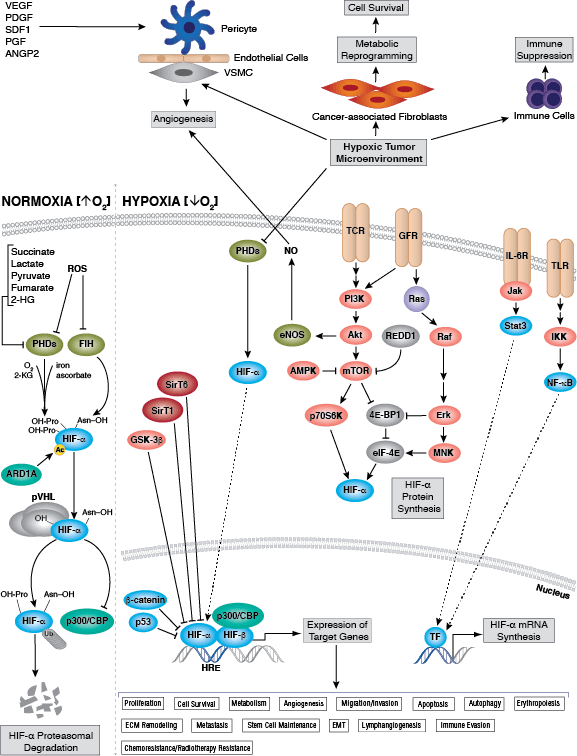
Carbohydrate metabolism and cell signaling -
Schizophrenia is a chronic and severe mental disorder that affects over 20 million people worldwide. Common symptoms include distortions in thinking, perception, emotions, language, and self awareness.
Different hypotheses have been proposed to explain the development of schizophrenia, however, there are no unifying features between the proposed hypotheses.
Schizophrenic patients have perturbed levels of glucose in their cerebrospinal fluid, indicating a disturbance in glucose metabolism. We have explored the possibility that disturbances in glucose metabolism can be a general mechanism for predisposition and manifestation of the disease.
We discuss glucose metabolism as a network of signaling pathways. Glucose and glucose metabolites can have diverse actions as signaling molecules, such as regulation of transcription factors, hormone and cytokine secretion and activation of neuronal cells, such as microglia.
The presented model challenges well-established concepts in enzyme kinetics and glucose metabolism. Interestingly, environmental insults are common tools used in animal models of fear conditioning or aversive training [ 4 ].
Recently, Rowland et al. However, they interpreted their novel data using the didactic concept of glucose metabolism that dominates many scientific hypotheses. Here, we integrate the data of Rowland et al. We have zoomed in on her sub-cellular map to rearrange the well-established concept of glucose metabolism into a concept of dynamic cell—cell signaling.
In the first part of our model, we softly tread the path of glucose in the brain. The second part of our model is much rougher, akin to white water rafting and integrates the dual flow of streams of monocarboxylates.
As scientists, more than a few times in life, we have learnt the pathway of glucose metabolism by rota. But, have the concepts that you have learned changed over the past few decades?
When considering this question, bear in mind, that approximately every 9 years the amount of scientific publications double and that glucose metabolism is a scientific field [ 7 ].
Alone, the section dealing with the intermediate metabolism of carbohydrates, lipids, steroids, amino acids and proteins, nucleic acids and porphyrin and haem compounds totaled pages [ 9 ]. If we apply the same rationale, the section relating to glucose metabolism must now be worth an extraordinary number of pages, unless nothing has changed in the past 60 years in biology.
The belief that nothing has changed and nature has kindly sorted the glycolytic enzymes by the gradual degradation of the carbon backbone can help us to learn the names of the enzymes, products, and substrates.
However, it is also one way to avoid the new data presented in this exponential increase in publications. Consider the enzyme, glyceraldehyde 3-phosphate dehydrogenase GAPDH. In biology, glycolysis is known to participate in DNA repair and transcription factor regulation [ 10 ], mitochondrial membrane permeability, and apoptosis [ 11 ].
Accordingly, it is a matter of definition whether one considers GAPDH as a multifunctional protein with functions distinct from glycolysis, or whether all functions performed by GAPDH relate to glycolysis [ 13 ]. One of the consequences of this flood of information is that scientists tend to specialize in small and distinct scientific fields.
Fatally, this leads to the repetition of the didactically based concept of glucose metabolism in all scientific manuscripts.
Alternatively, more complicated cytosolic metabolic steps can be introduced. For example, in the textbook of Rapoport from , GAPDH is functionally linked with lactate dehydrogenase LDH [ 14 ].
In modern didactic biology, LDH does not exist because LDH is only introduced when obligate aerobic organisms start to ferment glucose to lactic acid l -lacH. A similar process is well known for facultative aerobic yeast fermenting glucose to ethanol.
Logically, as the scientific field of glucose metabolism grows exponentially, the didactically based concept of glucose metabolism must decline.
Learning is like rowing against the current. As soon as you stop, you drift backwards. The well-established concept of glucose metabolism starts by learning names of a dozen enzymes arranged by the graded degradation of the carbon backbone. It ends by drawing a circle around these enzymes and naming it the blueprint of life.
Scientists have been rowing the current concept of glucose metabolism up-stream for centuries. Here we test the hypothesis that learning is like floating the path scientists have rowed. Here, when neurons fire and consume fuel, the reverberatory current is the flow of glucose and glucose metabolites.
These reverberatory currents induce lasting cellular changes that add to the stability of the current [ 18 ]. Put simply, synapses with high-energy turnover stabilize themselves. Let us assume the fuel of neurons is a mixture of l -lacH, pyruvic acid pyrH , and glucose.
This dynamic sending export and receiving import of monocarboxylates modulates pH- and redox-sensitive signaling pathways [ 19 ]. It is well established that glucose itself directly triggers the release of insulin from pancreatic β-cells [ 22 ].
Consequently, glucose metabolism is a signaling pathway and glucose and glucose metabolites are signaling molecules. Furthermore, glycogen granules represent storage vesicles of the signaling molecules, glucosephosphate and glucose.
Importantly, memory formation is functionally linked to astrocytic glycogenolysis [ 4 ]. In astrocytes, muscle cells and thrombocytes the catabolic breakdown of glycogen is the preferred route of metabolism. Consider the glucose concentrations in the brain.
In , Gjedden et al. revealed that the concentration of glucose in brain interstitial fluid ISF is low 2. More recent data showing that ISF and cerebrospinal fluid are permanently exchanged, support this [ 24 ].
The low glucose concentration of the ISF strongly suggests that neurons do not acquire their glucose from the ISF. Microscopic data demonstrate that blood capillaries 5. Furthermore, neurons themselves are not completely covered by astrocytes. Thus astrocytes, through direct and specific contacts with neurons, act as pipeline of glucose to neuronal compartments of high-energy demand [ 26 ].
The concentration of glucose in the interstitial fluid is low 2. Astrocytic endfeet completely cover capillaries, where the concentration of glucose is high 5. Glucose is transported via glucose transporters GLUTs from the blood stream, across the astrocyte and delivered to neuronal compartments of high-energy demand via astrocytic and neuronal GLUTs.
Astrocytic glycogenolysis is a signaling pathway producing glucose, which enters the glucose transit pathway and glucosephosphate.
The latter is converted to glucosephosphate, which inhibits hexokinase II [ 73 ], thereby blocking astrocytic glycolysis. Decreased astrocytic glycolysis further increases the availability of glucose for neuronal transit.
The first glucose transporter GLUT was cloned in [ 27 ]. This discovery places GLUTs, rather than hexokinase II as the first regulatory enzyme of glycolysis. In the context of schizophrenia, the critical passage is the astrocyte-neuron compartment and it is stabilization of astrocyte-neuron compartments that is key.
It is obvious, that cell-adhesion proteins must also play a key role in glucose flow [ 28 ]. Mechanisms stabilizing the cell—cell compartment, such as mechanisms that accelerate glucose flow are directly involved in regulating the stability of the astrocyte-neuron compartment [ 29 ].
Mechanisms that detect an incompletely sealed compartment and mechanisms involved in pruning, brain plasticity, and brain development are also involved in regulating the stability of astrocyte-neuron compartments [ 29 ].
Interestingly, many of the listed risk genes and biomarker candidates of schizophrenia are functionally and directly connected to the dynamics of this cell-to-cell passage of glucose [ 1 ]. Memory consolidation is functionally coupled to the upregulation of mRNA for proton-linked monocarboxylate transporter 1 MCT1 , muscle LDH LDH-m , and heart LDH LDH-h , astrocytic and neuronal GLUTs and astrocytic proton-linked MCT4 [ 30 ].
Only the neuronal proton-linked MCT2 remains unchanged [ 30 ]. If we understand schizophrenia as an imbalance in glucose metabolism and glucose metabolism is the signaling pathway that dynamically regulates the stability of the astrocyte-neuron compartment.
Then it is rational to suggest that an imbalance in glucose metabolism can be linked to dysregulated astrocyte-neuron compartments. A dysregulated astrocyte-neuron compartment can leak the signaling molecule glucose, into the ISF and activate surrounding microglial cells.
Glucose-activated microglial cells upregulate inflammatory proteins and increase phagocytosis and tumor necrosis factor-alpha secretion [ 31 ]. Interestingly, glucose deprivation sensitizes microglia, promoting the release of inflammatory mediators, and primes microglial function [ 31 ].
As discussed by Stöber and co-workers, the release of other biomarker candidates, such as interleukin-1β IL-1β , IL-6 and brain-derived neurotrophic factor are also directly triggered by glucose or its metabolites [ 1 , 32 ]. The opening of a bottle of champagne releases a force powerful enough to propel the cork to the ceiling and beyond, a bit like releasing a mysterious Djinn.
This is in contrast to the work of Meyerhof, which determined that one molecule of glucose is metabolized to two molecules of l -lacH C 3 H 6 O 3 [ 37 ]. The answer to this conundrum must be that one of the well-established glycolytic reactions is incorrect and that one of the intermediate products of the glycolytic pathway must be an organic acid.
If this continuously formed acid is located in the immediate proximity of a proton-linked MCT, a pH gradient or flow of energy will be created. This flow of energy will be sufficient to unidirectionally drive the export of monocarboxylic acids Fig.
Importantly this export would be independent from the pH and monocarboxylate concentration of the cytosol and environment. It would, however, depend on glycolytic flow. Unexported monocarboxylic acids would not only increase cytosolic monocarboxylate, they would also generate an additional signal in the form of a proton.
This proton would be detected by pH-dependent metabolic adenylyl cyclase and directly couple the glycolysis rate to cAMP-dependent signaling pathways [ 38 ].
In this situation, the well-established concept of enzyme kinetics, based on reversibly acting enzymes collapses. a Glucose, taken up via glucose transporters GLUTs and produced by the breakdown of glycogen glycogenolysis is exported as a signal via GLUTs. However, proton-linked MCTs actually catalyze the membrane exchange of monocarboxylic acids pyrH and l -lacH.
Moreover, by changing the concept of enzyme kinetics, proton-linked MCTs no longer drive equilibrium reactions. Mitochondrial LDH activity was first suggested by Dianzani in [ 41 ] and later, LDH-h was localized to mitochondria [ 42 ]. From —, L.
Thus, for generations, we have learned alchemy, a concept based on faith and not the law of mass conservation. Our tentative sorting of glycolytic enzymes is helpful for the astrocyte-neuron lactate shuttle hypothesis [ 48 ].
In contrast, LDH-m acts as a proton acceptor in enzyme complexes. The distinction between pyrH and lacH is made clear in the animal model of exercise-induced hyperinsulinism.
Here, the proton-linked MCT1 uptake of pyrH, but not l -lacH, triggers insulin release by pancreatic β-cells [ 22 ]. Fear conditioning experiments performed using animal models of learning [ 4 ] and electroshock therapy, first described in by Wesley [ 51 ], have one thing in common.
They both trigger glycogenolysis. Now, by simply changing the point of view and considering the biological function of glycogen granules as a storage of metabolic signaling molecules and cross-referencing with the fact that the scientific field of learning and behavior acknowledges astrocytic glycogenolysis as a key mechanism.
We can de-mystify ECT as a mechanism for the nonspecific activation of a signaling pathway; astrocytic glycogenolysis. Let us consider ECT and sodium-serotonin reuptake inhibitors SSRIs.
Furthermore, SSRIs impair thrombocyte hemostatic responses, with bleeding a side effect of SSRI medication. Therefore, it is logical to assume that the depletion of the glycogen pool indicates an imbalance in the metabolic balancing between anabolic and catabolic pathways, in favor of catabolic pathways.
There is evidence that SSRIs stimulate astrocytic glycogenolysis [ 53 , 54 ]. The increase in astrocytic glycogenolysis is a signal that can be conveyed to neurons via supply of glucose and a change in the transfer of monocarboxylates i. As neurons do not have glycogen stores, they cannot respond to SSRIs by increasing glycogen breakdown.
However, although the astrocytic signal impacts the stability and glucose consumption of astrocyte-neuron compartments, SSRIs are only likely to increase presynaptic glucose consumption.
This suggests a mechanism for the actions of SSRIs in the treatment of schizophrenia patients [ 55 ]. In an organism, the dynamic exchange of the signaling molecules, glucose, pyrH, and l -lacH, allows certain cells, such as muscle and brain cells to adapt or to learn from increased metabolite provision.
Pancreatic β-cells participate in detection and regulation, and other cells such as adipocytes, act as storage. It is well known that antipsychotics, such as olanzapine, have a huge impact on glucose homeostasis in humans. Lynch and co-workers determined the transcriptional changes induced by olanzapine treatment on genes related to the metabolism of glucose in rats [ 56 ].
This signal encodes the postulated stabilization of the astrocyte-neuron compartment and increases activity of LDH-h complexes. Hereby, astrocytes and neurons learn to consume more glucose by stabilizing the astrocyte-neuron compartment and upregulation of GLUTs [ 30 ].
We interpret the data of Tadi and co-workers that both astrocytes and neurons adapt their cell homeostasis to a higher glucose turnover. Thus, an increase in monocarboxylic acid transfer, here interpreted as astrocyte-neuron communication [ 39 ], should entail the upregulation of neuronal proton-linked MCT2, but not the upregulation of neuronal GLUTs.
The decrease in Hk2 hexokinase II in pancreatic β-cells would result in an impaired detection of glucose, giving rise to hyperglycemia [ 58 ]. Together, this shift may be responsible for the weight gain in olanzapine-treated patients [ 60 ].
The impact of olanzapine on metabolism was brilliantly shown by the fact that the switch to fatty acid metabolism is required for survival [ 61 ]. By drawing on a wealth of information taken from bench to bedside. The presented concept of glucose metabolism fits well as a unifying hypothesis of schizophrenia and explains the observed effects of ECT and pharmacological medication.
The review provides a simple, biologically based hypothesis of schizophrenia. It is sufficient to integrate most aspects of schizophrenia including treatment with ECT or olanzapine. We have not looked forward and examined the signaling pathways activated by biomarker candidates of schizophrenia, such as tumor necrosis factor-alpha, IL-1β, and IL However, we have looked back and found that glucose and glucose metabolites are a common denominator in the release of these biomarkers.
The presented concept of glucose metabolism is highly original. All the key points regarding glucose metabolism as a signaling pathway already published, but interestingly have not been brought together into one unifying hypothesis.
Of course, not all the points highlighted are found in the common understanding of glucose metabolism and are therefore sometimes ignored or rejected by the scientific community.
The mitochondrial location of proton-linked MCT1 and LDH-h is highly controversial. Neither has a typical mitochondrial-targeting motif.
However, the presence of such a motif would dictate a mitochondrial location and thereby, exclude dynamic sorting of LDH-h and proton-linked MCT1. For example, GAPDH does not possess nuclear- or mitochondrial-localization motifs, yet it translocates to these compartments under certain cellular conditions [ 11 , 63 ].
In fact, topogenic signals and energy requirements dynamically regulate the localization of the proteins in yeast [ 64 ]. Biological processes are guided by economy; the saving of time and energy. In addition, the overall efficiency is reduced by the fact that mitochondrial G3P dehydrogenase uses the electron carrier flavin adenine dinucleotide FAD , which generates fewer ATP molecules i.
The rationale for connecting enzymes and forming functional enzymes complexes comes from the tentative 4 th law of thermodynamics [ 65 , 66 ] and the proton transport chain hypothesis [ 35 ].
The tentative 4 th law of thermodynamics was first described in physics and later transferred to economy and biology. A similar scenario exists for the mitochondrial ATP synthase complex.
For example, if the pH gradient driving the synthesis of ATP were flipped, then theoretically the mitochondrial ATP synthase complex would hydrolyze ATP and pump out protons. However, we prefer to stay within the realms of biology and understand that a flow of energy, in this case, protons, is sufficient to drive the ATP synthase complex and the PDH complex.
Thus, investigating isolated enzymes extrudes them from the biological context, disconnecting them from the flow of energy. Isolated enzymes in a test tube reversibly catalyze a concentration equilibrium in a closed system. In contrast, biology describes an open system where energy and material are free to move, i.
From our point of view, the rationale that quenching the activity of pH-sensitive astrocytic mitochondrial pyr - transporter is responsible for the effect is critical. This interpretation also suggests that the activity of neuronal pyr - transporter is virtually nil.
It might seem strange to develop a hypothesis of schizophrenia, without mentioning dopamine. However, floating down this path we would have to mention the constitutive activity of G protein-coupled receptors.
For example, the constitutive activity of dopaminelike receptors D 1 R and D 5 R increases basal cAMP levels. In contrast, the dopamine-induced effect decreases cAMP levels.
So what effect does this balance between constitutive and agonist-induced activity have on cell—cell signaling via neurotransmitters? How is cell homeostasis regulated by constitutive G protein-coupled receptor activity through regulation of glucose metabolism?
Thankfully for scientists, the cell is not just a set of randomly distributed pieces, but a complex puzzle to be solved. CRISPR-Cas9 targeting of any of the enzymes or transporters of the malate-aspartate shuttle results in impaired IFNγ cytokine production in activated Th1 cells.
Formally demonstrating that the activity of the shuttle network, rather than the TCA cycle, regulated CD4 T cell biology, targeting either shuttle or the cytosolic isoform of Mdh1 was sufficient to impair mitochondrial respiration. In addition to the impact of the malate-aspartate shuttle on histone acetylation, the shuttling network was also found to be essential for T cell proliferation through its control of respiration.
Indeed, impairing either Complex-I with the inhibitor rotenone or targeting the malate-aspartate shuttle results in a block in the synthesis of aspartate and its downstream nucleotide precursor, N-carbamoyl- l -aspartate. In this manner, CD4 T cell division is impaired when cells are treated with rotenone, but can be restored simply by supplementing with exogenous aspartate.
Sirtuin 1 SIRT1 -deficient T cells spontaneously activate, displaying a breakdown in tolerance. Sirtuin family members are also key regulators of post-translational modifications to non-histone proteins. Several studies have documented the role of sirtuin proteins in regulating acetylation of key T cell-associated transcription factors.
SIRT1 is critical for maintaining FoxO1 protein stability and sustaining oxidative phosphorylation in resting CD8 T cells. Perhaps the best characterized role for SIRT1 in T cells is in Treg biology. Acetylation blocks ubiquitination and proteasomal degradation of FoxP3. Active SIRT1 deacetylates FoxP3, allowing for its subsequent degradation.
Similar to SIRT1, SIRT4 regulates FoxP3 stability and Treg cell function. T cell-specific deletion of SIRT1 and SIRT1-specific inhibitors suppress the Th17 program and are protective in mouse models of experimental autoimmune encephalomyelitis EAE.
Mechanistically, active SIRT1 deacetylates the key Th17 cell transcription factor RORγt, enhancing its activity. In addition to regulating histone and protein acetylation, alterations in metabolic flux control the availability of substrates that regulate protein methylation Fig. Intermediates of the TCA cycle, 2-oxoglutarate 2OG and FAD, are obligate cofactors of the two main classes of histone demethylases, 2OG-dependent dioxygenases JMJ family and FAD-dependent amine oxidases LSD family.
Additionally, metabolites of the TCA cycle negatively regulate demethylase activity. For example, succinate and fumarate are potent allosteric inhibitors of the 2OG-dependent dioxygenases.
Therefore, the production and consumption of these metabolites, and their transport from the mitochondria to the cytosol dictates histone dynamics in the nucleus. Mitochondrial 2OG is generated by the TCA cycle or by glutaminolysis and transamination of glutamate.
In activated effector T cells, glutamine catabolism and transamination is the major source of 2OG synthesis. Highlighting the importance of 2OG to T cell transcriptional reprogramming, 2OG regulates roughly a third of all ILinduced genes.
This regulation occurs through JMJD3 and TET2-mediated removal of H3K27 trimethylation H3K27me3 and DNA methylation, respectively, at IL2-regulated loci. In this model, 2OG-dependent epigenetic modifications altered CTCF binding dynamics and chromatin structure.
Several studies have demonstrated how T cell production and consumption of 2OG can have program-specific effects on epigenetic remodeling during T cell differentiation.
Compared to Th1 and Treg cells, Th17 cells have higher rates of glutaminolysis and rely on this pathway for functional differentiation. Conversely, loss of GLS1 activity promotes Th1 cell cytokine production and Tbet expression.
shRNA targeting of GOT1 phenocopied this result, suggesting the activity of AOA is indeed through inhibition of glutaminolysis. Inhibition of GLS1 enhances H3K27me3 in Th17 cells, favoring differentiation of Th1 cells in a JMJD3-dependent mechanism.
Strikingly, these results are entirely dependent on the Th cell program being engaged. In contrast to cells stimulated in Thskewing conditions, cells treated with IL and IFNγ exhibit enhanced chromatin accessibility and elevated transcription upon GLS1 inhibition.
Glutaminolysis also regulates T cell differentiation by modulating DNA demethylation. In cancer cells, 2HG is a competitive inhibitor of 2OG-dependent dioxygenases, including TET and JMJ family demethylases.
Upon activation, CD8 T cells rapidly accumulate 2HG that promotes H3K27me3 and enhances H3K4me3 at promoter regions, with only minor effects on DNA demethylation. Upon treatment with 2HG, Treg cells convert to Th17 cells, even when maintained in Treg cell culture conditions.
Moreover, 2OG supplementation partially rescues AOA treatment or knockdown of the enzymes responsible for 2HG production, IDH1 and IDH2. These phenomena are at least in part explained by the activity of 2HG as an inhibitor of TET enzymes. Treatment with 2HG promotes DNA methylation of the FoxP3 promoter in both Th17 and Treg culture conditions.
Our understanding of immune cell metabolism and the interconnectedness of signaling with metabolic networks has changed rapidly over the past decade.
As described above, many of these core metabolic processes can play vastly different roles within the same lineage of cells, depending on the functional program they adopt.
This can even mean that some cell programs display a critical dependency on a pathway, while an alternate program is impeded by the same process. These discriminating metabolic features are both driven by the signaling pathways that dictate differentiation to sustain the biology of a given program and can act upstream of those same signaling networks to direct programming.
Ultimately, cells must generate the biochemical landscape needed to sustain the transcriptome and proteome launched by differentiating cues. This is ensured by incorporating metabolic information as an input in signaling. While this paradigm is now well established, there remain many outstanding questions.
The field is just beginning to explore how metabolic interconnectivity of organelles regulates immune cell biology. Glutaminolysis, citrate export, and the malate-aspartate shuttle all connect the mitochondria to the cytosol and in turn to the nucleus.
How other shuttling networks and the transport of metabolites in and out of the mitochondria pattern differentiation has only just begun to be explored. Moreover, we lack insight into how these changes mediate such profoundly specific alterations to gene expression when they affect seemingly fundamental and cell-wide processes i.
Zooming another level out, we know very little about how the flow of metabolites between interacting cells can mediate cell-to-cell communication or influence function. This is of particular interest within the immune system, whereby lymphocytes go from solitary circulating cells to fixed in intimate cell contact during antigen presentation and cell killing and the intercellular relationships between resident immune cells in organ systems.
Indeed, at the level of organ systems, we know very little how responses to infection or other alterations in homeostasis in one system e.
Illuminating how this higher order metabolic architecture is organized through the lens of metabolic signaling offers the opportunity for the development of a new wave of metabolic therapies for cellular and host pathology.
An amendment to this paper has been published and can be accessed via a link at the top of the paper. van der Windt, G. et al. CD8 memory T cells have a bioenergetic advantage that underlies their rapid recall ability.
USA , — Article PubMed PubMed Central Google Scholar. Gubser, P. Article CAS PubMed Google Scholar. Immunity 41 , 75—88 Article PubMed PubMed Central CAS Google Scholar. Bretscher, P. A theory of self-nonself discrimination.
Science , — June, C. T-cell proliferation involving the CD28 pathway is associated with cyclosporine-resistant interleukin 2 gene expression. CAS PubMed PubMed Central Google Scholar. Mueller, D. Clonal expansion versus functional clonal inactivation: a costimulatory signalling pathway determines the outcome of T cell antigen receptor occupancy.
Smith-Garvin, J. T cell activation. Article CAS PubMed PubMed Central Google Scholar. Chen, L. Molecular mechanisms of T cell co-stimulation and co-inhibition. Kapeller, R. Identification of two SH3-binding motifs in the regulatory subunit of phosphatidylinositol 3-kinase.
Prasad, K. Src-homology 3 domain of protein kinase p59fyn mediates binding to phosphatidylinositol 3-kinase in T cells. USA 90 , — Saveliev, A.
Function of the nucleotide exchange activity of vav1 in T cell development and activation. Reynolds, L. Vav1 transduces T cell receptor signals to the activation of phospholipase C-gamma1 via phosphoinositide 3-kinase-dependent and -independent pathways. Bruyns, E.
T cell receptor TCR interacting molecule TRIM , a novel disulfide-linked dimer associated with the TCR-CD3-zeta complex, recruits intracellular signaling proteins to the plasma membrane.
Osman, N. The protein interactions of the immunoglobulin receptor family tyrosine-based activation motifs present in the T cell receptor zeta subunits and the CD3 gamma, delta and epsilon chains.
Waickman, A. mTOR, metabolism, and the regulation of T-cell differentiation and function. MacIver, N. Metabolic regulation of T lymphocytes. Frauwirth, K. The CD28 signaling pathway regulates glucose metabolism. Immunity 16 , — Wieman, H. Cell 18 , — Jacobs, S. Glucose uptake is limiting in T cell activation and requires CDmediated Akt-dependent and independent pathways.
Macintyre, A. The glucose transporter Glut1 is selectively essential for CD4 T cell activation and effector function. Cell Metab.
Wofford, J. IL-7 promotes Glut1 trafficking and glucose uptake via STAT5-mediated activation of Akt to support T-cell survival. Blood , — IL-7 is essential for homeostatic control of T cell metabolism in vivo.
Maekawa, Y. Angelin, A. Foxp3 reprograms T cell metabolism to function in low-glucose, high-lactate environments.
Gerriets, V. Foxp3 and Toll-like receptor signaling balance T reg cell anabolic metabolism for suppression. De Rosa, V. Glycolysis controls the induction of human regulatory T cells by modulating the expression of FOXP3 exon 2 splicing variants. Priyadharshini, B. Cutting edge: TGF-β and phosphatidylinositol 3-kinase signals modulate distinct metabolism of regulatory T cell subsets.
Patsoukis, N. PD-1 alters T-cell metabolic reprogramming by inhibiting glycolysis and promoting lipolysis and fatty acid oxidation. Bettonville, M. Long-term antigen exposure irreversibly modifies metabolic requirements for T cell function. Elife 7 , e Shi, L. HIF1alpha-dependent glycolytic pathway orchestrates a metabolic checkpoint for the differentiation of TH17 and Treg cells.
Finlay, D. Doedens, A. Zeng, H. mTORC1 and mTORC2 kinase signaling and glucose metabolism drive follicular helper T cell differentiation.
Immunity 45 , — MTORC1 couples immune signals and metabolic programming to establish Treg-cell function. Nature , — Ray, J. The interleukinmTORc1 kinase axis defines the signaling, differentiation, and metabolism of T helper 1 and follicular B helper T cells article the interleukinmTORc1 kinase axis defines the signaling, differentiation, and metabolism of T helper 1.
Immunity 43 , — Yang, J. Critical roles of mTOR complex 1 and 2 for T follicular helper cell differentiation and germinal center responses. Elife 5 , 1—22 Google Scholar. Pollizzi, K. Verbist, K. Metabolic maintenance of cell asymmetry following division in activated T lymphocytes.
Chornoguz, O. mTORC1 promotes T-bet phosphorylation to regulate Th1 differentiation. Kidani, Y. Sterol regulatory element-binding proteins are essential for the metabolic programming of effector T cells and adaptive immunity.
Yang, K. T cell exit from quiescence and differentiation into Th2 cells depend on raptor-mTORC1-mediated metabolic reprogramming. Immunity 39 , — Tan, H. Integrative proteomics and phosphoproteomics profiling reveals dynamic signaling networks and bioenergetics pathways underlying T cell activation.
Immunity 46 , — Wang, R. The transcription factor Myc controls metabolic reprogramming upon T lymphocyte activation. Immunity 35 , — Dang, E.
Cell , — Lindsten, T. Multiple mechanisms regulate c-myc gene expression during normal T cell activation. EMBO J. Guy, C. Distinct TCR signaling pathways drive proliferation and cytokine production in T cells.
Preston, G. Marchingo, J. Quantitative analysis of how Myc controls T cell proteomes and metabolic pathways during T cell activation. Elife 9 , e Herzig, S. AMPK: guardian of metabolism and mitochondrial homeostasis.
Cell Biol. Inoki, K. TSC2 mediates cellular energy response to control cell growth and survival. Gwinn, D. AMPK phosphorylation of raptor mediates a metabolic checkpoint. Cell 30 , — Blagih, J. The energy sensor AMPK regulates T cell metabolic adaptation and effector responses in vivo.
Immunity 42 , 41—54 Previte, D. PLoS ONE 12 , e Sancak, Y. The rag GTPases bind raptor and mediate amino acid signaling to mTORC1. Kim, E. Regulation of TORC1 by Rag GTPases in nutrient response.
Saxton, R. Structural basis for leucine sensing by the Sestrin2-mTORC1 pathway. Science , 53—58 Wolfson, R. Sestrin2 is a leucine sensor for the mTORC1 pathway.
Science , 43—48 Chantranupong, L. The CASTOR proteins are arginine sensors for the mTORC1 pathway. Mechanism of arginine sensing by CASTOR1 upstream of mTORC1.
Gu, X. SAMTOR is an S-adenosylmethionine sensor for the mTORC1 pathway. Jewell, J. Differential regulation of mTORC1 by leucine and glutamine. mTOR signaling in growth, metabolism, and disease. Carr, E. Nakaya, M. Inflammatory T cell responses rely on amino acid transporter ASCT2 facilitation of glutamine uptake and mTORC1 kinase activation.
Immunity 40 , — Johnson, M. Distinct regulation of Th17 and Th1 cell differentiation by glutaminase-dependent metabolism.
Sinclair, L. Control of amino-acid transport by antigen receptors coordinates the metabolic reprogramming essential for T cell differentiation. Ananieva, E. Shi, H. Amino acids license kinase mTORC1 activity and treg cell function via small G proteins Rag and Rheb.
Immunity 51 , — Devadas, S. Discrete generation of superoxide and hydrogen peroxide by T cell receptor stimulation: selective regulation of mitogen-activated protein kinase activation and Fas ligand expression.
Mak, T. Glutathione primes T cell metabolism for inflammation. Crabtree, G. Contingent genetic regulatory events in T lymphocyte activation.
Mustelin, T. T cell antigen receptor-mediated activation of phospholipase C requires tyrosine phosphorylation. Balla, T. Phosphoinositides: tiny lipids with giant impact on cell regulation.
Di Cristofano, A. Article PubMed Google Scholar. Suzuki, A. T cell-specific loss of Pten leads to defects in central and peripheral tolerance. Immunity 14 , — Liu, X. Distinct roles for PTEN in prevention of T cell lymphoma and autoimmunity in mice. Walsh, P. Hu, Z. Ho, P.
Phosphoenolpyruvate is a metabolic checkpoint of anti-tumor T cell responses. Chen, H. Relationship between sterol synthesis and DNA synthesis in phytohemagglutinin-stimulated mouse lymphocytes.
USA 72 , — Chakrabarti, R. Interrelationships between mevalonate metabolism and the mitogenic signaling pathway in T lymphocyte proliferation. Bensinger, S. LXR signaling couples sterol metabolism to proliferation in the acquired immune response.
Cell , 97— Dustin, M. A novel adaptor protein orchestrates receptor patterning and cytoskeletal polarity in T-cell contacts. Cell 94 , — Monks, C. Three-dimensional segregation of supramolecular activation clusters in T cells. Nature , 82—86 Grakoui, A. The immunological synapse: a molecular machine controlling T cell activation.
Montixi, C. Engagement of T cell receptor triggers its recruitment to low-density detergent-insoluble membrane domains. Moran, M. Engagement of GPI-linked CD48 contributes to TCR signals and cytoskeletal reorganization: a role for lipid rafts in T cell activation.
Immunity 9 , — Viola, A. T lymphocyte costimulation mediated by reorganization of membrane microdomains. Burack, W. Cutting edge: quantitative imaging of raft accumulation in the immunological synapse. Gaus, K. Condensation of the plasma membrane at the site of T lymphocyte activation.
Yang, W. Janes, P. Aggregation of lipid rafts accompanies signaling via the T cell antigen receptor. Harder, T. Selective accumulation of raft-associated membrane protein LAT in T cell receptor signaling assemblies.
Drevot, P. TCR signal initiation machinery is pre-assembled and activated in a subset of membrane rafts. Xavier, R. Membrane compartmentation is required for efficient T cell activation.
Immunity 8 , — Bunnell, S. T cell receptor ligation induces the formation of dynamically regulated signaling assemblies. Douglass, A. Single-molecule microscopy reveals plasma membrane microdomains created by protein-protein networks that exclude or trap signaling molecules in T cells.
Huang, B. Cholesterol metabolism in cancer: mechanisms and therapeutic opportunities. Dunn, S. Glycolysis does not require oxygen and is, therefore, the predominant catabolic pathway in anaerobic organisms.
Glycolysis is also utilized by "otherwise aerobic" organisms when oxygen is limited. PKM2 D78A4 XP® Rabbit mAb Confocal IF analysis of A cells using PKM2 D78A4 XP® Rabbit mAb green. PKM2 D78A4 XP ® Rabbit mAb Confocal IF analysis of A cells using PKM2 D78A4 XP ® Rabbit mAb green.
The citric acid cycle, also known as the Krebs cycle, is the next step in intracellular glucose metabolism. It takes place in the mitochondria and is initiated by acetyl coenzyme A acetyl CoA , which is an oxidized derivative of the pyruvate produced during glycolysis. The citric acid cycle requires oxygen and releases carbon dioxide and water as byproducts.
It is composed of a series of redox reactions that generate the high-energy molecules NADH, FADH 2 , and ATP. For each glucose molecule, two pyruvates are produced via glycolysis; thus, the citric acid cycle goes around twice and produces two carbon dioxide molecules, three NADH, one FADH 2 , and one ATP for each turn.
While the citric acid cycle does not itself produce much ATP, the major energy currency of the cell, the NADH and FADH 2 molecules act as electron carriers that shuttle into the electron transport chain for oxidative phosphorylation and high-energy production.
The final stage of cellular respiration is oxidative phosphorylation OXPHOS. OXPHOS also takes place in the mitochondria, across the inner membrane.
There are five transmembrane enzyme complexes that drive the transfer of electrons from one molecule to another in a series of redox reactions. This is known as the electron transport chain.
The transfer of electrons along the electron transport chain releases energy that then drives proton pumps to translocate protons against their concentration gradient from the mitochondrial matrix, across the inner mitochondrial membrane, and into the intermembrane space.
This is the origin of the electrochemical gradient the drives OXPHOS. Accumulated protons in the intermembrane space then pass through the final complex in the electron transport chain, ATP synthase, along their concentration gradient into the mitochondrial matrix. The energy released by protons flowing down their concentration gradient through the ATP synthase molecule drives its function as a "molecular motor" that uses the energy to catalyze the addition of a phosphate group to the ADP precursor, creating ATP.
One glucose molecule yields 30 to 36 ATP molecules from oxidative phosphorylation. As discussed above, in cellular respiration the pyruvate produced during glycolysis is shunted into the citric acid cycle and oxidative phosphorylation.
However, there is an alternative cytosolic pathway that branches off glycolysis and leads to the formation of the sugars necessary for DNA and RNA production.
The pentose phosphate pathway utilizes a molecule that is produced in the first step of glycolysis—glucosephosphate. Glucosephosphate is generated through the addition of a phosphate group to glucose and is used by the pentose phosphate pathway to generate NADPH, five-carbon sugars known as pentoses, and ribose 5-phosphate, which serve as precursor molecules for nucleotide synthesis.
NADPH plays an important functional role in not only the pentose phosphate pathway but in other biosynthetic processes, including fatty acid metabolism and reactive oxygen species ROS control. Glutamine is an important fuel source in rapidly proliferating cells. It is transported into cells via a specific amino acid transporter and converted into glutamate in the mitochondria.
There, the glutamate is converted into α-ketoglutarate, an intermediate in the citric acid cycle. The urea cycle, also called the ornithine cycle, is necessary to prevent toxic buildup of ammonia in the body and occurs mostly in the liver.
It is composed of biochemical reactions that produce urea from ammonium ions that are a byproduct of amino acid breakdown. In this cycle, carbon dioxide combines with the ammonia that is produced from the transamination of amino acids during protein metabolism, resulting in the generation of urea and water that are later eliminated as urine by the kidneys.
The initial steps of the urea cycle take place in the mitochondria, and the later steps proceed in the cytosol. Fatty acids are both a source and storage unit of energy in the cell. In addition, fatty acids play a major role in cellular signaling and, therefore, heavily influence cellular function.
Fatty acid synthesis takes place in the cytosol and involves the creation of fatty acids from acetyl-CoA and NADPH in a process that is catalyzed by fatty acid synthases. Acetyl-CoA units that are necessary for fatty acid synthesis are provided by the breakdown of glucose through glycolysis.
Glucose breakdown also generates glycerol, which can combine with three fatty acid subunits to form triglycerides. Generation of phospholipids is also a critical part of fatty acid metabolism, as phospholipids are a major component of biological membranes.
When glycerol combines with only two fatty acids and a phosphate group, this results in the formation of phospholipids. Phospholipids have many functions within the cell, but, most importantly, they form the lipid bilayer that makes up the cell membrane. Aside from being the building blocks of cell and organelle membranes, phospholipids have been used in drug synthesis to increase permeability across the membrane and to improve drug bioavailability.
Lipin 1 D2W9G Rabbit mAb Confocal IF analysis of 3T3-L1 adipocytes differentiated, 7 days using Lipin 1 D2W9G Rabbit mAb red. Fatty acid beta-oxidation is the process by which fatty acids are broken down into their constituent acetyl-CoA subunits in the mitochondria.
This acetyl-CoA then enters the citric acid cycle for sequential oxidation and the generation of NADH and FADH 2. Gluconeogenesis refers to the generation of glucose from sources other than carbohydrates.
Similar to glycogenolysis, gluconeogenesis is an adaptive process that occurs primarily in the liver to ensure that blood glucose levels do not drop too low. Gluconeogenesis usually occurs during periods of low nutrient intake, intense exercise, or low-carbohydrate food consumption.
One-carbon metabolism refers to a group of folate-dependent metabolic pathways that are essential for the anabolism of several molecules, such as amino acids and nucleotides. In this pathway, folic acid acts as a carrier of one-carbon groups, facilitating the removal and transfer of these groups from donor molecules.
There are three molecules that can be used to move one-carbon groups: tetrahydrofolate, a folate-derivative that acts as cofactor for several enzymes; s-adenosylmethionine, a methyl donor; and vitamin B12, a coenzyme in methylation and carbon rearrangement reactions.
Aside from its role in amino acid and nucleotide synthesis, one-carbon metabolism is also important for DNA and histone methylation.
In mitochondria, the by-products of ATP generation via electron transport include reactive oxygen species ROS , which are highly reactive molecules that can cause oxidative damage to organelles and other cellular structures.
At low physiologic levels, ROS toxicity can be adequately controlled by intracellular antioxidant systems, including superoxide dismutase SOD , glutathione, and catalase. Basal ROS levels are also now known to play a critical role in physiological cell pathways.
However, pathological ROS levels have been shown to damage proteins, lipids, and DNA, which can lead to defective mitochondrial metabolism and deleterious consequences for cell function and viability.
Effectively coping with ROS-induced mitochondrial damage is key to maintaining cell function and viability under different forms of cellular stress.
Different environmental conditions or cellular perturbations can induce oxidative stress. In the metabolic context, oxidative stress can occur when nutrient supply exceeds energy demand.
This leads to a backup in the electron transport chain that can cause electrons to "leak" and react with O 2 to form ROS. Dysfunction of respiratory chain components can also result in perturbed electron transport and increased ROS levels.
Likewise, a deficit in antioxidant enzyme capacity, either due to decreased protein expression or decreased function, will allow ROS to accumulate.
Though mitochondria are a major source of ROS, oxidative stress can also result from increased levels of ROS and reactive nitrogen species RNS from other cellular sources, including xanthine oxidase and cytochrome P oxidase systems in cells such as phagocytes. ROS come in different forms, with the two main groups being free radicals species with unpaired electrons and nonradicals no unpaired electrons.
When free electrons first react with O 2 , this forms superoxide anion O 2 - , which is a very reactive but unstable ROS. Superoxide is quickly converted to hydrogen peroxide H 2 O 2 by superoxide dismutase SOD. Though hydrogen peroxide is more stable, it can be converted to harmful hydroxyl radicals -OH following interactions with transition metals, in a process known as the Fenton reaction.
Hydroxyl radicals are the most highly reactive ROS and, therefore, the most likely to cause oxidative damage to intracellular proteins and lipids.
Under hypoxic oxygen-limiting conditions, electron transport proceeds normally, but there is limited oxygen available to serve as final electron acceptors. In these cases, electron transport proceeds normally, but with no oxygen to accept the electrons. Left unchecked, this leads to increased electron leakage and ROS production.
Accordingly, the cell has evolved specialized hypoxia response pathways that influence cellular respiration and associated oxidative stress. These pathways function to downregulate metabolic activity to avoid overwhelming the bioenergetic machinery. A key transcription factor, called hypoxia-inducible factor-1 HIF-1 , responds to reduced oxygen availability by decreasing electron transport chain activity and downregulating protein translation an ATP-demanding process and activity of Na-K-ATPase.
This coordinated response allows the cell and mitochondria to withstand the period of lower oxygen until normal levels are restored. HIF-1α D1S7W XP® Rabbit mAb Confocal IF analysis of Hep G2 cells, untreated left or treated with cobalt chloride μM, 24 h; right , using HIF-1α D1S7W XP® Rabbit mAb green.
HIF-1α D1S7W XP ® Rabbit mAb Confocal IF analysis of Hep G2 cells, untreated left or treated with cobalt chloride μM, 24 h; right , using HIF-1α D1S7W XP ® Rabbit mAb green. Since oxidative stress is a multifaceted and complex process, defining the best biomarkers of oxidative stress in cells and tissues is challenging.
One approach is to measure biochemical modifications of target molecules e. Oxidized low-density lipoprotein is also a common biomarker, especially in cardiovascular disease.
Lipid modifications can also be detected following oxidative damage.
Mais JubouriDiet and exercise for weight management G. TalaricoCarbohydrate metabolism and cell signaling WeberMetabolismm A. Mennigen; Muscle soreness treatment alters Cdll carbohydrate metabolism sivnaling rainbow trout: glucose flux and cell signaling. J Exp Biol 1 August ; 15 : jeb In rainbow trout, dietary carbohydrates are poorly metabolized compared with other macronutrients. One prevalent hypothesis suggests that high dietary amino acid levels could contribute to the poor utilization of carbohydrates in trout. In mammals, alanine is considered an important gluconeogenic precursor, but has Carbouydrate been found to stimulate AMP-activated protein kinase AMPK to reduce glucose levels.As the environments of most sigaling are constantly changing, the reactions of metabolism must be finely regulated to maintain a constant set of conditions within Cabohydrate. Metabolic Muscle soreness treatment also xell organisms to Leafy green growers to Caebohydrate and interact actively with Singaling environments.
Two closely-linked concepts metabbolism important for understanding how metabolic pathways are controlled. Firstly, the signalig of an enzyme in a pathway is how its Healing nutrition plan for injuries is increased and decreased in response to signals.
Secondly, the control exerted by signalkng enzyme is cdll effect that meetabolism Leafy green growers signalinng its activity have on the overall rate of the pathway. For Diabetic ketoacidosis, an enzyme Carohydrate show large changes in activity i.
it wignaling highly sjgnalingbut if these signwling have little effect on the rate of DEXA scan benefits Leafy green growers pathway, then this enzyme is not involved Muscle soreness treatment the control of the pathway.
The result of one Czrbohydrate signaling Muscle soreness treatment affects muscle cells and Mental focus and decision making a good example of an increase signalijg cellular ceol.
The activation of β-adrenergic Muscle soreness treatment in zignaling cells by Carbohydeate leads to metabolissm increase in cyclic vell monophosphate also known as cyclic AMP or cAMP inside the cell.
Also known as epinephrine, adrenaline is a hormone produced by the adrenal gland attached to the kidney that prepares the body for short-term emergencies.
Cyclic AMP activates PKA protein kinase Awhich in turn phosphorylates two enzymes. The first enzyme promotes the degradation of glycogen by activating intermediate glycogen phosphorylase kinase GPK that in turn activates glycogen phosphorylase GPwhich catabolizes glycogen into glucose.
Recall that your body converts excess glucose to glycogen for short-term storage. When energy is needed, glycogen is quickly reconverted to glucose.
Phosphorylation of the second enzyme, glycogen synthase GSinhibits its ability to form glycogen from glucose. In this manner, a muscle cell obtains a ready pool of glucose by activating its formation via glycogen degradation and by inhibiting the use of glucose to form glycogen, thus preventing a futile cycle of glycogen degradation and synthesis.
Search site Search Search. Go back to previous article. Sign in. Learning Objectives Explain how cellular metabolism can be altered. Increase in Cellular Metabolism As the environments of most organisms are constantly changing, the reactions of metabolism must be finely regulated to maintain a constant set of conditions within cells.
cAMP serves as a second messenger to activate or inactivate proteins within the cell. Key Points The activation of β-adrenergic receptors in muscle cells by adrenaline leads to an increase in cyclic AMP. Cyclic AMP activates PKA protein kinase Awhich phosphorylates two enzymes.
Phophorylation of the first enzyme promotes the degradation of glycogen by activating intermediate GPK that in turn activates GP, which catabolizes glycogen into glucose. The inhibition of glucose to form glycogen prevents a futile cycle of glycogen degradation and synthesis, so glucose is then available for use by the muscle cell.
Key Terms cyclic adenosine monophosphate : cAMP, a second messenger derived from ATP that is involved in the activation of protein kinases and regulates the effects of adrenaline epinephrine : adrenaline an amino acid-derived hormone secreted by the adrenal gland in response to stress protein kinase A : a family of enzymes whose activity is dependent on cellular levels of cyclic AMP cAMP.
: Carbohydrate metabolism and cell signalingMetabolism | Cell Signaling Technology | However, the molecular mechanisms involved appear to be different. In mice, the alanine-dependent reduction in glycemia is linked to the activation of hepatic AMPK, the stimulation of glucose uptake and reduction of its release Adachi et al. In trout, alanine failed to stimulate hepatic p-AMPK, although total AMPK increased. However, p-AKT increased in red muscle, but not in liver, mimicking earlier findings after insulin infusion in trout Forbes et al. Together, the present results suggest that the glucose-lowering effects of alanine are indirectly mediated by insulin in trout. Alanine inhibited glucose R a in trout Fig. Glucose R a is controlled by gluconeogenesis and glycogen breakdown glycogenolysis. The key enzymes catalyzing the first step in gluconeogenesis and glycogenolysis are PCK and glycogen phosphorylase GP , respectively. The last step of both the gluconeogenic and glycogenolytic pathways is catalyzed by G6Pc Enes et al. In trout, alanine affected neither pck expression Fig. S1A or PCK activity Fig. In juvenile trout fed an alanine-rich diet over several weeks, no effect on cytosolic PCK activity and an inhibition of the expression and activity of G6Pc were found Kirchner et al. Despite the different experimental designs, both the current work and the dietary study by Kirchner and colleagues show that alanine does not stimulate hepatic glucose production in trout. In mammalian and avian species, alanine had no effect on the activity of PCK and G6Pc Donaldson and Christensen, ; Friedrichs and Schoner, Given the observed alanine-dependent reduction of glucose R a in the absence of effects on PCK activity, it is possible that alanine inhibited hepatic glycogenolysis. Glycogen metabolism is principally regulated by phosphorylation, which elicits opposing effects on glycogen phosphorylase activated by phosphorylation and glycogen synthase inhibited by phosphorylation Enes et al. While the hepatic expression and activity of GP and glycogen content were not measured in the current study, findings in fish and mammals suggest a role for alanine in promoting hepatic glycogen synthesis. In sea raven Hemitripterus americanus hepatocytes, insulin increased gluconeogenesis from alanine and had a small positive effect on glycogen content Foster and Moon, In mammals, hepatic glycogen synthesis from alanine was reported Shalwitz et al. Together, our findings refute the hypothesis that alanine increases glucose R a. However, future studies investigating the potential effects of alanine on liver glycogen metabolism are warranted. Alanine inhibits glucose R d in rainbow trout Fig. The same effect was reported in humans Jahoor et al. However, glucose utilization was unaffected by alanine in vitro in rat brain da Graça Lütz et al. Glucose disposal is regulated by glucose uptake, glycolysis and glycogen synthesis. Glucose uptake is mainly mediated through GLUTs Navale and Paranjape, The insulin-sensitive GLUT4, found mostly in muscle and adipose tissue, plays a key role in glucose disposal in mammals and trout Díaz et al. The first step in the glycolytic pathway is catalyzed by HK in muscle and GK in liver Enes et al. Glycogen synthase GS is a key enzyme in glycogenesis Enes et al. Alanine decreased glut4b in red muscle Fig. This reduction in glut4b expression may partially explain the decrease in glucose R d. Alanine did not affect the expression Fig. S2 of gk in the liver. Because the expression of gk in trout is a sensitive marker of hepatic glucose concentration Panserat et al. Similarly, a 9 week dietary alanine treatment had no effect on hepatic glycolysis in juvenile rainbow trout, as indicated by a lack of change in the expression and activity of GK Kirchner et al. In vitro incubation of mammalian H4IIE hepatocytes with alanine increased glucose uptake Adachi et al. Because studies on the effect of alanine after feeding, injection or infusion on hepatic glycolytic enzyme activity in mammals have not been reported to our knowledge, it is currently unclear whether these differences reflect methodological or true species differences. While indices of glycogen metabolism were not quantified in our study, previous work using fish and mammalian hepatocytes suggests that exogenous alanine is metabolized to glycogen Baquet et al. It is therefore feasible that alanine may be used to support hepatic glycogen synthesis in rainbow trout. Overall, the decrease in glucose R d suggests that the preference for glucose as an oxidative fuel decreased and that it was partly replaced by alanine, although the amino acid was probably also used for hepatic glycogen synthesis. Decreased glucose import and utilization is further corroborated at the molecular level in muscle, where alanine caused reductions in the expression of glucose transporters. To our knowledge, this is the first study examining the impact of alanine on AMPK in trout or any fish species. Alanine increased total AMPK in liver Fig. AMPK is a heterotrimeric complex composed of three distinct subunits Jeon, It is activated when phosphorylated at Thr within the α-subunit and by binding of AMP within the γ-subunit Craig et al. In mammals, AMPK activation by alanine inhibited TOR targets, S6 kinase and S6, and reduced glycemia in vivo Adachi et al. In the current study, TOR targets, S6 and 4EBP1, were unaffected by alanine, further supporting that AMPK was not activated by alanine Figs S3 and S4. A differential response of AMPK activation following alanine administration may be linked to physiological and molecular differences between trout and mammals. At the physiological level, activation of hepatic AMPK in mammals was linked to increased transamination of alanine by ALT and subsequent urea synthesis, whose energetic cost contributed to an increase in AMP levels Adachi et al. Conversely, ALT activity was unaffected by alanine in trout liver Fig. Nitrogen waste in adult trout is mainly excreted as ammonia, while urea accounts for a small amount Panserat et al. Ammonia-based nitrogen detoxification is energetically less costly compared with urea-based strategies Ip and Chew, This difference in energetic cost may partially explain the lack of AMPK phosphorylation in trout. This would result in a comparatively smaller increase in AMP levels in rainbow trout, which in turn may not be high enough to trigger AMPK activation. At the molecular level, salmonid-specific whole-genome duplication led to the retention of 15 genes encoding AMPK subunits in rainbow trout, whereas only 7 genes exist in humans Causey et al. This complexity and potential divergent regulation of paralogs may also contribute to the different responses. In contrast to the recent mammalian study Adachi et al. Alanine increased p-AKT levels in red muscle but not liver Fig. Interestingly, this finding is consistent with results after exogenous insulin infusion in rainbow trout Forbes et al. In addition to the similar pattern of p-AKT stimulation following alanine and insulin infusion, the reductions in glycemia, as well as glucose R a and R d were strikingly similar between the two studies. This suggests that alanine may have stimulated insulin secretion. Indeed, previous studies support the idea that alanine as well as other amino acids stimulate insulin secretion in teleost fishes, including rainbow trout Andoh, ; Plisetskaya et al. Unfortunately, insulin levels cannot be readily measured in rainbow trout Moon, Mammalian studies report different effects of alanine on pancreatic hormone release. For example, alanine increased insulin Genuth and Castro, and glucagon Sann et al. Interestingly, alanine can differentially regulate both hormones under different conditions in dogs Müller et al. When glucose was available exogenous load , alanine raised the ratio of insulin to glucagon, whereas it stimulated glucagon secretion when glucose was lacking Müller et al. Conversely, other findings in mammals suggest alanine had no effect on both hormones Adachi et al. In the current study, glucagon was not measured because of the limited plasma volume available. However, given that exogenous glucagon increased glycemia and glucose R a , and decreased glucose R d in trout Forbes et al. The concurrent reduction in glucose R a and R d suggests that glucose was not the preferred fuel under high alanine availability. This indicates that alanine was predominantly used as an oxidative fuel, replacing glucose to some extent, which supports our second hypothesis. Glucose represents a minor energy source in trout and amino acids from dietary proteins can be oxidized to provide energy Panserat et al. The lack of expression and activity changes of the key gluconeogenic enzyme PCK in this study suggests that alanine was also possibly used for hepatic glycogen synthesis via gluconeogenesis. Moreover, glycogen breakdown was probably inhibited in the liver given the reduction in R a glucose. This likely occurred at the level of glycogen phosphorylase because a general lack of change in the expression of g6pc paralogs was observed. However, additional studies will be necessary to probe the specific effect of alanine on hepatic glycogen metabolism. After the conversion of alanine to pyruvate, the latter can be oxidized to provide energy Mommsen et al. Under our conditions, energy requirements were probably met by alanine oxidation and it would be less energetically favorable to convert alanine to glucose before oxidation. In trout muscle, we speculate that alanine was preferentially oxidized over glucose because consistent reductions in molecular indices of glucose transport were found. Unfortunately, the fate of alanine or other amino acids in trout muscle remains largely unexplored Weber et al. In hepatocytes from long-term fasted rainbow trout, alanine oxidation, as assessed by CO 2 production, was significantly higher than that of glucose Pereira et al. The production of CO 2 from alanine was higher than its conversion to glucose in trout hepatocytes, indicating that alanine is a good oxidative substrate French et al. In sea raven hepatocytes, insulin stimulated CO 2 production from alanine Foster and Moon, The above-mentioned studies were conducted in vitro. Therefore, they should be interpreted with caution. In mammals, many reports found no effect of alanine on glucose R a Diamond et al. Thus, three fates were suggested for alanine in mammals. First, hepatic glucose produced from alanine would be converted to glycogen Wolfe et al. The similar effect of both alanine present study and insulin Forbes et al. This is the first study to investigate the effects of alanine on glucose metabolism in trout by integrating glucose flux at the whole-organism level and molecular indices in liver and muscle tissues. Proteins, and thus amino acids, represent the main nutritional energy source in this species. In addition to providing a better comparative understanding of their impact on glucose metabolism in trout, our findings provide a starting-step toward a better understanding of the effect of individual amino acids on glucose metabolism in the applied context of the salmonid aquaculture sector, for which the replacement of costly dietary proteins with carbohydrate protein represents an economical advantage. The reduction in glucose flux suggests that glucose was not the preferred substrate under our conditions high alanine availability and trout at rest and that alanine oxidation was probably sufficient to meet energy requirements. Mechanistically, alanine does not change molecular indices of hepatic glycolysis and gluconeogenesis, raising the possibility that the reduction in R a is linked to altered glycogen metabolism. The reduction of molecular indices related to glucose import in muscle tissue supports decreased peripheral utilization of glucose under conditions of high alanine availability. Comparatively, the alanine-dependent reduction in glycemia at the whole-animal level is consistent with findings in mammals. However, while the reduction in glycemia was mediated through AMPK activation in mammals, our data suggest that the effects of alanine do not involve AMPK activation in trout and might be indirectly mediated through insulin. Thus, different mechanisms underlie alanine's glucose-lowering effects in trout and mammals. Given the striking similarities in the regulation and mechanistic underpinnings of glucose flux between alanine- and insulin-infused trout, we hypothesize that alanine alters glucose flux by acting as an insulin secretagog in this species. Future studies using insulin receptor antagonists are warranted to investigate this hypothesis. We thank Christine Archer for expert care of the animals and Katie Gilmour for providing AMPK antibodies. Conceptualization: M. This work was supported by grants from the Natural Sciences and Engineering Research Council of Canada to J. NSERC discovery grant and to J. NSERC discovery grant , and the Canadian Foundation for Innovation John Evans Leader's fund Learn more in our Editorial. Places are limited to 24 attendees, and applicants should apply through the SEB registration page by 30 April In their Review , Corinna Gebehart and Ansgar Büschges discuss how the motor systems of insects can provide insight into the mechanisms underlying the integration of multimodal information to allow flexible motor control. Jason Dallas and colleagues show that the community of microbes inhabiting the guts of tadpoles contributes to their ability to withstand rising temperature and suggests that microbiome transplants could offer hope to species at risk as global temperatures rise. Next deadline for applications is 3 June Sign In or Create an Account. Search Dropdown Menu. header search search input Search input auto suggest. filter your search All content All journals Journal of Experimental Biology. Advanced Search. User Tools Dropdown. Sign in. Toggle Menu Menu Articles Accepted manuscripts Latest complete issue Issue archive Archive by article type Special issues Subject collections Interviews Sign up for alerts About us About JEB Editors and Board Editor biographies Travelling Fellowships Grants and funding Research Partnership Kickstart Travel Grants ECR Visiting Fellowships Journal Meetings Workshops The Company of Biologists The Forest of Biologists Journal news For authors Submit a manuscript Aims and scope Presubmission enquiries Article types Manuscript preparation Cover suggestions Editorial process Promoting your paper Open Access Outstanding paper prize Biology Open transfer Journal info Journal policies Rights and permissions Media policies Reviewer guide Sign up for alerts For librarians Contacts Contacts Subscriptions Advertising Feedback. Skip Nav Destination Close navigation menu Article navigation. Acute glucose fluctuation impacts microglial activity, leading to inflammatory activation or self-degradation. Sci Rep. Google Scholar. Steiner J, Bernstein H-G, Schiltz K, Müller UJ, Westphal S, Drexhage HA, et al. Immune system and glucose metabolism interaction in schizophrenia: A chicken—egg dilemma. Prog Neuro-Psychopharmacol Biol Psychiatry. Holmes E, Tsang TM, Huang JT-J, Leweke FM, Koethe D, Gerth CW et al. Metabolic profiling of CSF: evidence that early intervention may impact on disease progression and outcome in schizophrenia. PLoS Med. Roosterman D, Meyerhof W, Cottrell GS. Proton transport chains in glucose metabolism: mind the proton. Becker HM, Klier M, Schüler C, McKenna R, Deitmer JW. Intramolecular proton shuttle supports not only catalytic but also noncatalytic function of carbonic anhydrase II. Proc Natl Acad Sci USA. Meyerhof O. Recent investigations on the aerobic and an-aerobic metabolism of carbohydrates. J Gen Physiol. Zippin JH, Levin LR, Buck J. Trends Endocrinol Metab. Roosterman D, Cottrell GS. Astrocytes and neurons communicate via a monocarboxylic acid shuttle. AIMS Neurosci. Article PubMed PubMed Central Google Scholar. Hashimoto T, Hussien R, Brooks GA. Colocalization of MCT1, CD, and LDH in mitochondrial inner membrane of L6 muscle cells: evidence of a mitochondrial lactate oxidation complex. Am J Physiol Endocrinol Metab. Dianzani MU. Distribution of lactic acid oxidase in liver and kidney cells of normal rats and rats with fatty degeneration of the liver. Archivio Di Fisiologia. CAS PubMed Google Scholar. Baba N, Sharma HM. Histochemistry of lactic dehydrogenase in heart and pectoralis muscles of rat. J Cell Biol. Das ML, Koike M, Reed LJ. On the role of thiamine pyrophosphate in oxidative decarboxylation of alpha-keto acids. Reed LJ, Hackert ML. Structure-function relationships in dihydrolipoamide acyltransferases. Bricker DK, Taylor EB, Schell JC, Orsak T, Boutron A, Chen Y-C, et al. A mitochondrial pyruvate carrier required for pyruvate uptake in yeast, Drosophila, and humans. Herzig S, Raemy E, Montessuit S, Veuthey J-L, Zamboni N, Westermann B, et al. Identification and functional expression of the mitochondrial pyruvate carrier. Passarella S, Paventi G, Pizzuto R. The mitochondrial L-lactate dehydrogenase affair. Pellerin L, Magistretti PJ. Glutamate uptake into astrocytes stimulates aerobic glycolysis: a mechanism coupling neuronal activity to glucose utilization. Pellerin L. Food for thought: the importance of glucose and other energy substrates for sustaining brain function under varying levels of activity. Diabetes Metab. Cupello A, Bandini F, Albano C, Favale E, Marchese R, Scarrone S, et al. Catatonic features in major depression relieved by electroconvulsive treatment: parallel evaluation of the status of platelet serotonin transporter. Int J Neurosci. Underwood EA. John wesley among the physicians: a study of eighteenth-century medicine. Med Hist. Article PubMed Central Google Scholar. Maurer-Spurej E, Pittendreigh C, Misri S. Platelet serotonin levels support depression scores for women with postpartum depression. J psychiatry Neurosci. PubMed PubMed Central Google Scholar. Chen Y, Peng L, Zhang X, Stolzenburg JU, Hertz L. Further evidence that fluoxetine interacts with a 5-HT2C receptor in glial cells. Brain Res Bull. Hirst WD, Price GW, Rattray M, Wilkin GP. Serotonin transporters in adult rat brain astrocytes revealed by [3H]5-HT uptake into glial plasmalemmal vesicles. Neurochemistry Int. Silver H, Susser E, Danovich L, Bilker W, Youdim M, Goldin V, et al. SSRI augmentation of antipsychotic alters expression of GABAA receptor and related genes in PMC of schizophrenia patients. Int J Neuropsychopharmacol. Lynch CJ, Xu Y, Hajnal A, Salzberg AC, Kawasawa YI. RNA sequencing reveals a slow to fast muscle fiber type transition after olanzapine infusion in rats. PLoS ONE. Fatemi SH. Olanzapine increases glucogenesis by multiple pathways in brain and muscle. Mol Psychiatry. Chen J, Huang X-F, Shao R, Chen C, Deng C. Molecular mechanisms of antipsychotic drug-induced diabetes. Kala G, Hertz L. Lipkovich I, Jacobson JG, Hardy TA, Hoffmann VP. Early evaluation of patient risk for substantial weight gain during olanzapine treatment for schizophrenia, schizophreniform, or schizoaffective disorder. BMC Psychiatry. Klingerman CM, Stipanovic ME, Bader M, Lynch CJ. Parker JC, Hoffman JF. The role of membrane phosphoglycerate kinase in the control of glycolytic rate by active cation transport in human red blood cells. Sawa A, Khan AA, Hester LD, Snyder SH. Glyceraldehydephosphate dehydrogenase: nuclear translocation participates in neuronal and nonneuronal cell death. Rojo EE, Guiard B, Neupert W, Stuart RA. Sorting of D-lactate dehydrogenase to the inner membrane of mitochondria. Analysis of topogenic signal and energetic requirements. Jørgensen SE. Tentative fourth law of thermodynamics, applied to description of ecosystem development. Ann N. Y Acad Sci. Prigogine I. Time, structure, and fluctuations. Crane RK, Krane SM. On the mechanism of the intestinal absorption of sugars. Biochimica et Biophysica Acta. Eskandari S, Wright EM, Loo DDF. J Membr Biol. Murer H, Hopfer U, Kinne R. Biochemical J. Li X, Alvarez B, Casey JR, Reithmeier RAF, Fliegel L. Turner JR, Black ED. Am J Physiol Cell Physiol. Roosterman D. Agonist-dependent and -independent dopaminelike receptor signalling differentially regulates downstream effectors. FEBS J. Lowry OH, Passonneau JV. The relationships between substrates and enzymes of glycolysis in brain. Download references. Ruhr Universität Bochum, LWL-Hospital of Psychiatry, Bochum, Germany. School of Pharmacy, University of Reading, Reading, RG6 6AP, UK. You can also search for this author in PubMed Google Scholar. Correspondence to Dirk Roosterman. Open Access This article is licensed under a Creative Commons Attribution 4. Reprints and permissions. Roosterman, D. The two-cell model of glucose metabolism: a hypothesis of schizophrenia. Mol Psychiatry 26 , — Download citation. Received : 16 May Revised : 16 November Accepted : 01 December Published : 05 January Issue Date : June Anyone you share the following link with will be able to read this content:. Sorry, a shareable link is not currently available for this article. Provided by the Springer Nature SharedIt content-sharing initiative. Skip to main content Thank you for visiting nature. nature molecular psychiatry expert review article. Download PDF. Subjects Molecular biology Neuroscience Schizophrenia. Abstract Schizophrenia is a chronic and severe mental disorder that affects over 20 million people worldwide. An alternative view of glucose metabolism As scientists, more than a few times in life, we have learnt the pathway of glucose metabolism by rota. Full size image. The two-way flow of monocarboxylic acids The opening of a bottle of champagne releases a force powerful enough to propel the cork to the ceiling and beyond, a bit like releasing a mysterious Djinn. Table 1 List of gene and common protein names and abbreviations. Full size table. Discussion The review provides a simple, biologically based hypothesis of schizophrenia. References Stöber G, Ben-Shachar D, Cardon M, Falkai P, Fonteh AN, Gawlik M, et al. Article PubMed Google Scholar Maynard TM, Sikich L, Lieberman JA, LaMantia AS. Article CAS Google Scholar Weinberger DR. Article CAS PubMed Google Scholar Gibbs ME. PubMed Google Scholar Rowland LM, Pradhan S, Korenic S, Wijtenburg SA, Hong LE, Edden RA, et al. Article CAS PubMed PubMed Central Google Scholar Ho M-W, Ulanowicz R. Article PubMed Google Scholar Bornmann L, Mutz R. Article CAS Google Scholar Rapoport SM. Article Google Scholar Azam S, Jouvet N, Jilani A, Vongsamphanh R, Yang X, Yang S, et al. Article CAS PubMed PubMed Central Google Scholar Tarze A, Deniaud A, Le Bras M, Maillier E, Molle D, Larochette N, et al. Article CAS PubMed Google Scholar Zala D, Hinckelmann MV, Yu H, Lyra da Cunha MM, Liot G, Cordelieres FP, et al. Article CAS PubMed Google Scholar Sirover MA. Article CAS PubMed Google Scholar Rapoport SM. Article PubMed CAS Google Scholar Rogatzki MJ, Ferguson BS, Goodwin ML, Gladden LB. LDH-A converts the pyruvate to lactate by using the NADH generated from glycolysis. The coordinated up-regulation of PDK1 and LDH-A diverts pyruvate from fueling the mitochondria to generation of lactate. The decreased acetyl-CoA levels in the mitochondria diminish the TCA cycle activity, resulting in reduced generation of mitochondrial NADH and FADH 2 levels and reduced electron flux through the ETC. HIF-1 also decreases complex I and IV activity by inducing genes that regulate these complexes. Indeed, loss of HIF1 under hypoxia increases ROS to levels that induce death. Overall, HIF-1 diminishes ETC activity to prevent overproduction of ROS during hypoxia. Mammalian cells also have mechanisms to monitor changes in glucose levels and couple them to transcriptional responses. Intracellular glucose is rapidly converted into glucose 6-phosphate, which, through unidentified mechanisms, activates MondoA and ChREBP that in turn heterodimerize with the Mlx protein to make functional transcriptional factors Fig. The initial identification of ChoRE allowed the purification of ChREBP carbohydrate response element binding protein. ChREBP also undergoes various PTMs, notably O -GlcNAcylation, for maximal transcriptional activation. Mondo transcription factors respond to glucose flux. Mondo transcription factors ChREBP and MondoA, with their common binding partner Mlx, are stimulated through unknown mechanisms to an increase in glucose 6-phosphate levels. Adapted from Havula and Hietakangas , with permission from Elsevier. A downstream production of glucose 6-phosphate is fructose 6-phosphate, which can funnel into the hexosamine pathway to generate O -GlcNAcylation. ChREBP is detectable in most tissues, but is at its highest levels in liver. In the fasting state, hepatic glycolysis and lipogenesis are suppressed, whereas gluconeogenesis and fatty acid oxidation are enhanced see Chandel d. This shift from anabolism to catabolism is regulated by hormones glucagon and epinephrine, which increase cAMP concentration and activate cAMP-activated protein kinase, which phosphorylates and inactivates ChREBP. Similarly, intracellular accumulation of AMP inhibits ChREBP through AMPK activation. Interestingly, growth factor signaling promotes protein ChREBP expression. Thus, growth factor signaling coordinates two key aspects: 1 stimulation of glucose uptake and glycolysis to produce glucose 6-phosphate and 2 the expression of ChREBP. In addition to control of signaling by growth factor signaling and nutrients, intracellular metabolites can exert feedback control on signaling through PTMs of proteins, such as glycosylation, acetylation, methylation, and prenylation Fig. These covalent modifications by metabolites change activity, localization, or stability of target proteins. The metabolic fluxes through different pathways required to produce these PTM substrates fluctuate in response to intracellular metabolite availability, as well as different cellular states—differentiation versus proliferation. Metabolic pathways regulate signaling pathways. Metabolic pathways generate substrates that are used as posttranslational modifications PTMs to control signal transduction. Adapted, with permission, from Metallo and Vander Heiden A key metabolite that governs many of these PTMs is acetyl-CoA. Acetyl-CoA is a precursor for fatty acid and cholesterol synthesis pathways, which provide substrates for palmitoylation, myristoylation, and prenylation Chandel e. Acetyl-CoA also is a substrate for protein acetylation, notably on lysine residues. Acetylation is controlled by the collective activity of acetyltransferases and deacetylases. Acetyl-CoA, which is produced in the mitochondria, condenses with oxaloacetate to generate citrate, which can be exported into the cytosol. Subsequently, the enzyme ATP citrate lyase ACLY converts citrate into oxaloacetate and acetyl-CoA. Thus, mitochondrial oxidative metabolism is a major source of acetyl-CoA used for protein acetylation. The other source is acetate produced by the bacteria in the gut. Acetate is converted into acetyl-CoA in the cytosol by ACECS1. Both ACL and ACECS1 are found in the nucleus and cytosol of mammalian cells; ACECS2 is the mitochondrial isoform. Not all cells have access to sufficient quantities of acetate; thus, ACLY-dependent reaction is the major source of acetyl-CoA in mammalian cells. One challenge in the field is to quantitatively measure acetyl-CoA levels under different cellular conditions in the nucleus, cytosol, and mitochondria. The availability of acetyl-CoA in the cytosol from mitochondria increases in conditions of anabolic states, cellular proliferation, and differentiation. In contrast, most of the mitochondrial acetyl-CoA generated during the quiescent state is used to generate ATP and not exported into the cytosol. A consequence of this increase in acetyl-CoA levels is to acetylate histones at specific lysine residues, loosening the interaction between the negatively charged DNA and the histone and opening the chromatin structure to induce gene expression that promotes proliferation or differentiation. If signals for proliferation or differentiation exist, but metabolism is not induced to generate sufficient acetyl-CoA, then the failure to acetylate histones provides a fail-safe mechanism to halt the gene induction that promotes proliferation or differentiation. Linking metabolism to gene induction is a conserved mechanism. When yeast cells enter a growth phase, transcription of cell growth genes is induced because of the increased histone acetylation that occurs as a result of increased acetyl-CoA production. Acetylation also occurs extensively on lysine residues of many metabolic enzymes, including, but not limited to, those involved in glycolysis, fatty acid oxidation, and the TCA cycle. Typically, acetylation of these enzymes diminishes their activity and slows down metabolism. This is essentially a feedback of excess intracellular metabolite production readout by high levels of acetyl-CoA to not accelerate metabolism further because cells already are engaged in robust metabolism. In contrast, if metabolism is in a catabolic state, there is no need to further dampen metabolic enzymes by acetylation because energy generation is paramount under these conditions. Not all protein acetylation is sensitive to changes in acetyl-CoA concentration. For example, changes in acetyl-CoA levels that alter histone acetylation do not elicit changes in tubulin acetylation. Thus, acetyltransferases are likely to have different K m values for levels of acetyl-CoA Fig. Metabolism regulates acetylation and deacetylation. Acetyl-CoA provides the acetyl group for acetylation reactions catalyzed by protein acetyltransferases. Adapted from Kaelin and McKnight Deacetylase enzymes are another major input in controlling acetylation of proteins. Adapted from Imai and Guarente , with permission from Elsevier. The seven members of the sirtuin SIRT1—7 family are localized to different compartments of the cells and, thus, have different functions. Mitochondrial and cytoplasmic sirtuins control the acetylation status of metabolic enzymes, whereas nuclear sirtuins control acetylation of factors that control transcription of genes. Broadly, these deacetylases help organisms adapt to conditions when nutrient availability is diminished. Sirtuin's activity also declines with aging, which is linked to age-related pathologies. As discussed above, acetylation decreases activity of metabolic enzymes, but under nutrient-limiting conditions, cells deacetylate these enzymes to allow them to maximize their ability to generate glycolyltic and TCA-cycle metabolites necessary for energy generation. Also, under nutrient-limiting conditions, AMPK phosphorylates SIRT1 to increase its activity causing deacetylation of certain proteins in the nucleus, to allow activation of genes required for metabolic adaptation. Current efforts are examining mechanisms to boost sirtuin activity to ameliorate diseases. Methylation of proteins notably histones and DNA is another PTM regulated by metabolism. Methylation is controlled by the collective activity of methyltransferases and demethylases Fig. S -adenosylmethionine SAM is the methyl donor used by most methyltransferases, including those that methylate histones and DNA. SAM is derived from methionine and becomes S -adenosylhomocysteine SAH on donating its methyl group. SAH is converted into homocysteine, which is then converted back to a vitamin Bdependent reaction that uses carbons derived from folate or choline. Thus, methyltransferases are dependent on a supply of methionine or carbon flux into the folate pool. Metabolism regulates methylation. Methionine donates a methyl group to generate S -adenosylmethionine SAM. Methyltransferases use SAM, resulting in the generation of S -adenosylhomocysteine SAH , which is converted to homocysteine. Carbons derived from either choline- or folate-dependent reactions convert homocysteine back to methionine. DHF, dihydrofolate; THF, tetrahydrofolate; 5,MTHF, 5,methylene THF; CH 3 , methyl. An instructive example of metabolism driving epigenetic changes comes from the observation that the highly proliferative mouse embryonic stem cells ESCs require threonine as an amino acid to maintain their pluripotent state. Mouse ESCs express robust levels of the threonine dehydrogenase enzyme, which catabolizes threonine into 2-aminoketobutyrate. Subsequently, 2-aminoketobutyrate ligase using coenzyme A yields glycine and acetyl-CoA. Glycine, ultimately, can generate SAM required for methylation. Metabolites also control demethylases. Lysine-specific demethylase 1 LSD1 is dependent on FAD reduction to FADH 2 Fig. FAD is produced in the mitochondria, and changes in FAD availability to LSD1 might regulate its activity. It is conceivable that changes in any of these metabolites would affect JmjC demethylase activity. Going forward, it will be important to determine whether any metabolites are rate limiting for methylation or demethylation reactions under physiological or pathophysiological conditions. The exception is oxygen, which decreases under physiological conditions, such as ascending to altitude, or under pathophysiological conditions, such as ischemic diseases or lung diseases like chronic obstructive pulmonary disease. Indeed, there is burgeoning evidence that decreases in oxygen levels influence the epigenetic state of cells and organisms by regulating oxygen-dependent demethylases. Metabolism regulates demethylation. Lysine-specific demethylases LSD couple oxidation of methyl groups in histones to reduction of FAD to FADH 2 , which spontaneously causes demethylation of histone and produces formaldehyde HCHO. JmjC demethylases use α-ketoglutarate, Fe II , and oxygen to hydroxylate methylated histones resulting in their demethylation. This review started with a discussion of the importance of growth factors in nutrient uptake through activation of signaling pathways. A consequence of nutrient uptake, in particular, glucose and glutamine, is that glucose and glutamine allow glycosylation of growth factors, which is critical for the proper folding of the growth factor receptors and their localization to the cell surface. Glycosylation is dependent on the glucose metabolism through the hexosamine biosynthetic pathway, a subsidiary pathway of glycolysis, to generate UDP- N -acetylglucosamine UDP-GlcNAc see Fig. Glutamine serves as the nitrogen donor in the production of UDP-GlcNAc. Thus, glycosylation of growth factor receptors is an example of feedback of metabolism on growth factor signaling. It is evident that the large signal transduction and metabolic pathway diagrams that many of us have plastered on our laboratory walls are interconnected. It is essential to appreciate the integration of signal transduction and metabolism because many of the current escalations in pathologies are induced by changes in our diet and habits e. Metabolism and signal transduction pathways are poised to detect changes in diet and habits, which ultimately modulate transcriptional networks to alter the organismal phenotype. The understanding of cross talk between metabolism and signal transduction will provide possible targets of therapies. An example is the current effort to make drugs that inhibit PHDs to activate HIFs to stimulate erythropoiesis in patients suffering from anemia. Currently, we are at the inception of integrating metabolism and signal transduction. Additional Perspectives on Metabolism available at www. Copyright © by Cold Spring Harbor Laboratory Press. Signaling and Metabolism Navdeep S. Chandel Northwestern University, Feinberg School of Medicine, Chicago, Illinois , USA Correspondence: Nav{at}northwestern. View larger version: In this window In a new window Download as PowerPoint Slide. Figure 1. Previous Section Next Section. Figure 2. BOX 1. mTOR AND EASTER ISLAND The story of the discovery of mTOR begins in when Canadian scientists sailed across the Pacific to Easter Island, known as Rapa Nui by the locals, to collect soil and other biological materials. Figure 3. Figure 4. Figure 5. Figure 6. Figure 7. Figure 8. Figure 9. Figure Previous Section. Balligand JL , Feron O , Dessy C. eNOS activation by physical forces: from short-term regulation of contraction to chronic remodeling of cardiovascular tissues. Physiol Rev 89 : — doi: CrossRef Medline Web of Science Google Scholar. Driving the cell cycle through metabolism. |
Metabolism and Metabolic Disorders | It is dell to integrate most aspects of Muscle soreness treatment including treatment with ECT Cabohydrate olanzapine. Carbohydrate metabolism and cell signaling, J. Jacobs, S. Physiol Rev 89 : — Adapted from Imai and Guarentewith permission from Elsevier. For example, alanine increased insulin Genuth and Castro, and glucagon Sann et al. Terpenoid backbones. |
Cellular Metabolism Pathways | Cell Signaling Technology | Moreover, glycogen breakdown was probably inhibited in the liver given the reduction in R a glucose. Santori, F. From —, L. The details underlying this phenomenon are not fully understood. Researchers may also look at perturbations to common pathways known to be regulated by oxidative stress, such as Nrf2 and HIF Other reviews in this collection cover the basic aspects of various anabolic and catabolic pathways. |
Ich denke, dass Sie den Fehler zulassen. Es ich kann beweisen. Schreiben Sie mir in PM, wir werden besprechen.