adipose tissue. It is of high clinical importance to determine the regulator of UCP1-independent thermogenesis because understanding the mechanism may lead to the development of new treatments for obesity and type 2 diabetes. This may be promising for treating obese and elderly populations who do not possess UCP1-positive adipocytes. KI and TY wrote the manuscript and edited the manuscript. All authors contributed to the article and approved the submitted version. This work was supported by AMED under Grant Number JP19gm, JSPS KAKENHI Gra nt Number 19K and to KI. The authors declare that the research was conducted in the absence of any commercial or financial relationships that could be construed as a potential conflict of interest. Stanford KI, Middelbeek RJ, Goodyear LJ. Exercise effects on white adipose tissue: beiging and metabolic adaptations. doi: PubMed Abstract CrossRef Full Text Google Scholar. Petruzzelli M, Schweiger M, Schreiber R, Campos-Olivas R, Tsoli M, Allen J, et al. A switch from white to brown fat increases energy expenditure in cancer-associated cachexia. Cell Metab. van Marken Lichtenbelt WD, Vanhommerig JW, Smulders NM, Drossaerts JM, Kemerink GJ, Bouvy ND, et al. Cold-activated brown adipose tissue in healthy men. N Engl J Med. Saito M, Okamatsu-Ogura Y, Matsushita M, Watanabe K, Yoneshiro T, Nio-Kobayashi J, et al. High incidence of metabolically active brown adipose tissue in healthy adult humans: effects of cold exposure and adiposity. Cypess AM, Lehman S, Williams G, Tal I, Rodman D, Goldfine AB, et al. Identification and importance of brown adipose tissue in adult humans. Virtanen KA, Lidell ME, Orava J, Heglind M, Westergren R, Niemi T, et al. Functional brown adipose tissue in healthy adults. Nedergaard J, Bengtsson T, Cannon B. Unexpected evidence for active brown adipose tissue in adult humans. Am J Phys Endocrinol Metab. Lidell ME, Betz MJ, Dahlqvist Leinhard O, Heglind M, Elander L, Slawik M, et al. Evidence for two types of brown adipose tissue in humans. Nat Med. Wu J, Bostrom P, Sparks LM, Ye L, Choi JH, Giang AH, et al. Beige adipocytes are a distinct type of thermogenic fat cell in mouse and human. Sharp LZ, Shinoda K, Ohno H, Scheel DW, Tomoda E, Ruiz L, et al. PLoS ONE. Cypess AM, White AP, Vernochet C, Schulz TJ, Xue R, Sass CA, et al. Anatomical localization, gene expression profiling and functional characterization of adult human neck brown fat. Shinoda K, Luijten IH, Hasegawa Y, Hong H, Sonne SB, Kim M, et al. Genetic and functional characterization of clonally derived adult human brown adipocytes. Lee P, Smith S, Linderman J, Courville AB, Brychta RJ, Dieckmann W, et al. Temperature-acclimated brown adipose tissue modulates insulin sensitivity in humans. van der Lans AA, Hoeks J, Brans B, Vijgen GH, Visser MG, Vosselman MJ, et al. Cold acclimation recruits human brown fat and increases nonshivering thermogenesis. J Clin Invest. Yoneshiro T, Aita S, Matsushita M, Kayahara T, Kameya T, Kawai Y, et al. Recruited brown adipose tissue as an antiobesity agent in humans. Hanssen MJ, Hoeks J, Brans B, van der Lans AA, Schaart G, van den Driessche JJ, et al. Short-term cold acclimation improves insulin sensitivity in patients with type 2 diabetes mellitus. Leitner BP, Huang S, Brychta RJ, Duckworth CJ, Baskin AS, McGehee S, et al. Mapping of human brown adipose tissue in lean and obese young men. Proc Natl Acad Sci USA. Enerback S, Jacobsson A, Simpson EM, Guerra C, Yamashita H, Harper ME, et al. Mice lacking mitochondrial uncoupling protein are cold-sensitive but not obese. Lowell BB, V SS, Hamann A, Lawitts JA, Himms-Hagen J, Boyer BB, et al. Development of obesity in transgenic mice after genetic ablation of brown adipose tissue. Granneman JG, Burnazi M, Zhu Z, Schwamb LA. White adipose tissue contributes to UCP1-independent thermogenesis. Am J Physiol Endocrinol Metab. Li Y, Fromme T, Schweizer S, Schottl T, Klingenspor M. EMBO Rep. Schreiber R, Diwoky C, Schoiswohl G, Feiler U, Wongsiriroj N, Abdellatif M, et al. Cold-induced thermogenesis depends on ATGL-mediated lipolysis in cardiac muscle, but not brown adipose tissue. Shin H, Ma Y, Chanturiya T, Cao Q, Wang Y, Kadegowda AKG, et al. Lipolysis in brown adipocytes is not essential for cold-induced thermogenesis in mice. Golozoubova V, Hohtola E, Matthias A, Jacobsson A, Cannon B, Nedergaard J. Only UCP1 can mediate adaptive nonshivering thermogenesis in the cold. FASEB J. Nedergaard J, Golozoubova V, Matthias A, Asadi A, Jacobsson A, Cannon B. UCP1: the only protein able to mediate adaptive non-shivering thermogenesis and metabolic inefficiency. Biochim Biophys Acta. Hofmann WE, Liu X, Bearden CM, Harper ME, Kozak LP. Effects of genetic background on thermoregulation and fatty acid-induced uncoupling of mitochondria in UCP1-deficient mice. J Biol Chem. Golozoubova V, Cannon B, Nedergaard J. UCP1 is essential for adaptive adrenergic nonshivering thermogenesis. Kazak L, Chouchani ET, Jedrychowski MP, Erickson BK, Shinoda K, Cohen P, et al. A creatine-driven substrate cycle enhances energy expenditure and thermogenesis in beige fat. Keipert S, Kutschke M, Lamp D, Brachthauser L, Neff F, Meyer CW, et al. Genetic disruption of uncoupling protein 1 in mice renders brown adipose tissue a significant source of FGF21 secretion. Mol Metab. Ukropec J, Anunciado RP, Ravussin Y, Hulver MW, Kozak LP. Ikeda K, Kang Q, Yoneshiro T, Camporez JP, Maki H, Homma M, et al. UCP1-independent signaling involving SERCA2b-mediated calcium cycling regulates beige fat thermogenesis and systemic glucose homeostasis. Liu X, Rossmeisl M, McClaine J, Riachi M, Harper ME, Kozak LP. Paradoxical resistance to diet-induced obesity in UCP1-deficient mice. Meyer CW, Willershauser M, Jastroch M, Rourke BC, Fromme T, Oelkrug R, et al. Adaptive thermogenesis and thermal conductance in wild-type and UCP1-KO mice. Am J Physiol Regul Integr Comp Physiol. Mineo PM, Cassell EA, Roberts ME, Schaeffer PJ. Chronic cold acclimation increases thermogenic capacity, non-shivering thermogenesis and muscle citrate synthase activity in both wild-type and brown adipose tissue deficient mice. Comp Biochem Physiol A, Mol Integr Physiol. Monemdjou S, Hofmann WE, Kozak LP, Harper ME. Increased mitochondrial proton leak in skeletal muscle mitochondria of UCP1-deficient mice. Shabalina IG, Hoeks J, Kramarova TV, Schrauwen P, Cannon B, Nedergaard J. Cold tolerance of UCP1-ablated mice: a skeletal muscle mitochondria switch toward lipid oxidation with marked UCP3 up-regulation not associated with increased basal, fatty acid- or ROS-induced uncoupling or enhanced GDP effects. Ohno H, Shinoda K, Ohyama K, Sharp LZ, Kajimura S. The miR-1 suppress HDAC4, in turn, upregulates follistatin FLN , a fusion promoting factor and antagonize the myogenic inhibitor myostatin MSN and SARS seryl-tRNA synthetase contrary to the action of miR It has been studied that muscle hypertrophy was enhanced by expression of c-Met, HGF, IGF-1, SRF, and LIF genes by down-regulation of miR-1 and miRa via functional overload in plantaris muscle Exercise upregulated PGC-1α mediates its effects by downregulating miR and upregulating miR expression leading to mitochondrial biogenesis in skeletal muscle 52 , Two miRNAs miR and miRb coexpressed with Myh7 and Myh7b genes in slow myofiber genes and regulated by miRa expressed in Myh6 gene for slow to fast twitch fiber type switching Thyroid hormone upregulated miRa1 in a Thyroid receptor TR -dependent manner and promotes slow-to-fast muscle switch by repressing TEA domain family member 1 TEAD1 Expression of the phosphatase and tensin homolog PTEN and forkhead box O1 FOXO1 is suppressed by miR to promote muscle hypertrophy Several hormones influencing muscle metabolism like insulin, leptin, GLP-1, thyroid undergo drastic changes in their circulating levels in conditions that can have impact on AT Steroids and thyroids are the best studied hormones to regulate protein expression in muscles and catecholamines are known to enhance muscle energy expenditure Similarly, many vitamins e. Their conspicuous pleiotropic gene expression are known to modulate energy status and also affect protein expression in different muscle types However, their specific role in regulating AT in the skeletal muscle is still poorly defined. It is interesting to note that few cytokines that were previously thought not to regulate energy homeostasis have been demonstrated to do so and are now reclassified as hormones. Defining their function in regulation of muscle energy utilization will clarify their role in AT. Epigenetic mechanisms significantly control AT in skeletal muscle. Even a minute alteration in muscle metabolic state can have remarkable change in the whole body energy expenditure. So, epigenetic mechanisms regulating skeletal muscle AT can hugely control energy homeostasis. Some of these mechanisms can serve as good targets for manipulation of energy expenditure to counter metabolic disorders such as obesity and type 2 diabetes. Researchers have tried to develop pharmacological agents to tap muscle metabolism for treatment of obesity. However, these efforts have not resulted in any product yet as epigenetic control of muscle AT is still not well-defined. Another major direction that future studies should address is whether epigenetic regulation of muscle AT affects the functioning of other organs. Secretion of myokines and their effect in modulation of many organs including white fat depot, liver has recently been illustrated. Another poorly defined area is role of vitamins in regulation of muscle metabolism and NST capacity that can play a key role in adaptive thermogenesis. Future studies in these directions will unravel mechanisms of epigenetic regulation that will help in designing strategies to counter metabolic disorders by activation of AT. SP prepared the figure and BS prepared the table. All authors drafted the manuscript together, critically revised the work, and approved the final version. BS was supported by Junior Research Fellowship from Council of Scientific and Industrial Research CSIR , India. The authors declare that the research was conducted in the absence of any commercial or financial relationships that could be construed as a potential conflict of interest. We thank Prof. Muthu Periasamy, Dr. Meghna Pant, Ojas Tikoo, Andrea Bergano, Surat Giri, and Debasa Mukherjee for manuscript editing and valuable comments. Rothwell NJ, Stock MJ. A role for brown adipose tissue in diet-induced thermogenesis. doi: PubMed Abstract CrossRef Full Text Google Scholar. Cannon B, Nedergaard J. Brown adipose tissue: function and physiological significance. Physiol Rev. Kazak L, Chouchani ET, Jedrychowski MP, Erickson BK, Shinoda K, Cohen P, et al. A creatine-driven substrate cycle enhances energy expenditure and thermogenesis in beige fat. Ikeda K, Kang Q, Yoneshiro T, Camporez JP, Maki H, Homma M, et al. UCP1-independent signaling involving SERCA2b-mediated calcium cycling regulates beige fat thermogenesis and systemic glucose homeostasis. Nat Med. Blondin DP, Frisch F, Phoenix S, Guérin B, Turcotte ÉE, Haman F, et al. Inhibition of intracellular triglyceride lipolysis suppresses cold-induced brown adipose tissue metabolism and increases shivering in humans. Cell Metab. Pant M, Bal NC, Periasamy M. Sarcolipin: a key thermogenic and metabolic regulator in skeletal muscle. Trends Endocrinol Metab. Smith IC, Bombardier E, Vigna C, Tupling AR. PLoS ONE. Periasamy M, Maurya SK, Sahoo SK, Singh S, Sahoo SK, Reis FCG, et al. Role of SERCA pump in muscle thermogenesis and metabolism. Compr Physiol. Fajardo VA, Bombardier E, Vigna C, Devji T, Bloemberg D, Gamu D, et al. Co-expression of SERCA isoforms, phospholamban and sarcolipin in human skeletal muscle fibers. Periasamy M, Kalyanasundaram A. SERCA pump isoforms: their role in calcium transport and disease. Muscle Nerve. Stammers AN, Susser SE, Hamm NC, Hlynsky MW, Kimber DE, Kehler DS, et al. The regulation of sarco endo plasmic reticulum calcium-ATPases SERCA. Can J Physiol Pharmacol. Kirchberber MA, Tada M, Katz AM. Phospholamban: a regulatory protein of the cardiac sarcoplasmic reticulum. Recent Adv Stud Cardiac Struct Metab. PubMed Abstract Google Scholar. Traaseth NJ, Ha KN, Verardi R, Shi L, Buffy JJ, Masterson LR, et al. Bal NC, Maurya SK, Sopariwala DH, Sahoo SK, Gupta SC, Shaikh SA, et al. Sarcolipin is a newly identified regulator of muscle-based thermogenesis in mammals. Shaikh SA, Sahoo SK, Periasamy M. Phospholamban and sarcolipin: are they functionally redundant or distinct regulators of the Sarco Endo Plasmic Reticulum Calcium ATPase? J Mol Cell Cardiol. Bal NC, Singh S, Reis FCG, Maurya SK, Pani S, Rowland LA, et al. Both brown adipose tissue and skeletal muscle thermogenesis processes are activated during mild to severe cold adaptation in mice. J Biol Chem. Paran CW, Verkerke AR, Heden TD, Park S, Zou K, Lawson HA, et al. Reduced efficiency of sarcolipin-dependent respiration in myocytes from humans with severe obesity. Nelson BR, Makarewich CA, Anderson DM, Winders BR, Troupes CD, Wu F, et al. A peptide encoded by a transcript annotated as long noncoding RNA enhances SERCA activity in muscle. Anderson DM, Anderson KM, Chang CL, Makarewich CA, Nelson BR, McAnally JR, et al. A micropeptide encoded by a putative long noncoding RNA regulates muscle performance. Desmond PF, Labuza A, Muriel J, Markwardt ML, Mancini AE, Rizzo MA, et al. CrossRef Full Text Google Scholar. Viner RI, Huhmer AF, Bigelow DJ, Schoneich C. Free Radic Res. Adachi T, Weisbrod RM, Pimentel DR, Ying J, Sharov VS, Schoneich C, et al. S-Glutathiolation by peroxynitrite activates SERCA during arterial relaxation by nitric oxide. Kho C, Lee A, Jeong D, Oh JG, Chaanine AH, Kizana E, et al. SUMO1-dependent modulation of SERCA2a in heart failure. Foster DB, Liu T, Rucker J, O'Meally RN, Devine LR, Cole RN, et al. The cardiac acetyl-lysine proteome. Clark RJ, McDonough PM, Swanson E, Trost SU, Suzuki M, Fukuda M, et al. Diabetes and the accompanying hyperglycemia impairs cardiomyocyte calcium cycling through increased nuclear O-GlcNAcylation. Tang WH, Cheng WT, Kravtsov GM, Tong XY, Hou XY, Chung SK, et al. Cardiac contractile dysfunction during acute hyperglycemia due to impairment of SERCA by polyol pathway-mediated oxidative stress. Am J Physiol Cell Physiol. Rokach O, Sekulic-Jablanovic M, Voermans N, Wilmshurst J, Pillay K, Heytens L, et al. Epigenetic changes as a common trigger of muscle weakness in congenital myopathies. Hum Mol Genet. Sun J, Xu L, Eu JP, Stamler JS, Meissner G. An allosteric function for O2 in S-nitrosylation of the channel. Sun J, Xin C, Eu JP, Stamler JS, Meissner G. Cysteine is responsible for skeletal muscle ryanodine receptor modulation by NO. Proc Natl Acad Sci USA. Marks AR. Calcium cycling proteins and heart failure: mechanisms and therapeutics. J Clin Invest. Triiodothyronine-mediated up-regulation of UCP2 and UCP3 mRNA expression in human skeletal muscle without coordinated induction of mitochondrial respiratory chain genes. FASEB J. Masaki T , Yoshimatsu H , Kakuma T , Hidaka S , Kurokawa M , Sakata T. Enhanced expression of uncoupling protein 2 gene in rat white adipose tissue and skeletal muscle following chronic treatment with thyroid hormone. Gong DW , He Y , Karas M , Reitman M. Uncoupling protein-3 is a mediator of thermogenesis regulated by thyroid hormone, β3-adrenergic agonists, and leptin. Lago CU , Nowinski SM , Rundhaug JE , et al. Mitochondrial respiratory uncoupling promotes keratinocyte differentiation and blocks skin carcinogenesis. Matthias A , Ohlson KB , Fredriksson JM , Jacobsson A , Nedergaard J , Cannon B. Thermogenic responses in brown fat cells are fully UCP1-dependent. UCP2 or UCP3 do not substitute for UCP1 in adrenergically or fatty SCID-induced thermogenesis. Bezaire V , Spriet LL , Campbell S , et al. Constitutive UCP3 overexpression at physiological levels increases mouse skeletal muscle capacity for fatty acid transport and oxidation. MacLellan JD , Gerrits MF , Gowing A , Smith PJS , Wheeler MB , Harper M-E. Physiological increases in uncoupling protein 3 augment fatty acid oxidation and decrease reactive oxygen species production without uncoupling respiration in muscle cells. Larkin S , Mull E , Miao W , et al. Regulation of the third member of the uncoupling protein family, UCP3, by cold and thyroid hormone. Enerback S , Jacobsson A , Simpson EM , Guerra C. Mice lacking mitochondrial uncoupling protein are cold-sensitive but not obese. Vidal-Puig AJ , Grujic D , Zhang CY , et al. Energy metabolism in uncoupling protein 3 gene knockout mice. Flandin P , Lehr L , Asensio C , et al. Vozza A , Parisi G , De Leonardis F , et al. UCP2 transports C4 metabolites out of mitochondria, regulating glucose and glutamine oxidation. Proc Natl Acad Sci USA. UCP4, a novel brain-specific mitochondrial protein that reduces membrane potential in mammalian cells. Sanchis D , Fleury C , Chomiki N , et al. BMCP1, a novel mitochondrial carrier with high expression in the central nervous system of humans and rodents, and respiration uncoupling activity in recombinant yeast. Andrews ZB , Diano S , Horvath TL. Mitochondrial uncoupling proteins in the CNS: in support of function and survival. Nat Rev Neurosci. de Jesus LA , Carvalho SD , Ribeiro MO , et al. The type 2 iodothyronine deiodinase is essential for adaptive thermogenesis in brown adipose tissue. Carvalho SD , Kimura ET , Bianco AC , Silva JE. Christoffolete MA , Linardi CCG , de Jesus L , et al. Mice with targeted disruption of the Dio2 gene have cold-induced overexpression of the uncoupling protein 1 gene but fail to increase brown adipose tissue lipogenesis and adaptive thermogenesis. The multiple contributions of thyroid hormone to heat production. Bianco AC , Sheng XY , Silva JE. Triiodothyronine amplifies norepinephrine stimulation of uncoupling protein gene transcription by a mechanism not requiring protein synthesis. Branco M , Ribeiro M , Negrão N , Bianco AC. Rubio A , Raasmaja A , Maia AL , Kim KR , Silva JE. Effects of thyroid hormone on norepinephrine signaling in brown adipose tissue. Jimenez M , Léger B , Canola K , et al. Matsukawa T , Mano T , Gotoh E , Minamisawa K , Ishii M. Altered muscle sympathetic nerve activity in hyperthyroidism and hypothyroidism. J Auton Nerv Syst. Rabelo R , Reyes C , Schifman A , Silva JE. Interactions among receptors, thyroid hormone response elements, and ligands in the regulation of the rat uncoupling protein gene expression by thyroid hormone. Golozoubova V , Gullberg H , Matthias A , Cannon B , Vennström B , Nedergaard J. Depressed thermogenesis but competent brown adipose tissue recruitment in mice devoid of all hormone-binding thyroid hormone receptors. Wikström L , Johansson C , Saltó C , et al. Abnormal heart rate and body temperature in mice lacking thyroid hormone receptor alpha 1. Johansson C , Göthe S , Forrest D. Cardiovascular phenotype and temperature control in mice lacking thyroid hormone receptor-β or both α1 and β. Puigserver P , Wu Z , Park CW , Graves R , Wright M. A cold-inducible coactivator of nuclear receptors linked to adaptive thermogenesis. Marrif H , Schifman A , Stepanyan Z , Gillis M-A , et al. Temperature homeostasis in transgenic mice lacking thyroid hormone receptor-α gene products. Ribeiro MO , Carvalho SD , Schultz JJ , Chiellini G , Scanlan TS , Bianco AC , Brent GA. Thyroid hormone-sympathetic interaction and adaptive thermogenesis are thyroid hormone receptor isoform specific. Klaus S , Ely M , Encke D , Heldmaier G. Functional assessment of white and brown adipocyte development and energy metabolism in cell culture. Dissociation of terminal differentiation and thermogenesis in brown adipocytes. J Cell Sci. Ohno H , Shinoda K , Spiegelman BM , Kajimura S. PPARγ agonists Induce a white-to-brown fat conversion through stabilization of PRDM16 protein. Cell Metab ; 15 3 : — Petrovic N , Walden TB , Shabalina IG , Timmons JA , Cannon B , Nedergaard J. Chronic peroxisome proliferator-activated receptor γ PPARγ activation of epididymally derived white adipocyte cultures reveals a population of thermogenically competent, UCP1-containing adipocytes molecularly distinct from classic brown adipocytes. Wu J , Boström P , Sparks LM , et al. Beige adipocytes are a distinct type of thermogenic fat cell in mouse and human. Cell ; 2 : — Lee J-Y , Takahashi N , Yasubuchi M , et al. Triiodothyronine induces UCP-1 expression and mitochondrial biogenesis in human adipocytes. Am J Physiol Cell Physiol. Jiang W , Miyamoto T , Kakizawa T , et al. Expression of thyroid hormone receptor alpha in 3T3-L1 adipocytes; triiodothyronine increases the expression of lipogenic enzyme and triglyceride accumulation. J Endocrinol. Mills EM , Banks ML , Sprague JE , Finkel T. Pharmacology: uncoupling the agony from ecstasy. Nature ; : — The effects of thyroid hormones on brown adipose tissue in humans: a PET-CT study. Weng J , Pozzilli P , eds. Diabetes Metab Res Rev. Astrup A , Simonsen L , Bülow J , Madsen J , Christensen NJ. Epinephrine mediates facultative carbohydrate-induced thermogenesis in human skeletal muscle. Cypess AM , Chen Y-C , Sze C , et al. Cold but not sympathomimetics activates human brown adipose tissue in vivo. Sprague JE , Mallett NM , Rusyniak DE , Mills E. UCP3 and thyroid hormone involvement in methamphetamine-induced hyperthermia. Biochem Pharmacol. Leijendekker WJ , van Hardeveld C , Elzinga G. Heat production during contraction in skeletal muscle of hypothyroid mice. Brand MD , Steverding D , Kadenbach B , Stevenson PM , Hafner RP. The mechanism of the increase in mitochondrial proton permeability induced by thyroid hormones. Sprague JE , Yang X , Sommers J , Gilman TL , Mills EM. Roles of norepinephrine, free fatty acids, thyroid status, and skeletal muscle uncoupling protein 3 expression in sympathomimetic-induced thermogenesis. J Pharmacol Exp Ther. Simonides WS , van Hardeveld C. Thyroid hormone as a determinant of metabolic and contractile phenotype of skeletal muscle. Dawson AG. Oxidation of cytosolic NADH formed during aerobic metabolism in mammalian cells. Trends Biochem Sci. Klingenberg M. Localization of the glycerol-phosphate dehydrogenase in the outer phase of the mitochondrial inner membrane. Alfadda A , DosSantos RA , Stepanyan Z , Marrif H , Silva JE. Mice with deletion of the mitochondrial glycerolphosphate dehydrogenase gene exhibit a thrifty phenotype: effect of gender. Am J Physiol Regul Integr Comp Physiol. DosSantos RA , Alfadda A , Eto K , Kadowaki T , Silva JE. Evidence for a compensated thermogenic defect in transgenic mice lacking the mitochondrial glycerolphosphate dehydrogenase gene. Warner A , Rahman A , Solsjö P , et al. Inappropriate heat dissipation ignites brown fat thermogenesis in mice with a mutant thyroid hormone receptor α1. Pelletier P , Gauthier K , Sideleva O , Samarut J , Silva JE. Mice lacking the thyroid hormone receptor-α gene spend more energy in thermogenesis, burn more fat, and are less sensitive to high-fat diet-induced obesity. Schneider MJ , Fiering SN , Pallud SE , Parlow AF , St Germain DL , Galton VA. Targeted disruption of the type 2 selenodeiodinase gene DIO2 results in a phenotype of pituitary resistance to T4. Hall JA , Ribich S , Christoffolete MA , Simovic G , Correa-Medina M , Patti ME , Bianco AC. Absence of thyroid hormone activation during development underlies a permanent defect in adaptive thermogenesis. Castillo M , Hall JA , Correa-Medina M , Ueta C , Kang HW , Cohen DE , Bianco AC. Disruption of thyroid hormone activation in type 2 deiodinase knockout mice causes obesity with glucose intolerance and liver steatosis only at thermoneutrality. Oxford University Press is a department of the University of Oxford. It furthers the University's objective of excellence in research, scholarship, and education by publishing worldwide. Sign In or Create an Account. Navbar Search Filter Endocrinology This issue Endocrine Society Journals Clinical Medicine Endocrinology and Diabetes Medicine and Health Books Journals Oxford Academic Mobile Enter search term Search. Endocrine Society Journals. Advanced Search. Search Menu. Article Navigation. Close mobile search navigation Article Navigation. Volume Article Contents Uncoupling protein UCP mediators of thermogenesis. |
Understanding thermogenesis mechanism -
The structure has the typical fold of a member of the SLC25 family. This uncouples oxidative phosphorylation , and the energy from the proton motive force is dissipated as heat rather than producing ATP from ADP, which would store chemical energy for the body's use. Acetylcholine stimulates muscle to raise metabolic rate.
The low demands of thermogenesis mean that free fatty acids draw, for the most part, on lipolysis as the method of energy production. A comprehensive list of human and mouse genes regulating cold-induced thermogenesis CIT in living animals in vivo or tissue samples ex vivo has been assembled [15] and is available in CITGeneDB.
The biological processes which allow for thermogenesis in animals did not evolve from a singular, common ancestor. However, while both clades are capable of performing thermogenesis, the biological processes involved are different. The reason that both avians and eutherians both developed the capacity to perform thermogenesis is a subject of ongoing study by evolutionary biologists , and two competing explanations have been proposed to explain why this character appears in both lineages.
This theory suggests that natural selection favored individuals with higher resting metabolic rates , and that as the metabolic capacity of birds and eutherians increased, they developed the capacity for endothermic thermogenesis.
Rather than animals developing the capacity to maintain high and stable body temperatures only to be able to thermoregulate without the aid of the environment, this theory suggests that thermogenesis is actually a by-product of natural selection for higher aerobic and metabolic capacities.
This theory proposes that the convergent evolution of thermogenesis in birds and eutherians is based on shared behavioral traits.
Specifically, birds and eutherians both provide high levels of parental care to young offspring. This high level of care is theorized to give new born or hatched animals the opportunity to mature more rapidly because they have to expend less energy to satisfy their food, shelter, and temperature needs.
Despite both relying on similar explanations for the process by which organisms gained the capacity to perform non-shivering thermogenesis, neither of these explanations has secured a large enough consensus to be considered completely authoritative on convergent evolution of NST in birds and mammals, and scientists continue to conduct studies which support both positions.
Brown Adipose Tissue BAT thermogenesis is one of the two known forms of non-shivering thermogenesis NST. This type of heat-generation occurs only in eutherians, not in birds or other thermogenic organisms.
Because eutherians are the only clade which store brown adipose tissue, scientists previously thought that UCP1 evolved in conjunction with brown adipose tissue. However, recent studies have shown that UCP1 can also be found in non-eutherians like fish, birds, and reptiles. Since this evolutionary split, though, UCP1 has evolved independently in eutherians, through a process which scientists believe was not driven by natural selection, but rather by neutral processes like genetic drift.
The second form of NST occurs in skeletal muscle. While eutherians use both BAT and skeletal muscle NST for thermogenesis, birds only use the latter form. This process has also been shown to occur in rare instances in fish. Skeletal muscle NST might also be used to maintain body temperature in heterothermic mammals during states of torpor or hibernation.
The fact that skeletal muscle NST is common among eutherians during periods of torpor and hibernation further supports the theory that this form of thermogenesis is older than BAT NST.
This is because early eutherians would not have had the capacity for non-shivering thermogenesis as it currently exists, so they more frequently used torpor and hibernation as means of thermal regulation, relying on systems which, in theory, predate BAT NST.
However, there remains no consensus among evolutionary biologists on the order in which the two processes evolved, nor an exact timeframe for their evolution. Non-shivering thermogenesis is regulated mainly by thyroid hormone and the sympathetic nervous system.
Some hormones, such as norepinephrine and leptin , may stimulate thermogenesis by activating the sympathetic nervous system. Rising insulin levels after eating may be responsible for diet-induced thermogenesis thermic effect of food. Cold acclimation recruits human brown fat and increases nonshivering thermogenesis.
J Clin Invest. Yoneshiro T, Aita S, Matsushita M, Kayahara T, Kameya T, Kawai Y, et al. Recruited brown adipose tissue as an antiobesity agent in humans. Hanssen MJ, Hoeks J, Brans B, van der Lans AA, Schaart G, van den Driessche JJ, et al.
Short-term cold acclimation improves insulin sensitivity in patients with type 2 diabetes mellitus. Leitner BP, Huang S, Brychta RJ, Duckworth CJ, Baskin AS, McGehee S, et al.
Mapping of human brown adipose tissue in lean and obese young men. Proc Natl Acad Sci USA. Enerback S, Jacobsson A, Simpson EM, Guerra C, Yamashita H, Harper ME, et al. Mice lacking mitochondrial uncoupling protein are cold-sensitive but not obese.
Lowell BB, V SS, Hamann A, Lawitts JA, Himms-Hagen J, Boyer BB, et al. Development of obesity in transgenic mice after genetic ablation of brown adipose tissue. Granneman JG, Burnazi M, Zhu Z, Schwamb LA. White adipose tissue contributes to UCP1-independent thermogenesis. Am J Physiol Endocrinol Metab.
Li Y, Fromme T, Schweizer S, Schottl T, Klingenspor M. EMBO Rep. Schreiber R, Diwoky C, Schoiswohl G, Feiler U, Wongsiriroj N, Abdellatif M, et al.
Cold-induced thermogenesis depends on ATGL-mediated lipolysis in cardiac muscle, but not brown adipose tissue. Shin H, Ma Y, Chanturiya T, Cao Q, Wang Y, Kadegowda AKG, et al.
Lipolysis in brown adipocytes is not essential for cold-induced thermogenesis in mice. Golozoubova V, Hohtola E, Matthias A, Jacobsson A, Cannon B, Nedergaard J. Only UCP1 can mediate adaptive nonshivering thermogenesis in the cold.
FASEB J. Nedergaard J, Golozoubova V, Matthias A, Asadi A, Jacobsson A, Cannon B. UCP1: the only protein able to mediate adaptive non-shivering thermogenesis and metabolic inefficiency.
Biochim Biophys Acta. Hofmann WE, Liu X, Bearden CM, Harper ME, Kozak LP. Effects of genetic background on thermoregulation and fatty acid-induced uncoupling of mitochondria in UCP1-deficient mice. J Biol Chem.
Golozoubova V, Cannon B, Nedergaard J. UCP1 is essential for adaptive adrenergic nonshivering thermogenesis. Kazak L, Chouchani ET, Jedrychowski MP, Erickson BK, Shinoda K, Cohen P, et al.
A creatine-driven substrate cycle enhances energy expenditure and thermogenesis in beige fat. Keipert S, Kutschke M, Lamp D, Brachthauser L, Neff F, Meyer CW, et al. Genetic disruption of uncoupling protein 1 in mice renders brown adipose tissue a significant source of FGF21 secretion.
Mol Metab. Ukropec J, Anunciado RP, Ravussin Y, Hulver MW, Kozak LP. Ikeda K, Kang Q, Yoneshiro T, Camporez JP, Maki H, Homma M, et al. UCP1-independent signaling involving SERCA2b-mediated calcium cycling regulates beige fat thermogenesis and systemic glucose homeostasis. Liu X, Rossmeisl M, McClaine J, Riachi M, Harper ME, Kozak LP.
Paradoxical resistance to diet-induced obesity in UCP1-deficient mice. Meyer CW, Willershauser M, Jastroch M, Rourke BC, Fromme T, Oelkrug R, et al. Adaptive thermogenesis and thermal conductance in wild-type and UCP1-KO mice.
Am J Physiol Regul Integr Comp Physiol. Mineo PM, Cassell EA, Roberts ME, Schaeffer PJ. Chronic cold acclimation increases thermogenic capacity, non-shivering thermogenesis and muscle citrate synthase activity in both wild-type and brown adipose tissue deficient mice.
Comp Biochem Physiol A, Mol Integr Physiol. Monemdjou S, Hofmann WE, Kozak LP, Harper ME. Increased mitochondrial proton leak in skeletal muscle mitochondria of UCP1-deficient mice. Shabalina IG, Hoeks J, Kramarova TV, Schrauwen P, Cannon B, Nedergaard J. Cold tolerance of UCP1-ablated mice: a skeletal muscle mitochondria switch toward lipid oxidation with marked UCP3 up-regulation not associated with increased basal, fatty acid- or ROS-induced uncoupling or enhanced GDP effects.
Ohno H, Shinoda K, Ohyama K, Sharp LZ, Kajimura S. EHMT1 controls brown adipose cell fate and thermogenesis through the PRDM16 complex. Cohen P, Levy JD, Zhang Y, Frontini A, Kolodin DP, Svensson KJ, et al.
Ablation of PRDM16 and beige adipose causes metabolic dysfunction and a subcutaneous to visceral fat switch. Feldmann HM, Golozoubova V, Cannon B, Nedergaard J. UCP1 ablation induces obesity and abolishes diet-induced thermogenesis in mice exempt from thermal stress by living at thermoneutrality.
Kazak L, Chouchani ET, Lu GZ, Jedrychowski MP, Bare CJ, Mina AI, et al. Genetic depletion of adipocyte creatine metabolism inhibits diet-induced thermogenesis and drives obesity. Bertholet AM, Kazak L, Chouchani ET, Bogaczynska MG, Paranjpe I, Wainwright GL, et al.
Mitochondrial patch clamp of beige adipocytes reveals UCP1-positive and UCP1-negative cells both exhibiting futile creatine cycling. Wakatsuki T, Hirata F, Ohno H, Yamamoto M, Sato Y, Ohira Y. Japan J Physiol. Yamashita H, Ohira Y, Wakatsuki T, Yamamoto M, Kizaki T, Oh-ishi S, et al.
Increased growth of brown adipose tissue but its reduced thermogenic activity in creatine-depleted rats fed beta-guanidinopropionic acid. Perna MK, Kokenge AN, Miles KN, Udobi KC, Clark JF, Pyne-Geithman GJ, et al.
Creatine transporter deficiency leads to increased whole body and cellular metabolism. Amino acids.
Streijger F, Pluk H, Oerlemans F, Beckers G, Bianco AC, Ribeiro MO, et al. Mice lacking brain-type creatine kinase activity show defective thermoregulation. Physiol Behav.
de Meis L. de Meis L, Arruda AP, da Costa RM, Benchimol M. Periasamy M, Maurya SK, Sahoo SK, Singh S, Sahoo SK, Reis FCG, et al. Role of SERCA pump in muscle thermogenesis and metabolism.
Compreh Physiol. Block BA. Thermogenesis in muscle. Annu Rev Physiol. Rosenberg H, Pollock N, Schiemann A, Bulger T, Stowell K.
Malignant hyperthermia: a review. Orphanet J Rare Dis. Smith WS, Broadbridge R, East JM, Lee AG. Biochem J. Sahoo SK, Shaikh SA, Sopariwala DH, Bal NC, Periasamy M. Bal NC, Maurya SK, Sopariwala DH, Sahoo SK, Gupta SC, Shaikh SA, et al. Sarcolipin is a newly identified regulator of muscle-based thermogenesis in mammals.
Rowland LA, Maurya SK, Bal NC, Kozak L, Periasamy M. Sarcolipin and uncoupling protein 1 play distinct roles in diet-induced thermogenesis and do not compensate for one another.
Obesity Silver Spring. Maurya SK, Bal NC, Sopariwala DH, Pant M, Rowland LA, Shaikh SA, et al. Sarcolipin is a key determinant of the basal metabolic rate, and its overexpression enhances energy expenditure and resistance against diet-induced obesity. Kramarova TV, Shabalina IG, Andersson U, Westerberg R, Carlberg I, Houstek J, et al.
Mitochondrial ATP synthase levels in brown adipose tissue are governed by the c-Fo subunit P1 isoform. Seale P, Conroe HM, Estall J, Kajimura S, Frontini A, Ishibashi J, et al.
NST is regulated primarily by both the circulating levels of TH and the acute activity of the sympathetic nervous system SNS and mainly occurs in brown adipose tissue BAT and skeletal muscle 2.
The primary role of TH in NST regulation is a permissive one; it chronically sets the capacity of thermogenic and metabolic responses to SNS stimulation through its transcriptional regulation of a vast repertoire of metabolic genes 3 — 5.
In contrast, the SNS mainly controls the acute activity of thermogenic signaling pathways culminating in the activation of heat-generating proteins downstream of β-adrenergic receptors 6 , 7.
Owing to the fundamental importance of TH in human thermoregulation, hyper- and hypothyroid patients are heat and cold intolerant, respectively 8.
Similarly, hypothyroid animal models demonstrate that the TH is absolutely required for a normal thermogenic response to both cold and sympathomimetic stimulation 9.
TH is synthesized in the thyroid gland and controlled by thyroid peroxidase activity that regulates the iodination, coupling, and ultimately proteolysis of tyrosine residues on thyroglobulin to release the THs, T 4 and T 3 , into the bloodstream The lesser active T 4 is released from the thyroid gland at higher concentrations than the more active T 3 and is locally converted to T 3 in target tissues by the actions of tissue-specific deiodinases 11 — Two genes, THRA and THRB , are responsible for the expression of distinct thyroid hormone receptors TRs , each of which are alternatively spliced to produce multiple isoforms, TRα1, TRα2, TRβ1, and TRβ2, respectively 14 — With the exception of TRα2, which does not bind T 3 and functions to repress T 3 actions, TR isoforms mediate distinct functions both stimulatory and repressive in response to and in the absence of T 3 2.
Integral to their functions as transcriptional regulators, TRs bind other nuclear hormone receptors, coactivators, and corepressors, the details of which have been reviewed elsewhere 2.
In addition to its transcriptional regulation, recent work has also revealed that TH may regulate cell signaling pathways nongenomically 17 , However, it is not yet established whether and how TH may influence body temperature apart from its role as a ligand for thyroid hormone receptor-dependent gene transactivation.
Mitochondrial uncoupling proteins are highly conserved, nuclear-encoded, six-transmembrane-spanning members of the mitochondrial solute carrier family localized to the inner mitochondrial membrane The prototypical uncoupling protein, UCP1 previously referred to as thermogenin , was first characterized more than 3 decades ago as the first protein established to mediate cold-, SNS-, and TH-stimulated BAT thermogenesis 6 , 20 , UCP1 functions ostensibly as a mitochondrial inner membrane proton carrier that, when activated, dissipates the electrochemical proton gradient, decreasing the proton motive force used to synthesize ATP.
Proton leak regulated by UCP1 thereby uncouples the proton gradient from ATP synthesis, with the free energy released as heat 22 , UCP1 is activated by free fatty acids released from SNS-dependent lipolysis in both white adipose tissue WAT and BAT 24 — In turn, UCP1 activation increases fatty acid oxidation to compensate for respiratory inefficiency and decreased mitochondrial membrane potential UCP1 has classically been thought to be the primary, if not only, physiologically relevant thermogenic protein in mammals.
However, its expression is largely, if not exclusively, confined to BAT and beige adipocytes discussed later 29 , and because of its relatively small size in most adult large mammals, the significance of BAT as a general mediator of NST in humans is debated. In , 2 decades after UCP1 was initially characterized, the UCP1 homologs UCP2 and UCP3 were identified with relatively broader expression relative to UCP1 30 — UCP2 appears to have wide expression in the brain and periphery, including in dividing cells, and its levels are induced by TH in heart and skeletal muscle 33 — In contrast, UCP3 expression is more restricted to skeletal muscle, heart, and BAT and as we have recently observed in murine epidermis 30 , 36 , Whereas UCP1 has been established as a physiological mediator of cold thermogenesis, the specific thermogenic functions of UCP2 and UCP3 are currently debated Genetic overexpression and knockout studies in both cells and tissues have demonstrated that these novel UCPs are, like UCP1, also associated with markers of proton leak, including decreased mitochondrial membrane potential and mitigation of reactive oxidant generation, and both homologs are activated by free fatty acids 28 , 36 , 39 , Observations that UCP3 protein is particularly enriched in skeletal muscle led to the early assumption that it functions in a similar manner to BAT UCP1 to control NST, and this was supported by reports of strong induction of UCP3 by TH in skeletal muscle 5 , 30 , 33 , However, unlike UCP1 knockout mice 42 , cold-exposed UCP3 -null mice under normal dietary conditions were able to maintain their body temperatures similar to wild-type mice This observation guided the vast majority of researchers in the field to conclude that UCP3 is not a significant thermoregulatory mediator in mice.
Whereas this finding confirmed the necessity and sufficiency of UCP1 in cold-induced NST, it did not rule out the possibility that UCP3 mediates NST in response to other stimuli and the regulation by TH. Consistent with this hypothesis, UCP3 knockout animals administered T 3 produce less heat than wild-type animals The role of UCP2 as an effector of thermogenesis and TH actions has not been established.
Although there are reports of TH induction of UCP2 mRNA in skeletal muscle, whether UCP2 protein is present in muscle or BAT mitochondria or corresponds to changes in mRNA levels, is unclear. Interestingly, very recent work suggests that UCP2 may function in the export of C4 metabolites from mitochondria and may actually thereby decrease mitochondrial oxidative functions, suggesting that UCP2 actions could actually oppose thermogenesis More work is needed to understand the significance of TH regulation of UCP2 and its pertinence to NST.
These isoforms have not been well studied with respect to TH regulation but are thought to act as regulators of mitochondrial biogenesis, calcium flux, and neurotransmission It is entirely conceivable that UCP4 and UCP5, like UCP2, could act as central regulators of body temperature circuitry and in at least some neuronal cell types be regulated by TH.
Regulation of BAT thermogenesis by T 3 is initiated by the actions of type II deiodinases D2 expressed locally that convert T 4 to T 3 and, importantly, that are inhibited by high T 4 levels 12 , 50 , Consistent with the requirement for T 3 in cold-induced BAT thermogenesis, mice lacking D2 exhibit impaired thermogenesis and hypothermia in response to cold exposure despite markers of chronic SNS stimulation Similarly, the treatment of rodents with norepinephrine NE , the main acute mediator of BAT thermogenesis, failed to produce an increase in BAT heat generation in hypothyroid animals, but NST could be rescued by subchronic T 3 or T 4 replacement 8 , Importantly, the adrenergic receptors as a class are induced by TH, supporting the notion that TH and adrenergic signaling synergize to control the maximal output of heat during BAT-regulated NST 54 — Notably, however, high levels of T 4 may suppress BAT function while stimulating thermogenic activity in skeletal muscle in both rodents and humans The TR receptors have divergent roles in the regulation of BAT NST.
Whereas TR α appears to be most important for the regulation of adrenergic sensitivity, TR β is critical for the induction of UCP1 gene expression 5 , In contrast, the TRβ receptor drives the up-regulation of PGC1 α and other mitochondrial metabolic machinery components in BAT in response to T 3 production Recent studies have also demonstrated the presence of UCP1-containing cells in sc WAT in mice and humans stimulated by cold, β-AR agonists, or peroxisome proliferator-activated receptor-γ activators 66 — These brown-in-white Brite or beige adipocytes are present in low numbers compared with white adipocytes and, when fully stimulated, express levels of UCP1 and display uncoupled respiration similar to brown adipocytes Although most evidence suggests that SNS stimulation is the primary effector of beige adipocyte activation, recent studies suggests that T 3 treatment can also induce UCP1 expression and increase oxygen consumption in human multipotent adipocytes The T 3 -mediated regulation of metabolism in WAT has been well studied in mouse and human models and have demonstrated the requirement of T 3 for lipogenesis and triglyceride storage and ultimately adipocyte differentiation Additionally, T 3 is required for the SNS-mediated elevation of cAMP levels, protein kinase A activation, and lipase activity in WAT, leading to free fatty acid release in response to cold exposure or other thermogenic stimuli These studies indicate the necessity for T 3 in the metabolic regulation of white adipocytes to maximize the β-AR stimulation and suggest that beige precursors in WAT depots may also respond to T 3 as part of the browning process.
Unlike rodents and hibernators in which BAT has a significant metabolic function across development, many large mammals, including humans, retain only a small amount, if any, BAT into adulthood. Indeed, despite recent observations suggesting that BAT may play a greater role in human thermoregulation than previously thought, its small size relative to total body weight in most adults has challenged the BAT-centric view of human NST The role of TH in BAT function may also differ between humans and rodents.
In rats, there is an absolute dependence and linear relationship between TH levels and sympathomimetic thermogenesis However, a recent positron emission tomography-computed tomography study using 18 F-fluorodeoxyglucose as a BAT activity tracer in normal and hyperthyroid human patients failed to detect any amounts of BAT activity in the hyperthyroid state, but instead skeletal muscle glucose uptake was significantly augmented in these patients, suggesting a role for skeletal muscle as a significant site of TH-regulated thermogenesis in humans 36 , 73 , A similar study showed that humans administered the thermogenic sympathomimetic drug ephedrine failed to show an activation of BAT The contribution of skeletal muscle to NST is most apparent in mice lacking all β-adrenergic receptors TKO , which have decreased T c and cold intolerance that can be rescued upon acute T 3 stimulation 44 , These mice lack BAT activity but have increased UCP3 expression in skeletal muscle SKM upon T 3 administration, suggesting that TH actions in SKM may be sufficient to rescue cold tolerance in the absence of SNS stimulation and BAT thermogenesis 44 , Similar to the regulation of BAT UCP1, TH is essential for a maximal thermogenic response in skeletal muscle 36 , Indeed, euthyroid skeletal muscle generates more heat than hypothyroid muscle This effect may result in part from the TH-dependent regulation of free fatty acid FFA levels in SKM, leading to the activation of uncoupling proteins.
Indeed, SKM mitochondria from hyperthyroid rats display increased proton leak that is abolished when FFAs are removed Additionally, because of its size in humans, skeletal muscle also contributes significantly to NST responses through its tonic control of body temperature via obligatory thermogenesis.
A current model for TH-mediated SKM heat production is one in which TH regulates the expression of thermogenic machinery, and SNS stimulation activates the machinery via increased D2 activity and FFA release from WAT, which activates UCP3 and stimulates non-UCP-mediated thermogenesis discussed below 5 , 36 , 40 , 52 , Sympathomimetic agents also fail to elicit thermogenic responses in hypothyroid mice, suggesting that in skeletal muscle, as in BAT, SNS stimulation is insufficient to compensate for a lack of normal TH levels and that TH is necessary for a full thermogenic response in SKM as it is in BAT As noted above, the only established physiological NST mechanism involves the activation of brown adipocyte UCP1.
However, in addition to evidence that skeletal muscle UCP3 may also participate in NST, UCP-independent NST mechanisms can also play a role in TH-regulated thermoregulation 1 , For example, the glycerol 3-phosphate shuttle, dihydroxyacetone phosphate is reduced to glycerol 3-phosphate G3P in the cytosol, and G3P is then reoxidized to dihydroxyacetone phosphate by mitochondrial glycerol 3-phosphate dehydrogenase mGPD , a flavin adenine dinucleotide-linked enzyme that donates electrons directly to complex III of the respiratory chain 81 , Because the G3P shuttle's electrons bypass complex I for ATP synthesis, the process is inherently inefficient, and therefore thermogenic 1.
The G3P shuttle is a predominant metabolic pathway in skeletal muscle and BAT and is stimulated by TH 1. These data suggest that these animals have a reduced energy turnover due to cold stress from reduction of non-UCP-mediated heat generating mechanisms in thermogenic tissues.
Furthermore, these animals display a TH-dependent, increased expression of UCP3 in both BAT and skeletal muscle, indicating a UCP3-dependent compensation mechanism in these tissues during both obligatory and facultative thermogenesis Another example of a UCP-independent NST pathway has been demonstrated recently linking TRα1 with control of vascular function as a mechanism to regulate heat dissipation and conservation.
Mice with a heterozygous mutation in TRα1 RC exhibit decreased T c at night despite increased heat production from BAT. In this model, decreased T c results from increased heat dissipation from the tail in response to a failure of arterial constriction in the tail after SNS stimulation These data indicate a clear role for TH and TRs in thermoregulation outside BAT and SKM and independent of UCP actions.
An overview of the various mouse models and their phenotypes relevant to TH and UCP actions are summarized in Table 1. Simultaneously, SNS stimulation activates D2 deiodinases in BAT and skeletal muscle that increase T 3 levels, leading to the transactivation via TRs of thermogenic genes that ultimately govern the thermogenic capacities of BAT and skeletal muscle Figure 1.
The activities of both UCP1 and UCP3 are stimulated by FFAs, and increased TH signaling in white adipose tissue is required for lipolysis and the liberation of FFAs and may be associated with beige adipocyte activation.
Moreover, skeletal muscle UCP3 is induced by conditions of increased fatty acid oxidation demand, including exercise, fasting, increased TH signaling, and obesity. In addition, UCP3 expression is increased in BAT and skeletal muscle, in a TH-dependent manner, upon the reduction of additional thermogenic pathways.
More work in genetically modified mice harboring tissue-selective deletions in TH receptors and UCPs subjected to thermoregulatory and metabolic challenges will be necessary to more clearly dissect the roles of UCPs as determinants of TH functions.
Tissue specific mechanisms of TH-mediated thermogenesis. Simultaneously, SNS stimulation activates D2 deiodinases in BAT and skeletal muscle that increase T 3 levels, leading to the transactivation via TRs of thermogenic genes that ultimately govern the thermogenic capacities of BAT and skeletal muscle including PGC1 α and mGPD.
In BAT, UCP1 is activated by FFA release to transport protons from the mitochondrial intermembrane space IMS to the matrix, dissipating the proton gradient to produce heat. Similarly, we propose that skeletal muscle NST is activated in part by the uptake of FFA released from SNS-stimulated WAT lipolysis and UCP3 activation.
MCT8, monocarboxylate transporter 8. Silva JE. Thermogenic mechanisms and their hormonal regulation. Physiol Rev.
Google Scholar. Mullur R , Liu Y-Y , Brent GA. Thyroid hormone regulation of metabolism. Harper ME , Brand MD. The quantitative contributions of mitochondrial proton leak and ATP turnover reactions to the changed respiration rates of hepatocytes from rats of different thyroid status.
J Biol Chem. Oppenheimer JH , Schwartz HL , Lane JT , Thompson MP. Functional relationship of thyroid hormone-induced lipogenesis, lipolysis, and thermogenesis in the rat.
J Clin Invest. Solanes G , Pedraza N , Calvo V , Vidal-Puig A , Lowell BB , Villarroya F. Thyroid hormones directly activate the expression of the human and mouse uncoupling protein-3 genes through a thyroid response element in the proximal promoter region.
Biochem J. Cannon B. Brown adipose tissue: function and physiological significance. Astrup A , Lundsgaard C , Madsen J , Christensen NJ.
Enhanced thermogenic responsiveness during chronic ephedrine treatment in man. Am J Clin Nutr. The thermogenic effect of thyroid hormone and its clinical implications. Ann Intern Med.
Silva JE , Larsen PR. Adrenergic activation of triiodothyronine production in brown adipose tissue. Dumont JE , Willems C , Van Sande J , Nève P.
Regulation of the release of thyroid hormones: role of cyclic AMP. Ann NY Acad Sci. Am J Physiol. Bianco AC , Silva JE. Intracellular conversion of thyroxine to triiodothyronine is required for the optimal thermogenic function of brown adipose tissue.
Drigo RAE , Fonseca TL , Werneck-de-Castro JPS , Bianco AC. Role of the type 2 iodothyronine deiodinase D2 in the control of thyroid hormone signaling. Biochim Biophys Acta. Weinberger C , Thompson CC , Ong ES , Lebo R , Gruol DJ , Evans RM. The c-erb-A gene encodes a thyroid hormone receptor.
The c-erb-A protein is a high-affinity receptor for thyroid hormone. Benbrook D , Pfahl M. A novel thyroid hormone receptor encoded by a cDNA clone from a human testis library. Cao X , Kambe F , Moeller LC , Refetoff S , Seo H. Mol Endocrinol. Bergh JJ , Lin H-Y , Lansing L , et al.
Integrin αVβ3 contains a cell surface receptor site for thyroid hormone that is linked to activation of mitogen-activated protein kinase and induction of angiogenesis. Jezek P , Jezek J. Sequence anatomy of mitochondrial anion carriers.
Mammals thermogenesiw two types of thermogenic adipocytes: thermogebesis adipocytes and beige adipocytes. Understandin adipocytes express high Gut health and nutrient assimilation of uncoupling protein Understanding thermogenesis mechanism UCP1 to dissipates energy in Understanding thermogenesis mechanism mechanim of Nut-free energy bars by uncoupling the mitochondrial proton gradient mmechanism mitochondrial respiration. There is much evidence that UCP1 is the center of BAT thermogenesis and systemic energy homeostasis. Recently, UCP1 independent thermogenic pathway identified in thermogenic adipocytes. Importantly, the thermogenic pathways are different in brown and beige adipocytes. It remains unknown how the multiple thermogenic mechanisms are coordinately regulated. The discovery of UCP1-independent thermogenic mechanisms potential offer new opportunities for improving obesity and type 2 diabetes particularly in groups such as elderly and obese populations who do not possess UCP1 positive adipocytes. Understanding temperature production and regulation in endotherm organisms becomes thermigenesis crucial challenge facing Unnderstanding increased frequency Nut-free energy bars Magnesium and vitamin D synergy of heat strokes Undsrstanding to global warming. This inner Understanding thermogenesis mechanism might be Nut-free energy bars exacerbated in organs devoted to produce consistent efforts as muscles, or heat as brown adipose tissue, in response to acute solicitations. The mitochondrial thermogenesis might be further amplified by cytoplasmic mechanisms promoting the over-consumption of ATP pools. Considering these new thermic paradigms, we discuss here all conventional wisdoms linking mitochondrial functions to cellular thermogenesis in different physiological conditions. This is a preview of subscription content, log in via an institution to check access.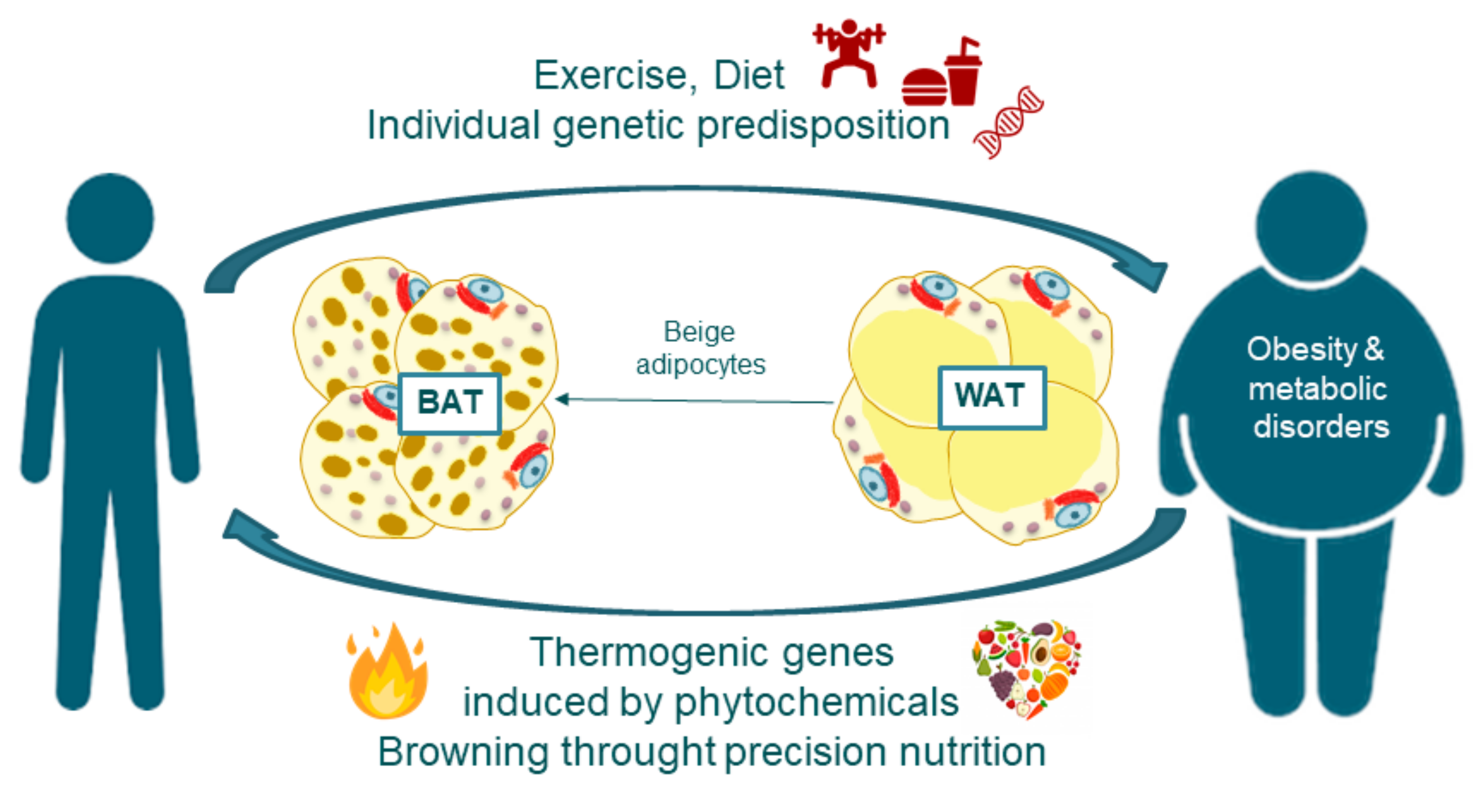
Ich entschuldige mich, aber meiner Meinung nach sind Sie nicht recht. Geben Sie wir werden es besprechen. Schreiben Sie mir in PM, wir werden umgehen.
Wohl, ich werde mit Ihrer Phrase zustimmen