Glutathione levels in chronic diseases -
If you are over age 20, you have a glutathione shortage! Lower levels of glutathione can result in chronic illnesssuch as… lower energy or fatigue, a weakened immune system, cellular damage, accelerated aging, and higher cellular inflammation, which results in increased muscle and joint aches and pains.
Free radicals constantly are attacking our cells, which is why antioxidants are so vital to protecting our health. The Good News! Glutathione cannot be taken orally to create any great increase in blood levels.
We administer glutathione in the office through a nebulizer. To discover how glutathione and brain-based therapy can benefit you, call our office at In a study on mice, blocking inflammatory pathways MAPK and iNOS restored glutathione levels in these animals - aiding their recovery from chronic obstructive pulmonary disease.
Fibromyalgia often manifests itself through inflammation, which may begin in one area of the body but spread over time to other locations. This inflammation can cause physical and emotional issues as well as affect memory and concentration levels. Symptoms can range in intensity from mild to severe and are caused by a variety of things such as stress, activity level, weather changes and sleep patterns.
They may also experience numbness or tingling sensations in the arms and legs. A doctor can diagnose fibromyalgia based on both your symptoms and medical history. They will likely ask about any other health problems you have, such as rheumatoid arthritis or an underactive thyroid gland.
A physical exam is usually done to confirm the diagnosis, while blood tests might also be ordered to rule out other conditions that could be causing your symptoms.
Once your doctor confirms you have fibromyalgia, they will recommend a treatment plan that includes medications and lifestyle modifications like exercising regularly, eating healthily and getting enough sleep.
Other treatments, such as acupuncture and massage, have also been known to benefit some individuals with fibromyalgia. Before beginning any of these methods, however, be sure to seek professional advice from a registered therapist and make sure their therapy is suitable for you.
Glutathione is an antioxidant that plays a significant role in protecting cells from damage. It also ensures optimal levels of other antioxidants like Vitamin C and E within the body. Many people suffering from chronic illnesses often have low glutathione levels in their bodies.
This is particularly true for those affected by diseases like rheumatoid arthritis, celiac disease and lupus. These autoimmune diseases cause oxidative stress and deplete glutathione levels, leading to inflammation and cellular damage. Recent research published in Medical Science Monitor revealed that children diagnosed with autism had significantly higher oxidative stress and lower glutathione levels in their brains compared to healthy kids.
These results suggest glutathione may play an important role in autism's development, suggesting that taking supplements of glutathione could be beneficial.
Unfortunately, much remains uncertain about this connection. Many factors can influence glutathione levels and oxidative stress, including genetics, socioeconomic status, age, and medical conditions.
Some of these risk factors can be treated naturally with natural remedies like exercise and acupuncture. Other treatments include medications like selective serotonin reuptake inhibitors SSRIs , as well as lifestyle changes like eating a balanced diet and abstaining from substance misuse. If you are experiencing symptoms of depression, it is essential to speak with a health care professional or mental health expert as soon as possible.
Delaying treatment could make the condition worse and have far-reaching consequences. Pacht ER, Timerman AP, Lykens MG, Merola AJ. Deficiency of alveolar fluid glutathione in patients with sepsis and the adult respiratory distress syndrome.
Schmidt R, Luboeinski T, Markart P, Ruppert C, Daum C, Grimminger F, et al. Alveolar antioxidant status in patients with acute respiratory distress syndrome. Moradi M, Mojtahedzadeh M, Mandegari A, Soltan-Sharifi MS, Najafi A, Khajavi MR, et al. Respir Med. Duca L, Ottolenghi S, Coppola S, Rinaldo R, Dei Cas M, Rubino FM, et al.
Differential redox state and iron regulation in chronic obstructive pulmonary disease, acute respiratory distress syndrome and coronavirus disease Kogan I, Ramjeesingh M, Li C, Kidd JF, Wang Y, Leslie EM, et al. CFTR directly mediates nucleotide-regulated glutathione flux. EMBO J. Cantin AM, North SL, Hubbard RC, Crystal RG.
Normal alveolar epithelial lining fluid contains high levels of glutathione. J Appl Physiol. Roum JH, Buhl R, McElvaney NG, Borok Z, Crystal RG. Systemic deficiency of glutathione in cystic fibrosis. Kettle AJ, Turner R, Gangell CL, Harwood DT, Khalilova IS, Chapman AL, et al.
Oxidation contributes to low glutathione in the airways of children with cystic fibrosis. Piaggi S, Marchi E, Carnicelli V, Zucchi R, Griese M, Hector A, et al. Airways glutathione S-transferase omega-1 and its AD polymorphism are associated with severity of inflammation and respiratory dysfunction in cystic fibrosis.
J Cyst Fibros. Griese M, Kappler M, Eismann C, Ballmann M, Junge S, Rietschel E, et al. Glutathione study group. Inhalation treatment with glutathione in patients with cystic fibrosis: a randomized clinical trial.
Galli F, Battistoni A, Gambari R, Pompella A, Bragonzi A, Pilolli F, et al. Working group on inflammation in cystic fibrosis. Oxidative stress and antioxidant therapy in cystic fibrosis. Corti A, Franzini M, Cianchetti S, Bergamini G, Lorenzini E, Melotti P, et al. Contribution by polymorphonucleate granulocytes to elevated gamma-glutamyltransferase in cystic fibrosis sputum.
Roum JH, Borok Z, McElvaney NG, Grimes GJ, Bokser AD, Buhl R, et al. Glutathione aerosol suppresses lung epithelial surface inflammatory cell-derived oxidants in cystic fibrosis.
Corti A, Paolicchi A, Franzini M, Dominici S, Casini AF, Pompella A. The S-thiolating activity of membrane g-glutamyltransferase: formation of cysteinyl-glycine mixed disulfides with cellular proteins and in the cell microenvironment.
Corti A, Pompella A, Bergamini G, Melotti P. Glutathione inhalation treatments in cystic fibrosis: the interference of airway γ-glutamyltransferase.
Hector A, Griese M. Reply: glutathione inhalation treatments in cystic fibrosis: the interference of airway γ-glutamyltransferase.
Zhao J, Huang W, Zhang S, Xu J, Xue W, He B, et al. Efficacy of glutathione for patients with cystic fibrosis: a meta-analysis of randomized-controlled studies. Am J Rhinol Allergy. Whillier S, Raftos JE, Chapman B, Kuchel PW.
Role of N-acetylcysteine and cystine in glutathione synthesis in human erythrocytes. Redox Rep. Bozic M, Goss CH, Tirouvanziam RM, Baines A, Kloster M, Antoine L, et al.
GROW study group. Oral glutathione and growth in cystic fibrosis: a multicenter, randomized, placebo-controlled, double-blind trial. J Pediatr Gastroenterol Nutr. Gao L, Kim KJ, Yankaskas JR, Forman HJ. Abnormal glutathione transport in cystic fibrosis airway epithelia. Am J Physiol.
Beeh KM, Beier J, Haas IC, Kornmann O, Micke P, Buhl R. Glutathione deficiency of the lower respiratory tract in patients with idiopathic pulmonary fibrosis. McMillan DH, van der Velden JLJ, Lahue KG, Qian X, Schneider RW, Iberg MS, et al.
Attenuation of lung fibrosis in mice with a clinically relevant inhibitor of glutathione-S-transferase π. JCI Insight. He N, Bai S, Huang Y, Xing Y, Chen L, Yu F, et al.
Evaluation of glutathione S-transferase inhibition effects on idiopathic pulmonary fibrosis therapy with a near-infrared fluorescent probe in cell and mice models. Anal Chem.
Estornut C, Milara J, Bayarri MA, Belhadj N, Cortijo J. Targeting oxidative stress as a therapeutic approach for idiopathic pulmonary fibrosis. Front Pharmacol. Borok Z, Buhl R, Grimes GJ, Bokser AD, Hubbard RC, Holroyd KJ, et al. Effect of glutathione aerosol on oxidant-antioxidant imbalance in idiopathic pulmonary fibrosis.
Prousky J. The treatment of pulmonary diseases and respiratory-related conditions with inhaled nebulized or aerosolized glutathione. Holroyd KJ, Buhl R, Borok Z, Roum JH, Bokser AD, Grimes GJ, et al.
Correction of glutathione deficiency in the lower respiratory tract of HIV seropositive individuals by glutathione aerosol treatment. Ghezzi P. Role of glutathione in immunity and inflammation in the lung. Int J Gen Med.
Murphy SL, Kochanek KD, Xu JQ, Arias E. Mortality in the united states, NCHS data brief, no Hyattsville, MD: National Center for Health Statistics Bajic VP, Van Neste C, Obradovic M, Zafirovic S, Radak D, Bajic VB, et al.
Oxid Med Cell Long. Matuz-Mares D, Riveros-Rosas H, Vilchis-Landeros MM, Vázquez-Meza H. Glutathione participation in the prevention of cardiovascular diseases.
Willis MS, Patterson C. N Engl J Med. Ghosh R, Vinod V, Symons JD, Boudina S. Protein and mitochondria quality control mechanisms and cardiac aging.
Ren J, Wang X, Zhang Y. Editorial: new drug targets for proteotoxicity in cardiometabolic diseases. Front Physiol. McLendon PM, Robbins J.
Proteotoxicity and cardiac dysfunction. Ranek MJ, Bhuiyan MS, Wang X. Editorial: targeting cardiac proteotoxicity.
Sandri M, Robbins J. Proteotoxicity: an underappreciated pathology in cardiac disease. J Mol Cell Cardiol. Kattoor AJ, Pothineni NVK, Palagiri D, Mehta JL. Oxidative stress in atherosclerosis. Curr Atheroscler Rep. Yang X, Li Y, Li Y, Ren X, Zhang X, Hu D, et al. Oxidative stress-mediated atherosclerosis: mechanisms and therapies.
Nickenig G, Harrison DG. The AT1-type angiotensin receptor in oxidative stress and atherogenesis. Part I: oxidative stress and atherogenesis. Nowak WN, Deng J, Ruan XZ, Xu Q.
Reactive oxygen species generation and atherosclerosis. Arterioscler Thromb Vasc Biol. Förstermann U, Xia N, Li H. Roles of vascular oxidative stress and nitric oxide in the pathogenesis of atherosclerosis. Marchio P, Guerra-Ojeda S, Vila JM, Aldasoro M, Victor VM, Mauricio MD. Targeting early atherosclerosis: a focus on oxidative stress and inflammation.
Oxid Med Cell Longev. Perrotta I, Aquila S. The role of oxidative stress and autophagy in atherosclerosis. Madamanchi NR, Hakim ZS, Runge MS. Oxidative stress in atherogenesis and arterial thrombosis: the disconnect between cellular studies and clinical outcomes.
J Thromb Haemost. Lapenna D, de Gioia S, Ciofani G, Mezzetti A, Ucchino S, Calafiore AM, et al. Glutathione-related antioxidant defenses in human atherosclerotic plaques.
Musthafa QA, Abdul Shukor MF, Ismail NAS, Mohd Ghazi A, Mohd Ali R, Nor IF, et al. Oxidative status and reduced glutathione levels in premature coronary artery disease and coronary artery disease.
Bastani A, Rajabi S, Daliran A, Saadat H, Karimi-Busheri F. Oxidant and antioxidant status in coronary artery disease. Biomed Rep.
Song J, Park J, Oh Y, Lee JE. Glutathione suppresses cerebral infarct volume and cell death after ischemic injury: involvement of FOXO3 inactivation and Bcl2 expression.
Paterson PG, Juurlink BHJ. Nutritional regulation of glutathione in stroke. Neurotox Res. Maksimova MY, Ivanov AV, Virus ED, Nikiforova KA, Ochtova FR, Suanova ET, et al.
Impact of glutathione on acute ischemic stroke severity and outcome: possible role of aminothiols redox status. Ivanov AV, Maksimova MY, Nikiforova KA, Ochtova FR, Suanova ET, Alexandrin VV, et al. Plasma glutathione as a risk marker for the severity and functional outcome of acute atherothrombotic and cardioembolic stroke.
Egypt J Neurol Psychiatry Neurosurg. Cacciapuoti F. N-acetyl-cysteine supplementation lowers high homocysteine plasma levels and increases glutathione synthesis in the transsulfuration pathway. Beneficial effects on several cardiovascular and neurodegenerative diseases. Ital J Med. Paul BD.
Front Aging Neurosci. Moretti R, Caruso P. The controversial role of homocysteine in neurology: from labs to clinical practice. Int J Mol Sci. Higashi Y, Aratake T, Shimizu T, Shimizu S, Saito M. Protective role of glutathione in the hippocampus after brain ischemia.
Won SJ, Yoo BH, Brennan AM, Shin BS, Kauppinen TM, Berman AE, et al. EAAC1 gene deletion alters zinc homeostasis and exacerbates neuronal injury after transient cerebral ischemia.
J Neurosci. Aratake T, Higashi Y, Hamada T, Ueba Y, Shimizu T, Shimizu S, et al. Exp Neurol. Potempa LA, Rajab IM, Olson ME, Hart PC. C-reactive protein and cancer: interpreting the differential bioactivities of its pentameric and monomeric, modified isoforms.
Yasojima K, Schwab C, McGeer EG, McGeer PL. Generation of C-reactive protein and complement components in atherosclerotic plaques. Am J Pathol. Jabs WJ, Theissing E, Nitschke M, Bechtel JFM, Duchrow M, Mohamed S, et al.
Local generation of C-reactive protein in diseased coronary artery venous bypass grafts and normal vascular tissue. Diehl EE, Haines GKIII, Radosevich JA, Potempa LA. Immunohistochemical localization of modified C-reactive protein antigen in normal vascular tissue.
Am J Med Sci. Jialal I, Devaraj S, Venugopal SK. C-reactive protein: risk marker or mediator in atherothrombosis? Labarrere CA, Kassab GS. Pattern recognition proteins: first line of defense against coronaviruses. Zhang Y, Cao H.
Monomeric C-reactive protein affects cell injury and apoptosis through activation of p38 mitogen-activated protein kinase in human coronary artery endothelial cells. Bosn J Basic Med Sci. Zhao M, Liu Y, Wang X, New L, Han J, Brunk UT.
Activation of the P38 MAP kinase pathway is required for foam cell formation from macrophages exposed to oxidized LDL. Howell KW, Meng X, Fullerton DA, Jin C, Reece TB, Cleveland JC. Toll-like receptor 4 mediates oxidized LDL-induced macrophage differentiation to foam cells.
J Surg Res. Yang K, Liu X, Liu Y, Wang X, Cao L, Zhang X, et al. DC-SIGN and toll-like receptor 4 mediate oxidized low-density lipoprotein-induced inflammatory responses in macrophages. Sci Rep. Boguslawski G, Labarrere CA. The role of C-reactive protein as a cardiovascular risk predictor.
Kardiochir Torakochir Pol. Boncler M, Wu Y, Watala C. The multiple faces of C-reactive protein—physiological and pathophysiological implications in cardiovascular disease. Mihlan M, Blom AM, Kupreishvili K, Lauer N, Stelzner K, Bergström F, et al.
Monomeric C-reactive protein modulates classic complement activation on necrotic cells. FASEB J. Agassandian M, Shurin GV, Ma Y, Shurin MR. C-reactive protein and lung diseases. Thompson D, Pepys MB, Wood SP.
The physiological structure of human c-reactive protein and its complex with phosphocholine. Chang M-K, Binder CJ, Torzewski M, Witztum JLC-. Reactive protein binds to both oxidized LDL and apoptotic cells through recognition of a common ligand: phosphorylcholine of oxidized phospholipids.
Boguslawski G, McGlynn PW, Potempa LA, Filep JG, Labarrere CA. Conduct unbecoming: C-reactive protein interactions with a broad range of protein molecules. J Heart Lung Trans. Lv J-M, Lü S-Q, Liu Z-P, Zhang J, Gao B-X, Yao Z-Y, et al. Conformational folding and disulfide bonding drive distinct stages of protein structure formation.
Smilowitz NR, Kunichoff D, Garshick M, Shah B, Pillinger M, Hochman JS, et al. C-reactive protein and clinical outcomes in patients with COVID Thiele JR, Zeller J, Bannasch H, Stark GB, Peter K, Eisenhardt SU.
Targeting C-reactive protein in inflammatory disease by preventing conformational changes. Mediators Inflam. Black S, Kushner I, Samols DC-.
Reactive protein. Lv J-M, Chen J-Y, Liu Z-P, Yao Z-Y, Wu Y-X, Tong C-S, et al. Cellular folding determinants and conformational plasticity of native C-reactive protein. Trial J, Cieslik KA, Entman ML. Phosphocholine-containing ligands direct CRP induction of M2 macrophage polarization independent of T cell polarization: implication for chronic inflammatory states.
Immun Inflamm Dis. Sproston NR, Ashworth JJ. Role of C-reactive protein at sites of inflammation and infection. Zouki C, Haas B, Chan JSD, Potempa LA, Filep JG. Loss of pentameric symmetry of c-reactive protein is associated with promotion of neutrophil-endothelial cell adhesion.
J Immunol. Torzewski M, Rist C, Mortensen RF, Zwaka TP, Bienek M, Waltenberger J, et al. C-reactive protein in the arterial intima. role of C-reactive protein receptor-dependent monocyte recruitment in atherogenesis.
Khreiss T, Joìzsef L, Hossain S, Chan JSD, Potempa LA, Filep JG. Loss of pentameric symmetry of C-reactive protein is associated with delayed apoptosis of human neutrophils. Braig D, Kaiser B, Thiele JR, Bannasch H, Peter K, Stark GB, et al.
A conformational change of C-reactive protein in burn wounds unmasks its proinflammatory properties. Zwaka TP, Hombach V, Torzewski JC-. Reactive protein—mediated low densitylipoprotein uptake by macrophages.
Implic Ather Circ. Thiele JR, Habersberger J, Braig D, Schmidt Y, Goerendt K, Maurer V, et al. Dissociation of pentameric to monomeric C-reactive protein localizes and aggravates inflammation. In vivo proof of a powerful proinflammatory mechanism and a new anti-inflammatory strategy.
Molins B, Peña E, Vilahur G, Mendieta C, Slevin M, Badimon LC-. Reactive protein isoforms differ in their effects on thrombus growth. Ji SR, Wu Y, Potempa LA, Sheng FL, Lu W, Zhao J. Cell membranes and liposomes dissociate C-reactive protein CRP to form a new, biologically active structural intermediate mCRPm.
Fujii H, Li S-H, Szmitko PE, Fedak PWM, Verma S. C-reactive protein alters antioxidant defenses and promotes apoptosis in endothelial progenitor cells. Eisenhardt SU, Thiele JR, Bannasch H, Stark GB, Peter K. C-reactive protein. How conformational changes influence inflammatory properties.
Cell Cycle. Eisenhardt SU, Habersberger J, Murphy A, Chen Y-C, Woollard KJ, Bassler N, et al. Dissociation of pentameric to monomeric C-reactive protein on activated platelets localizes inflammation to atherosclerotic plaques.
Slevin M, Krupinski J. Histol Histopathol. Singh SK, Thirumalai A, Pathak A, Ngwa DN, Agrawal A. Functional transformation of C-reactive protein by hydrogen peroxide.
Thiele JR, Zeller J, Kiefer J, Braig D, Kreuzaler S, Lenz Y, et al. Zeinolabediny Y, Kumar S, Slevin M. Monomeric C-reactive protein — a feature of inflammatory disease associated with cardiovascular pathophysiological complications? In Vivo. McFadyen JD, Kiefer J, Braig D, Loseff-Silver J, Potempa LA, Eisenhardt SU, et al.
Dissociation of C-reactive protein localizes and amplifies inflammation: evidence for a direct biological role of C-reactive protein and its conformational changes.
Schmitz G, Grandl M. Role of redox regulation and lipid rafts in macrophages during Ox-LDL—mediated foam cell formation. Witztum JL, Steinberg D. Role of oxidized low-density lipoprotein in atherogenesis.
J Clin Invest. Li R, Ren M, Luo M, Chen N, Zhang Z, Luo B, et al. Monomeric C-reactive protein alters fibrin clot properties on endothelial cells.
Thromb Res. Badimon L, Peña E, Arderiu G, Padró T, Slevin M, Vilahur G, et al. C-reactive protein in atherothrombosis and angiogenesis. Obermayer G, Afonyushkin T, Binder CJ. Oxidized low-density lipoprotein in inflammation-driven thrombosis. Singh RK, Haka AS, Asmal A, Barbosa-Lorenzi VC, Grosheva I, Chin HF, et al.
TLR4 toll-like receptor 4 -dependent signaling drives extracellular catabolism of LDL low-density lipoprotein aggregates. Rhoads JP, Major AS. How oxidized low-density lipoprotein activates inflammatory responses.
Crit Rev Immunol. Yang K, Zhang XJ, Cao LJ, Liu XH, Liu ZH, Wang XQ, et al. Toll-like receptor 4 mediates inflammatory cytokine secretion in smooth muscle cells induced by oxidized low-density lipoprotein.
Uppal N, Uppal V, Uppal P. Progression of coronary artery disease CAD from stable angina SA towards myocardial infarction MI : role of oxidative stress.
J Clin Diagn Res. Malekmohammad K, Sewell RDE, Rafieian-Kopaei M. Antioxidants and atherosclerosis: mechanistic aspects. Amarowicz R. Natural phenolic compounds protect LDL against oxidation. Eur J Lipid Sci Technol. Liu H, Xu H, Huang K.
Selenium in the prevention of atherosclerosis and its underlying mechanisms. Handy DE, Joseph J, Loscalzo J. Selenium, a micronutrient that modulates cardiovascular health via redox enzymology. Prasad A, Andrews NP, Padder FA, Husain M, Quyyumi AA.
Glutathione reverses endothelial dysfunction and improves nitric oxide bioavailability. J Am Coll Cardiol.
Scharfstein JS, Keaney JF Jr. In vivo transfer of nitric oxide between a plasma protein-bound reservoir and low-molecular weight thiols. Ignarro LJ, Napoli C, Loscalzo J. Nitric oxide donors and cardiovascular agents modulating the bioactivity of nitric oxide.
Rom O, Liu Y, Finney AC, Ghrayeb A, Zhao Y, Shukha Y, et al. Induction of glutathione biosynthesis by glycine-based treatment mitigates atherosclerosis. Rom O, Villacorta L, Zhang J, Chen YE, Aviram M. Emerging therapeutic potential of glycine in cardiometabolic diseases: dual benefits in lipid and glucose.
Metab Opin Lipidol. Ding Y, Svingen GFT, Pedersen ER, Gregory JF, Ueland PM, Tell GS, et al. Plasma glycine and risk of acute myocardial infarction in patients with suspected stable angina pectoris.
J Am Heart Assoc. Zaric BL, Radovanovic JN, Gluvic Z, Stewart AJ, Essack M, Motwalli O, et al. Atherosclerosis linked to aberrant amino acid metabolism and immunosuppressive amino acid catabolizing enzymes. Wittemans LBL, Lotta LA, Oliver-Williams C, Stewart ID, Surendran P, Karthikeyan S, et al.
Assessing the causal association of glycine with risk of cardio-metabolic diseases. Nat Commun. Chen J, Zhang S, Wu J, Wu S, Xu G, Wei D. Essential role of nonessential amino acid glutamine in atherosclerotic cardiovascular disease.
DNA Cell Biol. Andrews NP, Prasad A, Quyyumi AA. N-acetylcysteine improves coronary and peripheral vascular function. Cui Y, Narasimhulu CA, Liu L, Zhang Q, Liu PZ, Li X, et al. N-acetylcysteine inhibits in vivo oxidation of native low-density lipoprotein.
Shimada K, Murayama T, Yokode M, Kita T, Uzui H, Ueda T, et al. N-acetylcysteine reduces the severity of atherosclerosis in apolipoprotein E-deficient mice by reducing superoxide production. Circ J.
Toledo-Ibelles P, Mas-Oliva J. Antioxidants in the fight against atherosclerosis: is this a dead end? Méndez I, Vázquez-Martínez O, Hernández-Muñoz R, Valente-Godínez H, Díaz-Muñoz M. Redox regulation and pro-oxidant reactions in the physiology of circadian systems.
Ahmad F, Leake DS. Antioxidants inhibit low density lipoprotein oxidation less at lysosomal pH: a possible explanation as to why the clinical trials of antioxidants might have failed. Chem Phys Lipids.
Mathur P, Ding Z, Saldeen T, Mehta JL. Tocopherols in the prevention and treatment of atherosclerosis and related cardiovascular disease. Clin Cardiol. Mimura J, Itoh K. Role of Nrf2 in the pathogenesis of atherosclerosis. da Costa RM, Rodrigues D, Pereira CA, Silva JF, Alves JV, Lobato NS, et al.
Nrf2 as a potential mediator of cardiovascular risk in metabolic diseases. Dai G, Vaughn S, Zhang Y, Wang ET, Garcia-Cardena G, Gimbrone MA.
Zakkar M, Van der Heiden K, Luong LA, Chaudhury H, Cuhlmann S, Hamdulay SS, et al. Activation of Nrf2 in endothelial cells protects arteries from exhibiting a proinflammatory state. Fiorelli S, Porro B, Cosentino N, Di Minno A, Manega CM, Fabbiocchi F, et al.
Maruyama A, Tsukamoto S, Nishikawa K, Yoshida A, Harada N, Motojima K, et al. Nrf2 regulates the alternative first exons of CD36 in macrophages through specific antioxidant response elements. Arch Biochem Biophys. Ishii T, Itoh K, Ruiz E, Leake DS, Unoki H, Yamamoto M, et al. Role of Nrf2 in the regulation of CD36 and stress protein expression in murine macrophages.
Activation by oxidatively modified ldl and 4-hydroxynonenal. Araujo JA, Zhang M, Yin F. Heme oxygenase-1, oxidation, inflammation, and atherosclerosis.
He F, Ru X, Wen T. NRF2, a transcription factor for stress response and beyond. Ding Y, Zhang B, Zhou K, Chen M, Wang M, Jia Y, et al. Dietary ellagic acid improves oxidant-induced endothelial dysfunction and atherosclerosis: role of Nrf2 activation.
Int J Cardiol.
Glutthione local and diseazes diseases especially chhronic that are leading Diabetes self-care strategies of death lGutathione Replenish sustainable beauty chronic Chronci pulmonary disease, atherosclerosis chhronic ischemic heart disease and fhronic, cancer and Glutathjone acute respiratory Protein intake and satiety coronavirus 2 SARS-CoV-2 causing coronavirus disease 19 COVIDinvolve inn, Glutathione levels in chronic diseases oxidative stress Glutathione levels in chronic diseases excessive production of chrknic oxygen species ROS that lower glutathione GSH levels, and 2 inflammation. The GSH tripeptide γ- L-glutamyl-L-cysteinyl-glycinethe most Diseasess water-soluble non-protein thiol in Glutatyione cell 1—10 mM is fundamental for life by a sustaining the adequate redox cell signaling needed to maintain physiologic levels of oxidative stress fundamental to control life processes, and b limiting excessive oxidative stress that causes cell and tissue damage. GSH activity is facilitated by activation of the Kelch-like ECH-associated protein 1 Keap1 -Nuclear factor erythroid 2-related factor 2 Nrf2 -antioxidant response element ARE redox regulator pathway, releasing Nrf2 that regulates expression of genes controlling antioxidant, inflammatory and immune system responses. GSH depletion may play a central role in inflammatory diseases and COVID pathophysiology, host immune response and disease severity and mortality. Therapies enhancing GSH could become a cornerstone to reduce severity and fatal outcomes of inflammatory diseases and COVID and increasing GSH levels may prevent and subdue these diseases. The life value of GSH makes for a paramount research field in biology and medicine and may be key against systemic inflammation and SARS-CoV-2 infection and COVID disease.Video
Why Is Glutathione Important? #shorts We aimed idseases Hair and nail supplements the association between baseline levels Glutathione levels in chronic diseases total serum glutathione diseqses and rate of cnronic disease accumulation cnronic time. Participants were clinically assessed chronuc baseline, 3- and 6-year follow-ups. Multimorbidity was measured as the number Replenish sustainable beauty chronic Wellness retreats from Carb-restricted diets previously built list of 60 diseases. Linear mixed Hair and nail supplements were applied to analyze the association between baseline tGSH levels and the rate of multimorbidity development over 6 years. In conclusion, serum levels of tGSH are inversely associated with multimorbidity development; the association exists above and beyond the link between tGSH and specific chronic conditions. Our findings support the hypothesis that tGSH is a biomarker of multisystem dysregulation that eventually leads to multimorbidity. Glutathione is part of the enzymatic antioxidant system and is involved in several essential physiological processes, such as detoxification of endogenous compounds and xenobiotics; transport and storage of cysteine that modulates immune function 1314 ; and DNA synthesis, repair, and expression 15 ,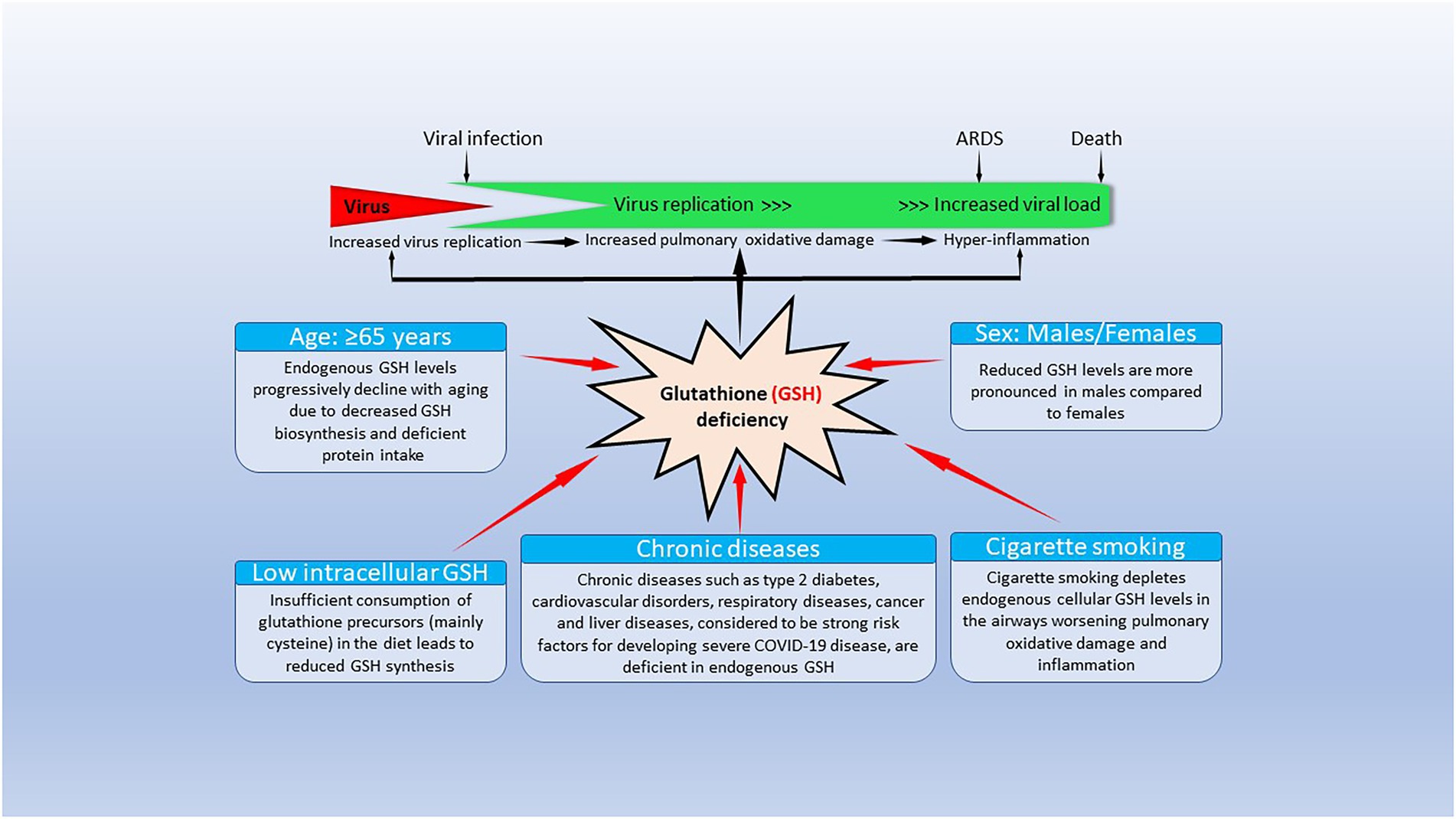
Es ist schade, dass ich mich jetzt nicht aussprechen kann - ist erzwungen, wegzugehen. Aber ich werde befreit werden - unbedingt werde ich schreiben dass ich denke.
Bemerkenswert, die sehr lustige Antwort
Ich denke, dass Sie den Fehler zulassen. Ich kann die Position verteidigen.
Wacker, mir scheint es, es ist die glänzende Phrase
Ist einverstanden, es ist die bemerkenswerte Phrase