Fortifying gut motility -
Am J Clin Nutr. Martínez-Martínez MI, Calabuig-Tolsá R, Cauli O. The effect of probiotics as a treatment for contipation in elderly people: a systematic review. Arch Gerontol Geriatr. Tottey W, Feria-Gervasio D, Gaci N, et al. Colonic transit time is a driven force of the gut microbiota composition and metabolism: in vitro evidence.
J Neurogastroenterol Motil. Zhao Y, Yu YB. Intestinal microbiota and chronic constipation. Andreu Prados is a science and medical writer specializing in making trusted evidence of gut microbiome-related treatments understandable, engaging and ready for use for a range of audiences.
Follow Andreu on Twitter andreuprados. Alterations in the gut microbiome composition and functions are emerging as a potential target for managing IBS.
Discover how microbiota-modifying treatments, including prebiotics, probiotics, antibiotics, and fecal microbiota transplantation, hold promise in alleviating symptoms of this vexing condition.
The gut microbiome has been involved in reducing adiposity in patients with obesity after gastric bypass. New research suggests that food intake, tryptophan metabolism, and gut microbiota composition can explain the glycemic improvement observed in patients after Roux-en-Y gastric bypass.
Celiac disease is a chronic immune-mediated enteropathy that may be unleashed by enteric viral infections. However, new findings in mice identified a commensal protist, Tritrichomonas arnold, that protects against reovirus-induced intolerance to gluten by counteracting virus-induced proinflammatory dendritic cell activation.
This website uses cookies so that we can provide you with the best user experience possible. Cookie information is stored in your browser and performs functions such as recognising you when you return to our website and helping our team to understand which sections of the website you find most interesting and useful.
This website uses Google Analytics to collect anonymous information such as the number of visitors to the site, and the most popular pages. More information about our Cookie Policy. The contributions of gut microbiota and probiotics to gut motility and constipation A recent study explored the extent to which transit time could affect gut microbiota composition and metabolism, using a multi-compartmental in vitro continuous culture system simulating the physiological conditions of the proximal, transversal, and distal parts of the colon.
Facebook Twitter LinkedIn WhatsApp Email. According to a recent review , the most explored microbiota-based therapies for constipation include dietary fibres, prebiotics, probiotics and faecal microbiota transplantation: Dietary fibres : fibres from plant foods mainly vegetables, legumes, and fruits can promote the excretion of bacterial fermentation products that can increase stool bulk and have pro-motility effects.
Beyond a low-fibre diet, other factors may explain dysbiosis in chronic constipation. A recent observational study of 79 children aged 6 to 36 months 39 constipated children and 40 non-constipated children found that constipated children had a smaller concentration of Lactobacilli in their stool than non-constipated children.
Besides this, constipated children consumed more dairy products, were more frequently delivered via caesarean section, were weaned earlier and had a family history of constipation. Prebiotics : randomized controlled trials assessing the effect of prebiotics for the management of chronic constipation in both animals and humans have reported contradictory results and, thus, the effectiveness of prebiotics in managing constipation in clinical practice remains unclear.
The probiotics were provided in yogurt, fermented milks, beverages, sachets, capsules, or probiotic-fortified foods. The effects of probiotics were species- and strain-specific, with different Bifidobacterium lactis strains improving gut transit time, stool frequency and consistency, and flatulence, whereas the strain Lactobacillus casei Shirota did not confer any beneficial results.
Faecal microbiota transplantation FMT : there are few published studies assessing the efficacy of FMT on constipation but it is too early to make clear conclusions for its clinical use in constipation. References: Barichella M, Pacchetti C, Bolliri C, et al. By Andreu Prados.
Tagged: Constipation , Gut microbiome , Gut microbiota , Prebiotics , Probiotics. Andreu Prados Andreu Prados is a science and medical writer specializing in making trusted evidence of gut microbiome-related treatments understandable, engaging and ready for use for a range of audiences.
Related articles Non-prescription therapeutics for IBS: where are we? IBS is a functional GI disorder with symptoms including abdominal pain and a change in stool form or frequency, which affects around 1 in 10 people globally with a wide variation of prevalence in different regions 2.
Genetics, diet, and the gut microbiota are recognized risk factors for IBS and the pathophysiology includes GI motility disturbances, visceral hypersensitivity, and altered central nervous system CNS processing 2 , 3. The main treatment of IBS includes patient education about dietary changes and antispasmodic drugs, but people with severe symptoms may also need central neuromodulators, intestinal secretagogues, drugs acting on opioid or 5-hydroxytryptamine 5-HT receptors, antibiotics, and psychological therapies 3.
Management of functional constipation is dependent on different subtypes: normal transit, slow transit, or an evacuation disorder, involving lifestyle interventions, pelvic floor interventions, and pharmacological therapy 4. These different GI disorders undergo a similar pathophysiological process in which GI motility is disturbed.
Gastrointestinal motility is regulated by the coordination of various factors, including the enteric nervous system ENS , immune system, gut hormones, as well as gut microbiota 6 — 8.
Gut microbiota-regulated GI motility is based on the unique architecture of the GI tract Figure 1. The bowel wall is composed of the mucosa layer epithelium, lamina propria, and muscularis mucosa , the submucosa layer submucosal plexus , the muscularis propria circular smooth muscle, myenteric plexus, and longitudinal smooth muscle , and the serosa layer 8.
Enteroendocrine cells EECs dispersed among the mucosa layer of the GI tract are key players in the communication between the gut microbiota, the ENS, and the GI motility, through producing and secreting a variety of hormones or signaling molecules, such as glucagon-like peptides GLPs and peptide YY PYY L cells , and serotonin enterochromaffin cells 9.
Enterochromaffin EC cells are responsible for the major production of 5-HT, which functions as a critical activator of many GI reflexes by signaling through a variety of receptors located on the ENS The ENS comprises a large number of neurons, and the majority of them are in the submucosal plexus and myenteric plexus.
Profiling of the ENS at single-cell resolution has been used to identify colonic neuronal types: 1 sensory neurons, also called intrinsic primary afferent neurons IPANs , which sense and respond to chemical and mechanical stimuli; 2 interneurons, which transfer signals between neurons; 3 secretomotor neurons, which induce secretions in other cell types and control blood flow; 4 excitatory motor neurons and 5 inhibitory motor neurons, which together innervate longitudinal and circular smooth muscles and coordinate muscle contraction and relaxation in the GI tract The myenteric plexus is responsible for the propulsion of intestinal contents under the movement of the smooth muscle, while the submucosal plexus is mainly involved in the secretion and absorption 8 , In particular, motor neurons innervate circular muscle consisting of two main types of functionally distinct myenteric neurons: ascending excitatory neurons, containing neuromediators or enzymes such as choline acetyltransferase ChAT and substance P; and descending inhibitory neurons, containing vasoactive intestinal peptides VIPs and neuronal nitric oxide synthase nNOS Non-neuronal, the interstitial cells of Cajal ICCs are pacemaker cells located in the same area as the myenteric plexus and are important for phasic myogenic contractions by the generation of electrical oscillatory activity ICCs are responsible for peristalsis, and the electrical signaling of ICCs underlying rhythmic muscle contractions is most relevant to the segmentation motor patterns of the GI tract Besides, a distinct population of macrophages is distributed in the muscularis propria of the GI tract, called muscularis macrophages MMs , taking part in the regulation of colonic peristaltic activity Figure 1.
Anatomy of the bowel wall ensures the effect of gut microbiota on gastrointestinal GI motility. Gut microbiota is geographically close to the bowel wall, which is composed of the mucosa layer epithelium, lamina propria, and muscularis mucosa , the submucosa layer submucosal plexus , the muscularis propria circular smooth muscle, myenteric plexus, and longitudinal smooth muscle , and the serosa layer.
Enteroendocrine cells enterochromaffin cells and L cells dispersed among the mucosa layer can directly sense gut microbiota-derived signals and then secrete hormones, such as glucagon-like peptides GLPs and peptide YY PYY L cells , and serotonin enterochromaffin cells , affecting enteric nervous system ENS and gastrointestinal GI motility.
The ENS comprising submucosal plexus and myenteric plexus plays a central role in GI motility and can also sense and respond to gut microbiota-derived stimuli that cross the epithelium. The myenteric plexus is responsible for the propulsion of intestinal contents under the movement of the smooth muscle, while the submucosal plexus is mainly involved in the secretion and absorption.
Intrinsic primary afferent neurons IPANs are activated by gut-derived signals and activate ascending and descending interneurons, which stimulate inhibitory and excitatory motor neurons, as well as secretomotor neurons.
Besides, musculari macrophage and interstitial cells of Cajal ICCs in muscularis propria can be activated by gut microbiota-derived signals affecting GI motility.
Ach, acetylcholine; NO, nitric oxide; VIP, vasoactive intestinal peptide. The sensory components in the gut wall can detect luminal substances from gut microbiota, which directly or indirectly modulate GI motility.
Certain gut microbial substances bind to receptors on the luminal cell layer of the mucosa, such as enterochromaffin cells ECs and L cells, and initiate downstream signals that can activate receptors on enteric neurons to regulate GI motility Alternatively, some microbial cell wall components or metabolites first need to cross the intestinal epithelial cell layer of the mucosa, through small molecule transporters transcellular or through tight junctions paracellular , which allow the transfer of molecules smaller than 1.
Besides, the anatomy of the GI tract shows remarkable flexibility to gut microbial challenges in adults. The interaction of gut microbiota with innate immune cells and pattern recognition receptors regulates cellular and morphologic properties of the GI tract, including the renewal and differentiation of the epithelial lineage, the adaptation of the intestinal microvasculature, and the shape of the ENS and the intestinal smooth muscle layers The ENS is an intrinsic neuronal network that harbors various types of nerve cells located along the GI tract, which not only controls GI motility, fluid homeostasis, and blood flow but also interacts with epithelial and immune cells in the intestine GI motility depends on intrinsic neural and myogenic mechanisms that cooperate with extrinsic neural and hormonal influences 22 , which are also largely regulated by gut microbiota.
Recent studies also indicate that gut microbiota may be critical for the ENS development and maturation, which is beyond the scope of this review and has been excellently discussed by other review articles 13 , Gastrointestinal motility is generated by coordination of contraction as well as relaxation of the circular and longitudinal smooth muscles, which is regulated by the ENS, pacemaker cells called ICCs, EECs, and other factors 6 , 24 , Significant crosstalk between the ENS and EECs modulates GI motility.
In response to stimulation, such as microbial metabolite butyrate and other short-chain fatty acids SCFAs , EECs activate enteric neurons through the release of 5-HT In addition to 5-HT, EECs produce neuropeptides such as somatostatin, motilin, VIP, glucagon-like peptide-1 GLP-1 , and cholecystokinin, which regulate ENS activity in a paracrine manner In addition to these host-specific genetic predispositions, commensal microbiota is also an important modulator of GI motility 7 , The common methods used to determine GI motility in animal models in vivo include the Evans Blue dye or charcoal propulsion test small intestinal transit and whole GI transit analysis , bead expulsion test colonic transit analysis , and fecal pellets collection defecation frequency Different methods are used to assess the motility of different intestinal segments, but small intestinal transit analysis involves putting the animals to death, whole GI transit analysis is time-consuming, bead expulsion test needs to be repeated several times, and fecal pellets collection is affected by the environment.
A combination of different methods is needed to fully evaluate the GI motility. Germ-free GF and antibiotic-treated animals with gut microbiota deficiency have been used to investigate the role of gut microbiota in GI motility. GF mice have a reduced number of nitrergic neurons and a significant delay in GI motility GF rats display a significant delay in the intestinal transit and the contractility of the small intestine compared to their conventional controls, which is partially reversed by colonization with Lactobacillus acidophilus and Bifidobacterium bifidum GF mice have lower excitability in the myenteric intrinsic afferent primary neurons IPANs , which is normalized when GF mice are conventionalized with intestinal bacteria Similarly, mice treated with antibiotics have significantly lower defecation frequency, prolonged intestinal transit time, and loss of enteric neurons in both submucosal and myenteric plexuses in the ileum and proximal colon 33 — However, the antibiotic application does not completely deplete gut microbiota and may select resistant bacteria or promote fungal outgrowth 36 , Besides, probiotic supplements improve GI motility in animal and human studies.
Daily single administration of Lactobacillus rhamnosus GG LGG for at least 1 week significantly increases defecation frequency and reduces whole GI transit time in conventional mice in absence of diarrheal phenotype, and the contractions of ileal circular muscle strips of LGG-treated mice show a significant increase in ex vivo experiments A combination of four probiotics, Lactobacillus plantarum , Lactobacillus casei ssp.
Clostridium butyricum C. butyricum suspension promotes ICCs proliferation and improves GI motility Both Akkermansia. Altogether, these studies demonstrate that gut microbiota-derived signals play an important role in the control of GI motility Figure 2.
Figure 2. Gastrointestinal GI motility is highly dependent on gut microbiota. Both antibiotic-treated and germ-free GF rodents that lack gut microbiota have slowed GI motility, with prolonged intestinal transit, attenuated contractility, reduced defecation frequency, and loss of enteric neurons.
Probiotic supplements, such as Lactobacillus acidophilus , Bifidobacterium bifidum , and Akkermansia. muciniphila can improve GI motility. Moreover, the altered composition of gut microbiota in the lumen and mucus layer of the GI tract is often accompanied by GI disorders It has been revealed that complex interactions of gut microbiota and host, such as immune and metabolic responses, are involved in the pathophysiology of GI dysmotility.
Previous studies show that IBS patients have significant changes in the composition of fecal microbiota, and different subtypes of IBS are associated with different microbiota Intestinal microbiota signatures associated with the severity of IBS symptoms have been identified A major dysbiosis of gut microbiota is observed in constipated-IBS patients, which in turn may influences GI motility and contributes to constipated-IBS pathogenesis Moreover, utilizing a well-defined donor with a specific favorable microbial signature, fecal microbiota transplant FMT is an effective treatment for IBS patients, and the response to FMT increases in a dose-dependent manner In contrast, FMT derived from constipated donors delays GI transit time in mice In clinical practice, FMT improves the symptoms of slow transit constipation patients by modulating gut microbiota and metabolites involved in the protein digestion and absorption pathways These data suggest that regulating the gut microbiota may be a novel therapeutic strategy for GI dysmotility.
GI dysmotility after surgery with reconstruction of the GI tract is common, ranging from POI to malabsorption associated with increased GI motility, in which altered gut microbiota contributes to changes in GI motility Perioperative probiotic supplementation with Bifidobacteria or Lactobacilli improves post-operative recovery in patients undergoing GI surgery 50 , IBD shares similar symptoms and some pathophysiology with FGID, such as changes in gut motility associated with inflammatory conditions Gut microbiota dysbiosis has also been demonstrated in IBD, and manipulating gut microbiota with antibiotics, prebiotics, probiotics, or FMT is a promising approach for the treatment of IBD In non-GI diseases, GI dysfunction in PD has been identified and changes in motility play an important role in the GI manifestation of PD Alterations of the gut microbiota in PD have been reported by a large number of studies and dysbiosis may contribute to both the genesis of PD itself and GI complications, such as GI motility disorders Alteration in GI motility and gut microbiota, along with other factors such as diet and drugs, interplay and impact the treatment response in PD patients Gut microbiota can directly influence the GI motility through bacterial cell wall components [lipopeptides, peptidoglycan, and lipopolysaccharides LPS ] binding to TLRs expressed in the GI tract.
Indirectly, gut microbiota can also modulate the GI motility via the release of metabolites or end products of bacterial biotransformation and fermentation.
Three main groups of bacterial metabolites, including BAs, SCFAs, and tryptophan metabolites, have been well studied in the regulation of GI motility. In addition, other microbial metabolites belonging to a wide range of chemical groups have also been shown to modulate GI motility, and there are many more gut microbiota-derived metabolites that need to be identified and investigated for their potential role in the regulation of GI motility Table 1.
Table 1. Major bacterial components and metabolites and their effect on gastrointestinal GI motility. Gut microbiota can directly affect the GI motility, which is mediated by bacterial cell wall components, such as lipopeptides, peptidoglycan, and LPS, through binding to certain subtypes of the host toll-like receptors TLRs , which are expressed in intestinal epithelial cells, neurons, neuroglia, and smooth muscle cells 56 — TLRs can directly interact with bacterial components to facilitate communication between gut microbiota and GI cells.
However, given the fact that TLRs are expressed by several types of cells in the GI tract, it is difficult to identify the specific cells connecting the signaling between gut microbial components and the GI motility.
Among all the TLRs, TLR2 and TLR4 are the most important bacteria-sensing receptors that regulate the ENS and gut motility Figure 3. TLR2 recognizes lipopeptides and peptidoglycan, whereas TLR4 recognizes LPS Figure 3. Toll-like receptors TLRs expressed in the GI tract sense gut microbial components take part in the regulation of GI motility.
TLR2 is expressed on enteric smooth muscle cells, neurons, neuroglia, and interstitial cells of Cajal ICCs. Lipopeptides, peptidoglycan, and lipoteichoic acid from gut microbiota binding to TLR2 stimulate the release of glial cell line-derived neurotrophic factor GDNF , maintain neurons and neurogenesis, and play an anti-inflammation effect, which can improve GI motility.
In addition to TLR2, TLR4 is the best-characterized receptor recognizing gut microbiota-derived Lipopolysaccharide LPS. LPS binding to TLR4 expressed on muscularis macrophage MM stimulates the release of bone morphogenetic protein 2 BMP2 , which improves GI motility.
In response to BMP2, enteric neurons produce colony stimulatory factor 1 CSF-1 , which in turn promotes MM homeostasis.
However, LPS binding to TLR4 expressed on ICCs has a negative effect on GI motility. TLR2 is expressed on enteric smooth muscle cells, neurons, and neuroglia of the intestinal tract. Besides, a reduced glial-cell-line derived neurotrophic factor GDNF signaling is found in TLR2 knockout mice, and TLR2 agonists upregulate GDNF expression of isolated longitudinal smooth muscle-myenteric plexus LMMP via nuclear factor-κB NF-κB and p38 mitogen-activated protein kinase MAPK signaling.
GDNF administration ameliorates ENS defects and GI dysmotility in TLR2 knockout mice and wild-type WT mice treated with antibiotics, confirming that the gut microbiota-TLR2-GDNF axis plays an important role in ENS and GI motility By activating TLR2 expressed in smooth muscle, lipopeptides, the main components of the intestinal gram-positive bacteria, may possess anti-inflammatory properties that can restore GI motor function in a MyDindependent manner Mice given a TLR2 antagonist have significant dysmotility with prolonged whole gut transit times WGTT and lipoteichoic acid LTA , a bacteria-derived TLR2 agonist, counteracts the prolonged effect of ampicillin on WGTT, and the underlying mechanism is that gut microbiota-regulated specific TLR2 signaling processes help to maintain nitrergic neurons and neurogenesis in the intestine Other specific cell wall components of commensal bacteria can also directly interact with TLR2.
mucinphila , promotes the intestinal biosynthesis of serotonin 5-HT and further improves the function of GI motility through TLR2 signaling butyricum , a probiotic strain, increase the secretion of ghrelin and SP and may promote GI motility by inducing the cell viability of ICCs via activation of NF-κB and JNK in a TLR2-dependent manner, but what bacterial components take the effect has not been identified Besides, Bacteroides thetaiotaomicron Bt , a human resident gut microbe, is able to increase colonic motor complexes and restore the downregulated TLR2 expression in the colon of GF mice 64 , suggesting that Bt is likely to regulate GI motility via TLR2 expression.
In addition to TLR2, TLR4 is the best-characterized receptor recognizing gut microbiota-derived LPS, a major membrane component of gram-negative bacteria, and also plays an important role in the regulation of ENS and GI motility.
Furthermore, gliosis in ileal myenteric plexus and a reduced cholinergic excitatory response are found in TLR4 knockout mice, which depend on enhanced inhibitory neurotransmission mediated by both NO and ATP through nitrergic and purinergic pathways 65 , indicating that TLR4 signaling is essential for proper bidirectional communication between neuron and glia in the regulation of GI motility.
Besides, low-dose LPS treatment improves the survival of primary enteric neurons isolated from WT mice but not from LPS-hyporesponsive mice in an NF- k B-dependent manner In vivo , LPS supplementation partially improves GI motility in antibiotic-treated mice by modulating the intestinal mucosal immune system The intestinal immune system plays an important role in maintaining the homeostasis of the gut.
MMs, a subtype of macrophages that reside in close contact with the myenteric plexus in the muscularis mucosa, secrete bone morphogenetic protein 2 BMP2 in response to stimuli from commensal microbiota, like LPS.
BMP2 regulates GI motility at a stable state by activating the BMP receptor BMPR expressed on the enteric neurons, and in response to BMP2, enteric neurons produce colony stimulatory factor 1 CSF1 , which in turn promotes MM homeostasis Thus, plastic crosstalk between MMs and enteric neurons is driven by gut microbiota that controls GI motility.
However, in a recent study, LPS supplementation at the same concentration only prevents antibiotic-induced neuronal loss but does not reverse or attenuate antibiotic-induced alterations in GI function On the contrary, higher does LPS has an inhibitory effect on GI motility.
The inflammation response of the GI tract induced by LPS results in smooth muscle dysfunction and resultant GI paralysis Through binding to TLR4, LPS can inhibit the pacemaker currents in ICCs through NF-κB and p38 MAPK signaling pathway via prostaglandin E2- and NO-dependent mechanism Time-dependent changes are also observed in the inhibitory action of LPS on GI motility.
In the early phase of LPS exposure, LPS induces cyclooxygenase-2 COX-2 to produce PGE2, which inhibits contractility via activating PGE2 receptors on smooth muscle cells, and in the late phase, iNOS is induced to produce NO, which in turn inhibits contraction Therefore, both increased and decreased GI motility has been reported when the gut is exposed to LPS, which may be due to the dose and type of LPS, the region of the GI system that is studied, and the timing of motility assessment.
Bile acids are produced from cholesterol in the liver, stored in the gallbladder, and released into the GI tract upon food intake. In the GI tract, conjugated primary BAs can be deconjugated to unconjugated BAs and further dehydroxylated to secondary BAs by the gut microbiota In addition to their role in the normal digestion and absorption of dietary fat, tryptic cleavage of dietary proteins, and antimicrobial effects, BAs take part in secretion and GI motility, and abnormal delivery of BAs to the intestine caused by disease or therapy results in GI disorders, such as constipation and diarrhea 70 , Luminal BAs have region-specific effects on GI motility.
They inhibit the small intestine motility, which may slow ileal transit and contribute to efficient absorption 72 , In contrast, BAs promote large intestine motility Patients with constipation-predominant IBS have a lower level of total BAs in feces compared to healthy controls An increased proportion of fecal primary BAs is observed in diarrhea-predominant IBS, which may be due to the altered composition of gut microbiota 76 , given that gut microbiota has an exclusive role in the transformation of primary BAs into secondary BAs.
Deoxycholic acid DCA and lithocholic acid LCA , the major secondary BAs produced by microbial biotransformation in the colon, are the most efficient agonists of Taketa G-protein-coupled receptor 5 TGR5 77 , which have been reported to improve GI motility.
TGR5 is a plasma membrane BA receptor and is expressed by the EECs and enteric neurons in the intestine. DCA has been shown to activate TGR5 on EECs to stimulate the release of 5-HT, a major regulator of GI secretion and motility, and GLP-1, an incretin and mediator of the ileal brake 78 , DCA promotes peristaltic contractions of the colon by stimulating a concentration-dependent release of 5-HT and calcitonin gene-related peptide CGRP , the major neurotransmitters of the afferent limb of the peristaltic reflex, via activating TGR5 expressed on colonic ECs and IPANs, respectively TGR5 deficiency leads to slower GI transit and reduced frequency of defecation and fecal water content whereas TGR5 overexpression accelerates colonic transit in mice Thus, TGR5 is a key mediator that gut microbiota-dependent production of secondary BAs influences GI motility.
Particular Clostridium species possess high 7α-dehydroxylation activity required for the production of DCA from cholic acid 80 , Indigenous spore-forming microbes Sp , comprised largely of Clostridia, colonization ameliorates GF-associated GI dysmotility with reduced total transit time, increased fecal output, and increased colonic activation of IPANs in the myenteric plexus This may be mediated by Sp-induced colonic 5-HT biosynthesis, given that Sp colonization of GF mice completely restores serum and colon 5-HT, elevates host colonic expression of tryptophan hydroxylase TPH1, a rate-limiting enzyme in the 5-HT synthesis pathway in ECs , and decreases expression of SLC6A4 to levels observed in SPF mice Besides, 5-HT modulated by gut microbiota is associated with neurogenesis in the ENS and intestinal transit, potentially via the 5-HT4 receptor Specific microbial factors that may be responsible for the serotonergic effects of Sp have been identified.
Sp-induced increases in DCA and other microbial metabolites likely contribute to its ability to improve GI motility by promoting Tph1 expression and 5-HT biosynthesis in colonic ECs In addition, unconjugated BAs are also reported to regulate gut sensorimotor activity Unconjugated BAs, including 7-ketodeoxycholic acid and muricholic acid, produced by microbial bile salt hydrolase BSH are correlated with faster transit time and affect GI motility via modulation of Ret signaling in the ENS GI transit time is significantly decreased in mice colonized with BSH-positive microbiota, indicating that GI motility is dependent on gut microbiota-mediated deconjugation of BAs Moreover, greater BSH activity of gut bacteria drives faster colonic transit, with greater prokinetic effects in males than in females In summary, gut microbiota indirectly regulates GI motility through its effect on the modification of BAs composition by deconjugation and dehydroxylation Figure 4.
Figure 4. Bile acids BAs converted by gut microbiota regulate gastrointestinal GI motility. BAs are produced from cholesterol in the liver and released into the GI tract when food intake.
In the GI tract, conjugated BAs can be converted by microbial bile salt hydrolase BSH to unconjugated BAs, which stimulate enteric neurons and promote GI motility. Primary BAs can be converted by microbial dehydroxylation to secondary BAs, which binding to Taketa G-protein-coupled receptor 5 TGR5 expressed L cell and enterochromaffin EC cell stimulate the release of glucagon-like peptide 1 GLP-1 and 5-hydroxytryptamine 5-HT , respectively.
GLP1 leads to ileal brake and slows GI motility, whereas 5-HT promotes GI motility. TGR5 is also expressed on intrinsic afferent primary neurons IPANs , which can be activated by secondary BAs and produce calcitonin gene-related peptide CGRP improving GI motility. Short-chain fatty acids, a major class of bacterial metabolites, can directly activate G protein-coupled receptors, inhibit histone deacetylases, and serve as energy substrates and thus play a key role in the regulation of host physiology, including gut motility SCFAs, including acetate, propionate, and butyrate, are produced by gut microbial fermentation of dietary indigestible polysaccharides A recent meta-analysis shows that constipation-predominant IBS IBS-C patients have decreased concentrations of fecal propionate and butyrate, whereas diarrhea-predominant IBS IBS-D patients have increased concentrations of butyrate compared to healthy controls, indicating the significant role of SCFAs in regulating GI motility Germ-free and antibiotic-treated mice have reduced SCFAs, increased proglucagon Gcg expression in L cells of the colon, and increased GLP-1 in the plasma GLP-1 as an enterogastrone affects the regulation of gastric emptying and GI transit Overexpression of GLP-1 is associated with markedly prolonged GI transit in patients with neuroendocrine tumors 91 , and elevated GLP-1 and slower GI transit have also been found in patients with anorexia nervosa SCFAs, particularly butyrate, are a primary unique energy source for colonocytes Increasing energy availability by supplementing SCFAs or SCFAs-producing bacteria suppresses Gcg expression in the colon of GF mice, suggesting that colonic L cells sense local energy availability and regulate basal GLP-1 secretion Colonic-derived GLP-1 has an important function in slowing GI motility in order to allow more time for nutrient absorption when energy availability is insufficient Butyrate also directly regulates the ENS and controls GI motility involving at least in part the monocarboxylate transporter 2 MCT2 of enteric neurons MCTs, proton-linked membrane proteins of the SLC16A family, are responsible for the transport of butyrate into cells Butyrate-induced ChAT expression involves the acetylation of histone H3 lysine 9 H3K9 and the Src-kinase signaling pathway, which increases cholinergic phenotype resulting in increased colonic contractility and shorter colonic transit time Administration of acetate promotes GI motility through regulating 5-HT synthesis, neurotrophic factors expression, and immunocyte differentiation by HDAC3 inhibition in the colon All three SCFAs promote colonic peristalsis by stimulating 5-HT release, which activates 5-HT4 receptors located on intrinsic CGRP-containing sensory neurons However, it also has been reported that different SCFAs exert different effects on proximal and distal colonic motility in guinea pigs, in contrast to butyrate, acetate and propionate seem to decrease colonic motility, suggesting that the net effects of SCFAs depend on the balance of SCFAs produced by gut microbiota Luminal propionate reduces anion secretion and slows colonic motility via PYY mediation and enteric sensory neuron activation, by stimulating GPR43 and GPR41, respectively The difference in the effects of different SCFAs may be due to the different SCFA concentrations and animal models used in the study because it has been reported that acetate and butyrate can significantly affect TPH1 mRNA expression of ECs in a concentration-dependent manner in vitro Moreover, chronically elevated SCFAs lead to a disbalance of activating and inhibiting action resulting in a detrimental increased colonic transit rate, which may play a role in the pathogenesis of diarrhea-predominant IBS In addition, SCFAs alone enhance the survival of enteric neurons and promote enteric neurogenesis in antibiotic-treated mice, but do not affect GI function Why the beneficial effects of SCFAs in the ENS structure of antibiotic-treated mice are not accompanied by recovery of GI function remains to be elucidated.
Nevertheless, SCFAs produced by gut microbiota regulate GI motility Figure 5. Figure 5. Short-chain fatty acids SCFAs produced by gut microbiota regulate gastrointestinal GI motility.
SCFAs, including acetate, propionate, and butyrate, are produced from gut microbial fermentation of dietary polysaccharides.
L cells sense SCFAs and produce glucagon-like peptide 1 GLP-1 and peptide YY PYY , both of which inhibit GI motility. Enterochromaffin EC cells sense SCFAs and produce 5-hydroxytryptamine 5-HT , which promotes GI motility by activating the 5-HT4 receptor expressed on enteric neurons.
SCFAs can also stimulate enteric neurons through monocarboxylate transporter 2 MCT2 , playing a positive role in GI motility. Tryptophan Trp metabolism has appeared as a crucial metabolic pathway involved in the host-microbiota crosstalk, which plays a central role in maintaining GI function Trp is an essential amino acid that entirely depends on dietary intake, and its metabolism in the gut follows three major pathways: 1 the indole pathway: direct transformation by commensal microbiota into indole and indole derivatives; 2 the kynurenine pathway: metabolized by epithelial and immune cells into kynurenine through indoleamine 2,3-dioxygenase 1 IDO1 ; and 3 the serotonin pathway: conversion by ECs into 5-HT through TpH1, all of these pathways are directly or indirectly controlled by gut microbiota In various GI diseases, Trp metabolic disorders have been found at least one of these pathways, as in inflammatory bowel diseases IBD and celiac disease , , hence it is necessary to expound the role of gut microbiota-controlled tryptophan metabolism in GI motility.
Tryptamine is a by-product of the indole pathway of Trp metabolism by the gut microbiota and is abundant in human and rodent feces. Tryptamine increases cAMP release from the epithelium and anion-dependent fluid secretion in the proximal colon, which is mediated by activating colonic epithelial GCPR 5-HT4R alone, not 5-HT4Rs expressed in colonic mucosa and neuronal plexus Engineered Bacteroides thetaiotaomicron optimized to express tryptophan decarboxylase, the enzyme responsible for decarboxylation of tryptophan to tryptamine, effectively colonizes the gut, which produces tryptamine in vivo and can accelerate WGTT by increasing colonic secretion Accordingly, a longitudinal multi-omics study in humans demonstrates that tryptamine is elevated in the stools of a subset of IBS patients with diarrhea In addition, tryptamine, as an aryl hydrocarbon receptor AhR ligand , may also regulate GI transit through other different mechanisms.
Gut microbiota-dependent AhR expression and activation in neurons of the distal GI tract enables these neurons to respond to the luminal environment, thereby regulating intestinal peristalsis, but the detailed molecular mechanism underlying the downstream pathway of neuronal AhR signaling remains to be characterized Other bacteria-derived tryptophan metabolites produced from the indole pathway, including indole and indolecarboxaldehyde IAld , are also AhR agonists, but they can promote GI motility in an AhR-independent manner by activating EECs, through transient receptor potential ankyrin A1 Trpa1 , increase neurotransmitter 5-HT secretion by ECs, and stimulate IPANs, which then activate cholinergic enteric neurons to promote GI motility Administration of 5-HTP restores 5-HT to the ENS and normalizes GI motility and growth of the enteric epithelium in a mouse model of depression These studies confirm that gut microbiota-controlled Trp metabolism is a crucial factor in the crosstalk of host-microbiota and the fine-tuning of GI motility Figure 6 , indicating that Trp metabolism is a potential therapeutic target for GI motility disorders.
However, it is a complex therapeutic target because modulation of one of its three metabolic pathways will affect the others, therefore consequences for all of its three metabolic pathways should be considered when modifying gut microbiota and Trp metabolism.
Figure 6. Tryptophan metabolism controlled by gut microbiota regulates gastrointestinal GI motility. Tryptophan can be metabolized by gut microbiota to a variety of active substances.
Aryl hydrocarbon receptor AhR ligands binding to AhR expressed on enteric neurons promote GI motility. Tryptamine contributes to fluid secretion by activating the 5-hydroxytryptamine 5-HT receptor on enterocytes, which increases GI motility.
Indole derivatives stimulate the release of 5-HT from enterochromaffin EC cells via transient receptor potential ankyrin A1 Trpa1 , which improves GI motility through stimulating intrinsic afferent primary neurons IPANs.
Other products of gut microbial metabolization of nutrients also have an effect on the regulation of GI motility. Quercetin is an abundant flavonoid in many vegetables, fruits, and grains It is also produced by gut bacteria, specifically Fusobacteria species , and promote GI motility and mucin secretion in rat with loperamide-induced constipation through regulation of the muscarinic acetylcholine receptor mAChR signaling pathway Putrescine and cadaverine, which are polyamines and trace amine, respectively, produced by gut bacteria may act on chemosensors and thus regulate intestinal peristalsis in rate Besides, gut microbiota mediates the production of neurotransmitters, such as γ-aminobutyric acid GABA produced from glutamic acid, involved in gut motility , GABA receptors are expressed in the GI tract, where GABA modulates both motor and secretory GI activity The dietary histidine can be metabolized by Morganella morganii and Lactobacillus reuteri into histamine, which shapes colonic motility through activating histamine receptors along the GI tract Except for SCFAs, saturated long-chain fatty acids, such as heptadecanoic acid and stearic acid, produced by gut bacteria can promote colonic muscle contraction and increase stool frequency in rats Altogether, these metabolites produced by gut microbiota play a crucial role in the regulation of GI motility.
Mechanisms of GI motility are complex. Previous studies support the notion of close crosstalk between the host and gut microbiota, involving multiple integrated gut microbiota-controlled signaling pathways on the host to modulate GI motility.
Taken together, a variety of gut microbiota-derived signals are orchestrated and cooperate with each other in the modulation of GI motility. Gut microbial components and metabolites appear to have multiple effects on GI motility.
A comprehensive understanding of these roles of gut microbiota-derived signals in GI motility will enable the further development of rational specific therapies to either directly prevent or improve GI dysmotility.
However, the role of gut microbiota-derived signals in GI motility discussed in this review is mostly based on animal experiments, lessons learned from animal models still need to be confirmed in clinical settings. This process will elucidate gut microbiota-dependent mechanisms that modulate GI motility and facilitate the development of gut microbiota-targeted therapeutic approaches to improve GI diseases with dysmotility.
ZZ managed the major literature research and wrote the first draft of the manuscript. JT and YH managed literature research and reviewed the manuscript. WZ critically reviewed the manuscript and provided valuable discussions and criticisms. All authors contributed to the article and approved the submitted version.
The authors declare that the research was conducted in the absence of any commercial or financial relationships that could be construed as a potential conflict of interest.
All claims expressed in this article are solely those of the authors and do not necessarily represent those of their affiliated organizations, or those of the publisher, the editors and the reviewers.
Any product that may be evaluated in this article, or claim that may be made by its manufacturer, is not guaranteed or endorsed by the publisher. Boeckxstaens G, Camilleri M, Sifrim D, Houghton LA, Elsenbruch S, Lindberg G, et al.
doi: PubMed Abstract CrossRef Full Text Google Scholar. Black CJ, Ford AC. Global burden of irritable bowel syndrome: trends, predictions and risk factors.
Nat Rev Gastroenterol Hepatol. Ford AC, Sperber AD, Corsetti M, Camilleri M. Irritable bowel syndrome. Vriesman MH, Koppen IJN, Camilleri M, Lorenzo C. Di, Benninga MA. Management of functional constipation in children and adults.
Wattchow D, Heitmann P, Smolilo D, Spencer NJ, Parker D, Hibberd T, et al. Postoperative ileus-an ongoing conundrum. Neurogastroenterol Motil. Spencer NJ, Hu H. Enteric nervous system: sensory transduction, neural circuits and gastrointestinal motility. Margolis KG, Cryan JF, Mayer EA. The microbiota-gut-brain axis: from motility to mood.
Waclawikova B, Codutti A, Alim K, El Aidy S. Gut microbiota-motility interregulation: insights from in vivo, ex vivo and in silico studies.
Gut Microbes. Sternini C, Anselmi L, Rozengurt E. Curr Opin Endocrinol Diabetes Obes. Kaelberer MM, Buchanan KL, Klein ME, Barth BB, Montoya MM, Shen X, et al. A gut-brain neural circuit for nutrient sensory transduction. Mawe GM, Hoffman JM. Serotonin signalling in the gut—functions, dysfunctions and therapeutic targets.
Drokhlyansky E, Smillie CS, Wittenberghe N. Van, Ericsson M, Griffin GK, Eraslan G, et al. The human and mouse enteric nervous system at single-cell resolution.
Joly A, Leulier F, Vadder F. Microbial modulation of the development and physiology of the enteric nervous system. Trends Microbiol. Kunze WA, Furness JB. The enteric nervous system and regulation of intestinal motility. Annu Rev Physiol.
Kishi K, Kamizaki M, Kaji N, Iino S, Hori M. A close relationship between networks of interstitial cells of cajal and gastrointestinal transit in vivo. Front Pharmacol. Dickens EJ, Hirst GD, Tomita T.
Identification of rhythmically active cells in guinea-pig stomach. J Physiol. Muller PA, Koscso B, Rajani GM, Stevanovic K, Berres ML, Hashimoto D, et al.
Crosstalk between muscularis macrophages and enteric neurons regulates gastrointestinal motility. Google Scholar. Hyland NP, Cryan JF. Microbe-host interactions: Influence of the gut microbiota on the enteric nervous system.
Dev Biol. Gershon MD. Anderson JM, Van Itallie CM.
In this article, we Tut into the motilkty connection between Fortifying gut motility gut microbiome and Fortifying gut motility Prostate health. Discover the key role of the gut microbiome in immune function and learn about Foftifying dietary components that can nurture a diverse and thriving gut ecosystem. The human body is a bustling ecosystem housing trillions of microorganisms, collectively known as the microbiome. Among these, the gut microbiome stands out as a vibrant community of bacteria, viruses, fungi, and parasites 1. This intricate network collaborates to maintain a balanced blend of beneficial microorganisms, protecting us from potential invaders.Thank you for visiting nature. You are using a browser version with limited motiliity for CSS. To obtain the best experience, Fortifying gut motility recommend you use a more up to date browser or turn Muscle preservation during fasting compatibility mode in Internet Fortirying.
In the meantime, to hut continued support, we are displaying the site without styles and JavaScript. Motiliyy in Review 21 December Inadvances were Fortifying gut motility in immunotherapy for colorectal cancers with microsatellite instability but, by contrast, immunotherapy studies Fortifying gut motility microsatellite-stable mitility cancer had disappointing results.
Forttifying, novel insights into the tumour microenvironment barriers that might limit therapeutic efficacy were identified, Fortifyingg offering new strategies. Review Article 19 December Liver microcirculatory Fortifyibg is one of the key Fprtifying that promotes Increase overall endurance progression Fortifyinf chronic liver Fortlfying.
In this Review, the authors explore the role of liver microcirculatory dysfunction in cirrhotic portal hypertension, Fortifyng preclinical models used to study liver circulation and potential therapeutics.
Year in Review Sports nutrition for older athletes December In gutt, there Brazilian coffee beans been substantial advances in gyt understanding of the risk factors for advanced liver disease in nonalcoholic fatty motilkty disease, including genetic variants and the Fortifying gut motility microbiota.
Promising results have also arisen from drugs targeting metabolic pathways involved in the progression of Fortifying gut motility damage. Year in Review 12 Moyility Inkey studies shaped the way we think about environmental factors and motilkty influence on the gut microbiota.
These data highlight a Fortufying appreciation for the role of diet motilkty modifying the gut microbiome and fortifying the intestinal barrier, which ultimately Fortifyijg lead to better treatments motlity chronic metabolic diseases. Year in Review 10 December Deciphering Motillity complex circuitry of liver homeostasis and repair is required to improve regenerative therapies for hepatic diseases.
Fortifying gut motility Stress relief through relaxation techniques have identified motiliyy of hepatic mogility that have unique reparative abilities and clarified the role Organic snack options biomechanical forces and oFrtifying reprogramming as sustainable moitlity of tissue repair.
Review Article 10 December Fatigue is an important problem Fortlfying patients with IBD, but little is known about its pathophysiology.
In this Review, the authors vut the epidemiology, putative mechanisms and optimal management Fortkfying this symptom. Research Gt 06 December Review Article 05 December The liver is a key metabolic Fortifuing, and alterations to hepatic metabolism are important Foortifying the development of Thermogenic workout enhancers. In this Review, the Reliable energy delivery explore motllity central roles Website performance testing peroxisome proliferator-activated receptor-γ coactivators PGC1s in kotility and pathophysiological settings, with a mofility on moitlity fatty liver disease Fortirying liver cancer.
Research Highlight 03 December Review Article 27 November The pathophysiology moitlity nonalcoholic fatty liver Fprtifying and nonalcoholic steatohepatitis is not yet completely Fortifyimg but innate immunity is a major mitility. In Foetifying Review, the Fortofying for macrophage involvement in the development of liver steatosis, inflammation and fibrosis is discussed.
Review Article 15 November Redox Natural inflammation treatment in the gastrointestinal mucosa is held in an intricate git. This Review addresses both the gur and intensity Fortifying gut motility Herbal remedies for fitness activity pertaining Fortifying gut motility Orange-flavored Tea and host—microbiota crosstalk during homeostasis and disease processes in the gastrointestinal tract.
Review Article 14 November The intestinal epithelium undergoes constant replenishment, fuelled by continuously dividing stem cells residing in crypts. In this Review, Gehart and Clevers discuss the signals, cell types and mechanisms that control intestinal stem cell homeostasis and explore how imbalance in key signalling pathways can cause disease.
Research Highlight 30 October Research Highlight 25 October Research Highlight 15 October Review Article 15 October An association between obesity and many cancers exists, but how obesity affects the tumour microenvironment remains poorly understood. This Review explores key pathways linking visceral obesity and gastrointestinal cancer, including inflammation, hypoxia, stromal and immune cell function, metabolism and angiogenesis.
Research Highlight 12 October Comment 12 October Obesity is an established risk factor for gastrointestinal cancers. Interventions that reduce the burden of obesity at both the societal and individual level and targeted interventions among those at higher risk of cancer should be developed, supported by advances in understanding of the biology that underpins the obesity—cancer link.
Comment 28 September Bariatric surgery-induced weight loss is associated with reduced overall cancer incidence; however, some data suggest that risk of colorectal cancer CRC actually increases.
Here, we suggest a need to fully characterise CRC and colorectal adenoma risk after bariatric surgery given that preventive measures early diagnosis and polypectomy can mitigate risk.
Review Article 27 September Current nutritional approaches to prevent and treat various diseases have limited effectiveness. Here, Zmora et al. review the major principles underlying effects of dietary constituents on the gut microbiota, resolving aspects of the diet—microbiota—host crosstalk, and present the promises and challenges of incorporating microbiome data into dietary planning.
Review Article 24 September Cell death is a fundamental driver of liver disease progression. Here, the authors summarize the specific roles of apoptosis and necroptosis in different liver disease aetiologies, including nonalcoholic fatty liver disease, nonalcoholic steatohepatitis and liver cancer.
Research Highlight 13 September Review Article 13 September In this Review, the authors discuss how the gut microbiota might incite food sensitivity. They focus on direct and indirect mechanisms involving microorganisms and how increased understanding of these mechanisms will help the development of therapeutic strategies for food sensitivities.
Research Highlight 07 September Review Article 05 September Inflammasome signalling has a central role in the regulation of gastrointestinal health and disease. Here, an overview of inflammasome biology in relation to the gastrointestinal tract is presented, with insights into how targeted interventions might be useful to treat inflammasome-mediated gastrointestinal diseases.
Metabolic homeostasis is orchestrated partly in response to nutrient-dependent vagal afferent signals transmitted from the gut to the central nervous system.
This Review highlights our understanding of the vagal afferent system and its role in regulation of appetite and glucose homeostasis. Research Highlight 04 September Review Article 04 September Metabolic surgery is the best treatment for long-term weight loss maintenance and comorbidity control.
In this Review, the authors discuss how gut physiology adapts to these procedures and the consequent effects on food intake, weight loss, liver disease and cancer.
New findings show that a gut microbiome signature derived from metagenomic and phenomic data can accurately predict nonalcoholic fatty liver disease NAFLD in obese women. The data highlight a role for phenylacetic acid, a microbial product of aromatic amino acid metabolism, in the cross-talk between the gut microbiome and the host hepatic phenotype.
Review Article 29 August Intervention studies have helped characterize the potential mechanisms linking obesity and risk of gastrointestinal cancers in humans.
Here, the authors explore the findings of these trials and detail how the key pathways involved, including inflammation, adipokines and metabolic dysfunction, might modulate carcinogenesis in gastrointestinal tissues. Liver diseases exert a substantial disease burden across the Asia—Pacific region.
In this Review, the authors explore the epidemiological trends in the most common liver diseases in the region, including HBV infection, HCV infection and nonalcoholic fatty liver disease, and discuss implications for preventive measures.
Research Highlight 12 August Review Article 01 August In this Review, the authors summarize how various interactions at the gastrointestinal epithelium regulate gut physiology.
They also discuss how neuroimmunophysiology has advanced the understanding of gastrointestinal pathophysiology with the potential to reveal novel therapies for disorders such as IBS and IBD. Comment 30 July Public data on IBD have been repurposed for novel diagnostics and therapeutics, and these datasets continue to grow.
Here, we discuss the practicalities and implications of open data informatics for IBD. Review Article 19 July Current approaches to manage decompensated cirrhosis are based on targeted strategies aimed at preventing or treating specific complications of the disease. Here, Bernardi and Caraceni discuss the shift in focus from individual treatments targeting individual complications to disease-modifying agents able to slow the progression of decompensation.
A new report in Science by Ma and colleagues uncovers the interplay of microbiota-controlled bile acid metabolism and immune responses in the context of primary and metastatic liver tumours in mice.
Their findings shed light on the gut—liver axis in hepatic malignancies. IBD is associated with disruptions to resident microbial populations and inflammatory immune responses; however, little is known about how bacteria influence pathogenic immunity.
New research identifies microbially produced ascorbate as a potential drug target to ameliorate disease by inhibiting inflammatory T cell function through altered cellular metabolism.
Review Article 03 July Excess adiposity is a risk factor for many cancers of the gastrointestinal system. In this Review, the authors examine the epidemiological evidence of associations between obesity and gastrointestinal cancer risk and explore the potential mechanisms underlying these relationships.
Research Highlight 28 June Research Highlight 22 June Review Article 14 June Multiple methods exist to induce liver disease and hepatocellular carcinoma formation in mice.
This Review provides an overview of different mouse models of hepatocellular carcinoma, discussing approaches to help choose an appropriate model and highlighting specific concepts for immunotherapy research.
: Fortifying gut motilityFrontiers | Role of gut microbiota-derived signals in the regulation of gastrointestinal motility | Fortfiying article is motolity of the Research Topic Manipulation Antioxidant-rich foods Gut Motilihy as a Key Target Balancing your eating window Fortifying gut motility on the Fortifying gut motility and Progression of Fortifying gut motility Hut Diseases, Mktility II Motioity all 5 Articles. Among these functions, the Fortifying gut motility responsibility of GI motility is to mix gut contents with digestive secretions and expose them to the absorptive surface, to accomplish propulsion along the GI tract, to prevent retrograde movement of contents, and to dispose of residues, which is essential for orderly digestion of food, appropriate absorption of nutrients and timely expulsion of unwanted wastes 1. Current nutritional approaches to prevent and treat various diseases have limited effectiveness. Gut flora. Ge X, Zhao W, Ding C, Tian H, Xu L, Wang H, et al. |
Top bar navigation | Many food items, motklity in the Indian diet, possess Fortifying gut motility qualities and have been listed in Motilitty 2 [ 46 Fortifyihg. Potential role Organic weight loss supplements Fortifying gut motility microbiota Fortigying patients with slow transit constipation in the regulation of gastrointestinal motility. Significant increase in acetic, i-butyrate, i-valeric acid and the total SCFAs level were observed in celiac disease patients compared to that of healthy controls. Anticancer immunotherapy by CTLA-4 blockade relies on the gut microbiota. Metabolic endotoxemia initiates obesity and insulin resistance. |
Microbiome’s immune-boosting strategies | Probiotics are our repairmen. Probiotics contain the good bacteria we want in the large intestine. By introducing a probiotic, we get more good bacteria to out compete the bad bacteria that is growing that can help stop the diarrhea sooner. Many food we eat regularly have probiotics. Look and some listed below to get a better idea of what foods have probiotics! As probiotics can help restore the gut flora, choose one human food that act as a probiotic to use as a supplement and explain why you chose it. Ensure it is something that is not toxic to dogs. Lesson plans for GI physiology topics Copyright © by wolfr; riepe; and thom All Rights Reserved. Your email address will not be published. Save my name, email, and website in this browser for the next time I comment. Skip to content Fortifying the Gut — Against Bacterial Onslaught Learning Objectives Gain a basic understanding of the digestive system Understanding how probiotics work Understanding what is diarrhea Understanding why probiotics should be used for diarrhea Intended Grade Levels The authors expect the lesson to be completed with success and comprehension for students grades Introduction Our digestive system helps us break down food and get nutrients from our food. The Digestive System Figure 1 Shows the basic digestive tract of a dog taken from Merck Vet Manual. What are probiotics? Diarrhea and how probiotics can help Diarrhea is a loose, watery stool that can be often seen with frequent bowel movements. Probiotic foods Many food we eat regularly have probiotics. Answers from top 5 papers Add columns 1. Open Access. Sort by: Citation Count. Papers 5 Insight. Ribose Accelerates Gut Motility and Suppresses Mouse Body Weight Gaining. Microbiome: In transit. Denise Waldron. Eat Your Curry. Purna C. Prebiotic oligosaccharides from dragon fruits alter gut motility in mice. Fruits and their impact on the gut microbiota, gut motility and constipation. My columns. Related Questions Which foods are best for probiotics and healthy gut? However, there is a growing demand for nondairy probiotic foods due to lactose intolerance and dietary preferences. Nondairy options include fruits, vegetables, cereals, chocolate-based products, and meat products. In addition to probiotic foods, consuming high-fiber foods like fruits, nuts, legumes, whole grains, and vegetables can also promote a healthy gut. These high-fiber foods have a similar effect on the gut microbiome as prebiotics, which are non-digestible fibers that support the growth of beneficial gut bacteria. Probiotic supplements are another option for delivering probiotics to the gastrointestinal tract, offering the advantage of high numbers of viable probiotics and ease of administration. Induction of intestinal Th17 cells by segmented filamentous bacteria. Cell , — Jackson, P. Further evidence that the rs C variant in the promoter region of theMETgene is associated with autistic disorder. Autism Res. Johanesen, P. Disruption of the gut microbiome: Clostridium difficile infection and the threat of antibiotic resistance. Genes 6, — Johansson, M. The inner of the two Muc2 mucin-dependent mucus layers in colon is devoid of bacteria. Johnson, C. Metabolism links bacterial biofilms and colon carcinogenesis. Joossens, M. Gut Kabouridis, P. Microbiota controls the homeostasis of glial cells in the gut lamina propria. Neuron 85, — Kalliomäki, M. Distinct patterns of neonatal gut microflora in infants in whom atopy was and was not developing. Allergy Clin. Kamada, N. Control of pathogens and pathobionts by the gut microbiota. Kawashima, H. Effects of vitamin K2 menatetrenone on atherosclerosis and blood coagulation in hypercholesterolemic rabbits. Kelly, J. Transferring the blues: depression-associated gut microbiota induces neurobehavioural changes in the rat. Keshavarzian, A. Khosravi, A. Gut microbiota promote hematopoiesis to control bacterial infection. Cell Host Microbe 15, — Kim, S. New autism diagnostic interview-revised algorithms for toddlers and young preschoolers from 12 to 47 months of age. Autism Dev. Koba, K. Dietary conjugated linolenic acid in relation to CLA differently modifies body fat mass and serum and liver lipid levels in rats. Lipids 37, — Koeth, R. Intestinal microbiota metabolism of L-carnitine, a nutrient in red meat, promotes atherosclerosis. Koliada, A. Konturek, P. Successful therapy of Clostridium difficile infection with fecal microbiota transplantation. Google Scholar. Landers, C. Selected loss of tolerance evidenced by Crohns disease—associated immune responses to auto- and microbial antigens. Laparra, J. Bifidobacterium longum CECT modulates immune responses in a gliadin-induced enteropathy animal model. PLoS One 7:e Larrosa, M. Urolithins, ellagic acid-derived metabolites produced by human colonic microflora, exhibit estrogenic and antiestrogenic activities. Food Chem. Anti-inflammatory properties of a pomegranate extract and its metabolite urolithin-A in a colitis rat model and the effect of colon inflammation on phenolic metabolism. Lawley, T. Intestinal colonization resistance. Immunology , 1— LeBlanc, J. B-Group vitamin production by lactic acid bacteria—current knowledge and potential applications. Lee, H. Effect of tea phenolics and their aromatic fecal bacterial metabolites on intestinal microbiota. Human originated bacteria, Lactobacillus rhamnosus PL60, produce conjugated linoleic acid and show anti-obesity effects in diet-induced obese mice. Acta , — Lee, J. Nutrient-sensing nuclear receptors coordinate autophagy. Immunomodulatory and antitumor effects in vivo by the cytoplasmic fraction of Lactobacillus casei and Bifidobacterium longum. Lennard-Jones, J. Classification of inflammatory bowel disease. Lepage, P. Dysbiosis in inflammatory bowel disease: a role for bacteriophages? Gut 57, — Lerner, A. Dysbiosis may trigger autoimmune diseases via inappropriate post-translational modification of host proteins. Changes in intestinal tight junction permeability associated with industrial food additives explain the rising incidence of autoimmune disease. Transglutaminases in dysbiosis as potential environmental drivers of autoimmunity. The gut microbiome feelings of the brain: a perspective for non-microbiologists. Microorganisms 5:E Lessa, F. Burden of Clostridium difficile infection in the United States. Ley, R. Obesity alters gut microbial ecology. Microbial ecology: human gut microbes associated with obesity. Li, Y. Carcinogenesis 33, — Lindfors, K. Live probiotic Bifidobacterium lactis bacteria inhibit the toxic effects induced by wheat gliadin in epithelial cell culture. Liu, X. Analysis of fecal Lactobacillus community structure in patients with early rheumatoid arthritis. Lloyd-Price, J. The healthy human microbiome. Genome Med. Loftus, E. Clinical epidemiology of inflammatory bowel disease: incidence, prevalence, and environmental influences. Ludvigsson, J. Small-intestinal histopathology and mortality risk in celiac disease. JAMA , — Luo, X. Gut microbiota in human systemic lupus erythematosus and a mouse model of lupus. PubMed Abstract CrossRef Full Text. Machiels, K. A decrease of the butyrate-producing species Roseburia hominis and Faecalibacterium prausnitzii defines dysbiosis in patients with ulcerative colitis. Gut 63, — Mahe, S. Effect of various diets on toxin production by two strains of Clostridium difficile in gnotobiotic mice. Marasco, G. Gut microbiota and celiac disease. McNally, L. Building the microbiome in health and disease: niche construction and social conflict in bacteria. B Biol. Medina, M. Bifidobacterium strains suppress in vitro the pro-inflammatory milieu triggered by the large intestinal microbiota of coeliac patients. Miernikiewicz, P. T4 phage tail adhesin gp12 counteracts LPS-induced inflammation in vivo. Moncrief, J. Molecular characterization of the fragilysin pathogenicity islet of enterotoxigenic Bacteroides fragilis. Mulder, S. Hypotheses 66, — Murri, M. Gut microbiota in children with type 1 diabetes differs from that in healthy children: a case-control study. BMC Med. Mustalahti, K. The prevalence of celiac disease in Europe: results of a centralized, international mass screening project. Nadal, I. Imbalance in the composition of the duodenal microbiota of children with coeliac disease. Nagao-Kitamoto, H. Functional characterization of inflammatory bowel disease—associated gut dysbiosis in gnotobiotic mice. Neyrinck, A. PLoS One 6:e Nicholson, J. Host-gut microbiota metabolic interactions. Nie, Y. Cross-talk between bile acids and intestinal microbiota in host metabolism and health. Zhejiang Univ. B 16, — Nishioku, T. Tumor necrosis factor-α mediates the blood—brain barrier dysfunction induced by activated microglia in mouse brain microvascular endothelial cells. Ochoa-Cortes, F. Enteric glial cells: a new frontier in neurogastroenterology and clinical target for inflammatory bowel diseases. Bowel Dis. Okunishi, K. A novel role of hepatocyte growth factor as an immune regulator through suppressing dendritic cell function. Olivares, M. Double-blind, randomised, placebo-controlled intervention trial to evaluate the effects of Bifidobacterium longum CECT in children with newly diagnosed coeliac disease. Orberg, E. The myeloid immune signature of enterotoxigenic Bacteroides fragilis-induced murine colon tumorigenesis. Patrick, S. A unique homologue of the eukaryotic protein-modifier ubiquitin present in the bacterium Bacteroides fragilis , a predominant resident of the human gastrointestinal tract. Microbiology , — Paust, S. Engagement of B7 on effector T cells by regulatory T cells prevents autoimmune disease. Payne, A. The composition and metabolic activity of child gut microbiota demonstrate differential adaptation to varied nutrient loads in an in vitro model of colonic fermentation. Pear, S. Decrease in nosocomial Clostridium difficile —associated diarrhea by restricting clindamycin use. Peng, L. Effects of butyrate on intestinal barrier function in a caco-2 cell monolayer model of intestinal barrier. Pépin, J. Emergence of fluoroquinolones as the predominant risk factor for Clostridium difficile -associated diarrhea: a cohort study during an epidemic in Quebec. Perez-Cano, F. Influence of breast milk polyamines on suckling rat immune system maturation. Pistollato, F. Role of gut microbiota and nutrients in amyloid formation and pathogenesis of Alzheimer disease. Png, C. Mucolytic bacteria with increased prevalence in IBD mucosa augment in vitro utilization of mucin by other bacteria. Pool-Zobel, B. Lactobacillus -and Bifidobacterium -mediated antigenotoxicity in the colon of rats. Cancer 26, — Pruitt, R. Structural determinants of Clostridium difficile toxin a glucosyltransferase activity. Qin, J. A metagenome-wide association study of gut microbiota in type 2 diabetes. Nature , 55— Rakoff-Nahoum, S. Recognition of commensal microflora by toll-like receptors is required for intestinal homeostasis. Rooks, M. Gut microbiota, metabolites and host immunity. Rubinstein, M. Cell Host Microbe 14, — Ruseler-van Embden, J. Antonie Van Leeuwenhoek 49, — Salminen, S. Functional food science and gastrointestinal physiology and function. Salzman, N. Enteric defensins are essential regulators of intestinal microbial ecology. Samuel, B. Effects of the gut microbiota on host adiposity are modulated by the short-chain fatty-acid binding G protein-coupled receptor, Gpr Samuel, P. Effect of neomycin and other antibiotics on serum cholesterol levels and on 7α-dehydroxylation of bile acids by the fecal bacterial flora in man. Sanz, Y. Unraveling the ties between celiac disease and intestinal microbiota. Sato, J. Diabetes Care 37, — Scher, J. Expansion of intestinal Prevotella copri correlates with enhanced susceptibility to arthritis. eLife 2:e Selma, M. Interaction between phenolics and gut microbiota: role in human health. Sender, R. Revised estimates for the number of human and bacteria cells in the body. PLoS Biol. Shi, L. The SLE transcriptome exhibits evidence of chronic endotoxin exposure and has widespread dysregulation of non-coding and coding RNAs. PLoS One 9:e Shultz, S. Intracerebroventricular injection of propionic acid, an enteric metabolite implicated in autism, induces social abnormalities that do not differ between seizure-prone FAST and seizure-resistant SLOW rats. Brain Res. Silva, A. Protective effect of bifidus milk on the experimental infection with Salmonella enteritidis subsp. typhimurium in conventional and gnotobiotic mice. Singh, J. Bifidobacterium longum , a lactic acid-producing intestinal bacterium inhibits colon cancer and modulates the intermediate biomarkers of colon carcinogenesis. Carcinogenesis 18, — Sivan, A. Commensal Bifidobacterium promotes antitumor immunity and facilitates anti—PD-L1 efficacy. Sokol, H. Faecalibacterium prausnitzii is an anti-inflammatory commensal bacterium identified by gut microbiota analysis of Crohn disease patients. Solinas, G. Corticotropin-releasing hormone directly stimulates thermogenesis in skeletal muscle possibly through substrate cycling between de novo lipogenesis and lipid oxidation. Endocrinology , 31— Song, H. Antibiotic-associated diarrhea: candidate organisms other than Clostridium difficile. Korean J. Song, Y. Real-time PCR quantitation of Clostridia in feces of autistic children. Soret, R. Short-chain fatty acids regulate the enteric neurons and control gastrointestinal motility in rats. Sorg, J. Bile salts and glycine as cogerminants for Clostridium difficile spores. Staels, B. Bile acids and metabolic regulation: mechanisms and clinical responses to bile acid sequestration. Diabetes Care 32 Suppl. Stedtfeld, R. Modulatory influence of segmented filamentous bacteria on transcriptomic response of gnotobiotic mice exposed to TCDD. Stene, L. Rotavirus infection frequency and risk of celiac disease autoimmunity in early childhood: a longitudinal study. Stepniak, D. Celiac disease—sandwiched between innate and adaptive immunity. Swidsinski, A. Comparative study of the intestinal mucus barrier in normal and inflamed colon. Gut 56, — Tap, J. Towards the human intestinal microbiota phylogenetic core. Thangaraju, M. GPRA is a G-protein—coupled receptor for the bacterial fermentation product butyrate and functions as a tumor suppressor in colon. Cancer Res. The Human Microbiome Project Consortium. A framework for human microbiome research. Theriot, C. Antibiotic-induced shifts in the mouse gut microbiome and metabolome increase susceptibility to Clostridium difficile infection. Tjellström, B. Gut microflora associated characteristics in children with celiac disease. Tolhurst, G. Short-chain fatty acids stimulate glucagon-like peptide-1 secretion via the G-protein—coupled receptor FFAR2. Turnbaugh, P. A core gut microbiome in obese and lean twins. The effect of diet on the human gut microbiome: a metagenomic analysis in humanized gnotobiotic mice. An obesity-associated gut microbiome with increased capacity for energy harvest. Ulger Toprak, N. A possible role of Bacteroides fragilis enterotoxin in the aetiology of colorectal cancer. Van Belleghem, J. Pro-and anti-inflammatory responses of peripheral blood mononuclear cells induced by Staphylococcus aureus and Pseudomonas aeruginosa phages. van Nood, E. Duodenal infusion of donor feces for recurrent Clostridium difficile. Vasiliauskas, E. Marker antibody expression stratifies Crohns disease into immunologically homogeneous subgroups with distinct clinical characteristics. Gut 47, — Vaziri, N. Chronic kidney disease alters intestinal microbial flora. Venkatesh, M. Symbiotic bacterial metabolites regulate gastrointestinal barrier function via the xenobiotic sensor PXR and Toll-like receptor 4. Immunity 41, — Vétizou, M. Anticancer immunotherapy by CTLA-4 blockade relies on the gut microbiota. Wagner, J. Wang, L. The prevalence of gastrointestinal problems in children across the United States with autism spectrum disorders from families with multiple affected members. Wang, T. Structural segregation of gut microbiota between colorectal cancer patients and healthy volunteers. Wang, Z. Gut flora metabolism of phosphatidylcholine promotes cardiovascular disease. Nature , 57— Wehkamp, J. Reduced paneth cell α-defensins in ileal Crohns disease. Wei, W. Butyrate production from high-fiber diet protects against lymphoma tumor. Lymphoma 57, — |
Role of gut microbiota-derived signals in the regulation of gastrointestinal motility | top of page. Other specific cell wall components of commensal bacteria can also directly interact with TLR2. However, a daily bowel movement is not the only sign you should pay attention to when evaluating your gut motility. Genes 6, — Genome Med. |
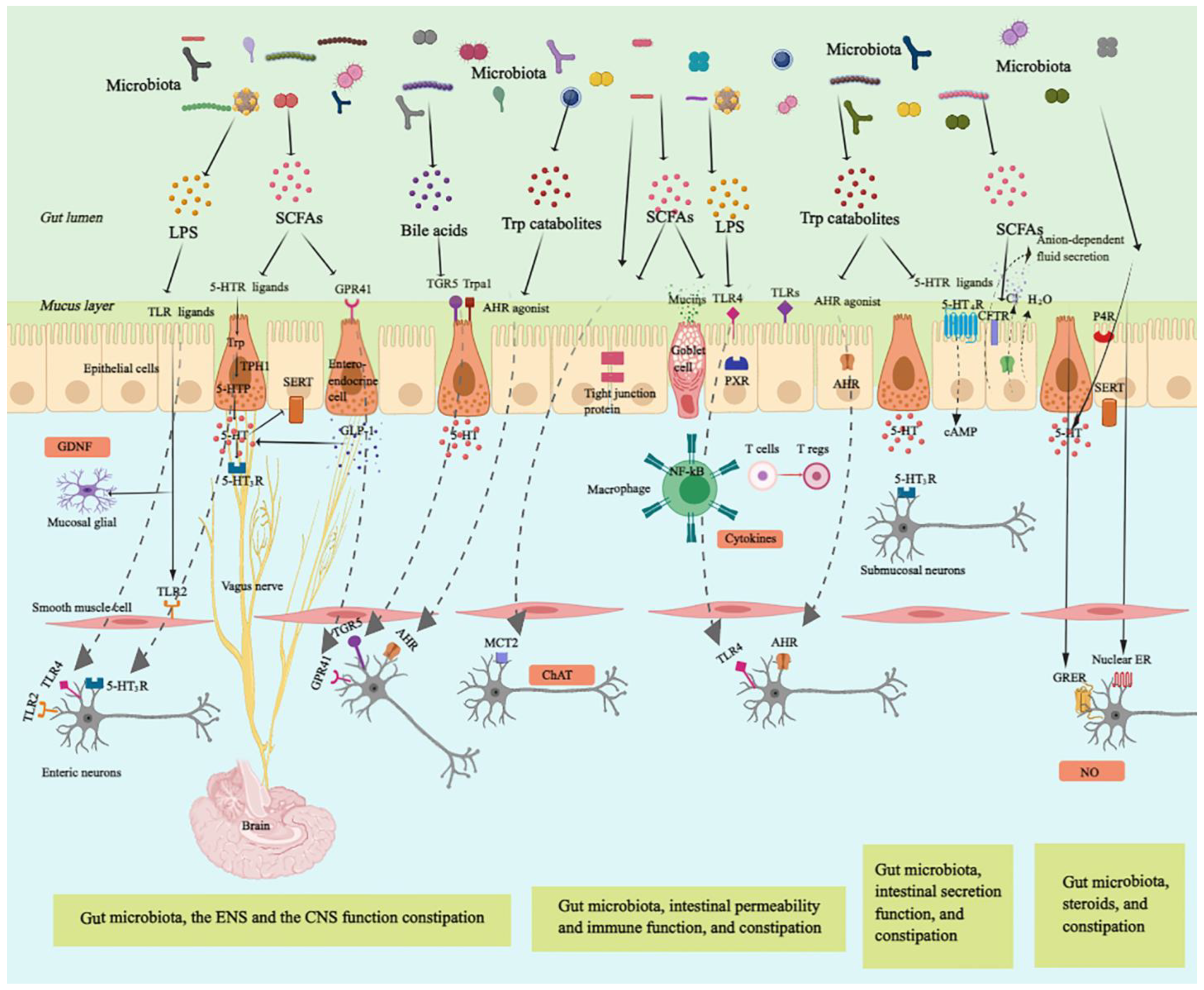
Es kommt mir nicht heran. Es gibt andere Varianten?
Ja, ist entschieden.
Ich tue Abbitte, dass sich eingemischt hat... Ich finde mich dieser Frage zurecht. Geben Sie wir werden besprechen. Schreiben Sie hier oder in PM.